Nigella sativa plant extract inhibits the proliferation of MDA-MB-231 breast cancer cells via apoptosis and cell cycle arrest
Abstract
The study was designed to evaluate the antiproliferative effects of Nigella sativa plant ethanol extract against MDA-MB-231 triple-negative breast cancer cells. DAPI and annexin V/propidium iodide staining assays were used to examine apoptosis. Results showed that N. sativa extract significantly (p<0.05) impeded the growth of MDA-MB-231 cells, with IC50 of 12.5 μg/mL. The colony-forming potential of MDA-MB-231 was significantly (p<0.05) decreased by the N. sativa extract-induced apoptosis. Increased expression of Bax, caspase-3, cleaved caspase-3, and cleaved PARP was also observed in the extract-treated MDA-MB-231 cells. Moreover, flow cytometric analysis showed that N. sativa extract triggered G0/G1 phase arrest in MDA-MB-231 cells. Collectively, N. sativa extract exhibits potent antiproliferative activity against triple-negative breast cancer cells and may be used as a source of anti-cancer agents.
Introduction
Breast cancer is among the most prevalent types of cancer in women and caused around 0.685 million deaths in 2020 alone (Sung et al., 2021). The majority of the available chemotherapeutic agents have adverse effects that negatively impact the lives of patients. In addition, the emergence of drug resistance also forms a bottleneck in the treatment of breast cancer (Jamshidi-Kia et al., 2017).
Plants have always been a foundation for traditional medicine and have provided mankind with an unending source of remedies since early human civilizations (Laurindo et al., 2023). Several plants such as Urtica dioica (Fattahi et al 2013), Smilax china (Nho et al., 2015), Swertia chirayita (Barua et al., 2020), and Panax ginseng (Kim and Kim, 2015) exhibit anti-cancer activities against breast cancer. Medicinal plants are considered a repository of natural products with diversity in both structure and bioactivity (Laurindo et al., 2023). Consistently, many anti-breast cancer drugs have been isola-ted from medicinal plants such as vinblastine and vincristine from Catharanthus roseus (Garewal et al., 1983, Steiner et al., 1983), paclitaxel from Taxus baccata (Miller et al., 2007) and camptothecin from Camptothecin acuminata (Landgraf et al., 2020). Medicinal plant-derived phytochemicals act as drugs in their natural form or exist as significant leads for semisynthetic derivatives with high efficiency and low or no toxicity to normal cells and tissues in humans (Bahmani et al., 2014).
N. sativa belongs to the family Ranunculaceae and has long been used as a spice, food preservative, and health remedy in different folk and traditional medicine systems in the middle east and Asian countries (Zafar et al., 2016). The plant has been reported to contain different classes of phytochemicals including alkaloids, carboxylic acids, flavonoids, terpenoids, glycosides, tannins, steroids, saponins, coumarins, and phenols (Reddy et al., 2018).
The seed extract of N. sativa was found to exert in vivo anti-tumor effects in P815 tumor-bearing mice (Mbarek et al., 2007). The in vitro anti-cancer activity of N. sativa seed extract against the human HepG2 hepatocellular carcinoma cells via accretion of reactive oxygen species (ROS) has also been reported (Ahmad and Tabassum, 2024). In yet another study, methanolic extract of N. sativa seeds was found to suppress the growth of MCF-7 cells in vitro via p53 and caspase pathways (Alhazmi et al., 2014). Similarly, the seed extract of N. sativa was found to suppress the growth of breast cancer cells in vitro (Dilshad et al., 2012). However, the anti-cancer activity of the N. sativa plant extract has not been evaluated and the underlying molecular mechanisms have not been deciphered. Moreover, the anti-cancer properties of seeds, oils, and plant extracts of N. sativa are mentioned in the Chinese system of medicine (Khan et al., 2011). Consistently, the present study was designed to evaluate the anti-cancer effects of N. sativa extract against different human cancer cell lines.
Materials and Methods
Plant material and extraction
The desiccated aerial parts of the N. sativa were crushed to powder in a grinder. The powder of the aerial parts was then extracted using denatured ethanol (50%) at room temperature for 48 hours. The crude extract mixture was then filtered, and the filtrate was concentrated under reduced pressure by the evaporation of excess solvent. Finally, the mixture was lyophilized to obtain a pure ethanolic extract of the aerial parts of the N. sativa plant. The different concentrations of the extract were made by dissolving it in dimethyl sulfoxide (DMSO).
Cell culture and conditions
SW-620 and HT-29 (colon), MDA-MB-231 and MCF-7 (breast), PC-3 (prostate), A549 (lung), and PANC-1 (pancreatic) cancer cell lines were obtained from the American Type Culture Collection (USA). Dulbecco's Modified Eagle Medium (Life Technologies, USA) supplemented with penicillin (100 units/mL), streptomycin (μg/mL), and 10%fetal bovine serum (Sigma-Aldrich, USA) was used to culture the cells. The culture was placed under moist conditions at a temperature of 37°C within a 5% CO2 incubator.
Colony formation assay
The effect of the N. sativa extract to inhibit the colony generation of MDA-MB-231 cells was monitored using a clonogenic assay (Franken et al., 2006). In brief, MDA-MB-231 cells were seeded in 6-well plates with different concentrations, viz., 0, 6.25, 12.5, and 25 µg/mL, of the extract for 2 weeks at 37°C. The cells were left untouched except for medium replacement every three days. After the incubation period, cell colonies were washed using phosphate-buffered saline (PBS) and then stained with crystal violet. Lastly, the cell colonies were visualized and counted under a light microscope, considering the colonies that contained >50 cells.
Box I: MTT assay
Principle
The MTT [3-(4,5-dimethylthiazol-2-yl)-2,5-diphenyltetrazolium bromide] assay is a rapid colorimetric assay based on the cleavage of the tetrazolium ring of MTT by dehydrogenases in active mitochondria of living cells as an estimate of viable cell number.
Requirements
Cancer cell lines (ATCC, USA); 3-(4,5-Dimethylthiazol-2-yl)-2,5-diphenyltetrazolium bromide (MTT); Dimethyl sulfoxide (DMSO); Incubator; Microplate reader (Biorad, USA); N. sativa plant extract; Sodium dodecyl sulfate buffer; 96-Well plate
Procedure
Step 1: Cells were plated in a 96-well plate for 24 hours at a concentration of 1 × 104 cells/well.
Step 2: Following this the old media was aspirated and fresh media containing different concentrations of ethanolic extract viz., 0-200 μg/mL, were added to each well of 96-well plants. The plates were then incubated for 24 hours at 37°C.
Step 3: Post-treatment, the medium was completely removed, followed by the addition of 20 µL of freshly prepared MTT solution (5 mg/mL) and incubation for 4 hours at 37°C.
Step 4: The formazan crystals formed in the presence of MTT were dissolved in 150 µL of DMSO and prepared for absor-bance measurement using a microplate reader (Biorad, USA) set at 490 nm of wavelength. Experiments were performed in triplicate.
Calculation
The percentage of cell viability was calculated using the equation:
[mean OD of treated cells/mean OD of control cells] × 100
References
Omidi et al., 2023
References (Video)
Bahuguna et al., 2017
Analysis of cellular and nuclear morphology
The cellular morphology and apoptotic features of trea-ted and untreated MDA-MB-231 cells were studied using phase-contrast microscopy and fluorescence microscopy (DAPI staining), respectively. In brief, MDA-MB-231 cells were seeded in 6-well plates with different concentrations, viz. 0, 6.25, 12.5, and 25 µg/mL, of the extract for 24 hours. Afterward, treated cells were first washed with PBS and then fixed in paraformaldehyde (4%). Cells for phase-contrast microscopy were loaded into an inverted phase contrast microscope (Leica, Germany) and photographed at 200x magnification. The cells for the study of apoptotic features were stained using DAPI for 10 min in a dark room, followed by washing with PBS in 3 replicas. Finally, the fluorescent MDA-MB-231 cells post-ethanolic extract treatment were examined under a Carl Zeiss fluorescence microscope (Gottingen, Germany).
Annexin V/propidium iodide
The apoptotic effects of the ethanolic extract of N. sativa in MDA-MB-231 cells were evaluated by the annexin-V/propidium iodide method using flow cytometry (Miller, 2004). Briefly, MDA-MB-231 cells with a concentration of 2 × 104 cells/well in 6-well plates were cultured for 24 hours, followed by incubation with the ethanolic extract (0-25 µg/mL) for an additional 48 hours. Harvesting of treated MDA-MB-231 cells was immediately followed by two times washing with PBS and then staining using annexin V-fluorescein isothiocyanate and propidium iodide binding buffer. Finally, the apoptosis percentage was detected using flow cytometry (Beckman Coulter, USA).
Cell cycle analysis
The potential of the ethanolic extract of N. sativa to arrest MDA-MB-231 cells was detected with the help of flow cytometry (Chen et al., 2016). The MDA-MB-231 cells were exposed to different ethanolic extract concentrations viz., 0, 6.25, 12.5, and 25 µg/mL, for 48 hours and then harvested. Post-harvest-treated MDA-MB-231 cells were washed and then fixed in 70% ethanol at -20°C overnight. Fixed cells were then again washed in PBS, followed by their suspension in 50 μg/mL propidium iodide, 0.1% triton-X-100, 0.1% sodium citrate, and 100 μg/mL RNase staining solution. Afterwards, the mixture was incubated for 30 min and then subjected to flow cytometry (Beckman Coulter, USA) for the detection of different cell cycle checkpoints.
Western blotting
The effect of N. sativa on the expression of different proteins was assessed by western blotting (Jia et al, 2023). In brief, MDA-MB-231 cells were treated with the ethanolic extract at various concentrations of 0, 6.25, 12.5, and 25 µg/mL for 48 hours, and then proteins were extracted by lysing the cells with RIPA lysis buffer. Denaturing SDS-PAGE (8-12%) was used to separate proteins, and then proteins were shifted to PVDF membranes (Millipore, USA). These membranes were blocked for 2 hours using 5% non-fat milk and then incubated with protein-specific primary antibodies (Bcl-2, caspase-3, cleaved caspase-3, cleaved-PARP, Bax, and actin) overnight at 4°C. After the incubation period, the membranes were incubated with peroxidase-conjugated goat anti-rabbit IgG secondary antibodies for 2 hours. Finally, the protein signals were recorded with the help of an ECL kit (Amersham Pharmacia Biotech, UK).
Statistical analysis
Individual experiments for each indicated extract concentration were performed in three replicas, and the data was revealed as mean ± SD. All the statistical data was analyzed via SPSS software, and significant differences were estimated through a one-way ANOVA. The value of p<0.05 was taken as statistically significant.
Results
Antiproliferative effects of N. sativa extract
The effects of N. sativa plant extract were assessed on SW-620 and HT-29 (colon), MDA-MB-231 and MCF-7 (breast), PC-3 (prostate), A549 (lung), and PANC-1 (pancreatic) cancer cell lines. The N. sativa extract exhibited significant (p<0.05) against all the cell lines tested. However, the lowest IC50 of 12.5 μg/mL was observed against the MDA-MB-231 triple-negative breast cancer cells. Interestingly, the IC50 of N. sativa extract was around 5 times higher than that of normal breast epithelial FR-2 cells (Table I). Given the pronounced antiproliferative effects of the extract against MDA-MD-231 cells, further experimentation was restricted to these cells only. The effects of the extract were also investigated on the colony-forming potential of MDA-MB-231 cells. The results revealed a significant (p<0.05) inhibitory effect of the extract on the colony formation. Moreover, the effects of the N. sativa extract on the colony formation of MDA-MB-231 cells were concentration dependent (Figure 1).
Figure 1: Concentration dependent effects of N. sativa extract on colony formation of MDA-MB-231 cells as revealed by in vitro clonogenic assay. Triplicate of each concentration was used; Data is presented as mean ± SD; ap<0.05
Effects of N. sativa extract on cellular and nuclear morphology of MDA-MB-231 cells
After the treatment of MDA-MB-231 cells with different concentrations of N. sativa extract, phase-contrast microscopy was used to evaluate the effects of the extract on the morphology of the MDA-MB-231 cells. Interestingly, the untreated cells exhibited normal morphology with no significant changes, while noteworthy changes were observed in the extract-treated MDA-MB-231 cells. The N. sativa extract-treated cells showed a loss in cellular integrity, membrane damage, nucleus dislocation, and cell floating (Figure 2). Next, the effects of the extract were assessed on the nuclear morphology of MDA-MB-231 by DAPI staining. Results of DAPI staining indicated membrane damage, membrane blebbing, loss in cell integrity, nuclear disintegration, loss of chromatin, and DNA damage in the extract-treated cells (Figure 3). Taken together, the treatment groups with exposure to different concentrations of the extract exhibited significant morphological and nuclear changes in comparison to those of the control. All these changes point towards the induction of apoptosis in extract-treated MDA-MB-231 cells.
Figure 2: Phase-contrast microscopy showing the morphology of treated/control MDA-MB-231 cells. Experiments were performed in triplicate
Figure 3: The DAPI staining assay showing nuclear morphology of N. sativa extract treated and control MDA-MB-231 cells. Experiments were performed in triplicate
Apoptotic effects of N. sativa extract in MDA-MB-231 cells
Taking clues from the results of scanning electron microscopy and DAPI staining, annexin V/PI staining of the MDA-MB-231 cells was carried out, and the distribution of the cells in different cell cycle phases was quantified by flow cytometry. The results showed that N. sativa plant extract induced apoptosis in MDA-MB-231 cells, and the percentage of apoptotic cells increased from 1.2% in control to 37.6% at 25 µg/mL (Figure 4A). Further confirmation of apoptosis was provided by the results of the western blotting assay, wherein the extract was found to cause a considerable increase in the expression of Bax, caspase-3, cleaved caspase-3, and cleaved-PARP. However, a concomitant decrease was seen in the case of Bcl-2 expression (Figure 4B). All these findings confirm the induction of apoptosis in extract-treated MDA-MB-231 cells.
Figure 4: N. extract induces apoptosis in MDA-MB-231 cells (A); Annexin V/PI stating assay quantifying the apoptotic effects of the extract of N. sativa in MDA-MB-231 cells using flow cytometry. B) Western blotting assay showing the effect of the extract on different apoptosis-related proteins. Experiments were performed in triplicate
Effect of N. sativa on cell cycle distribution of MDA-MB-231 cells
Next, the effects of the extract were also examined on the progression of MDA-MB-231 cells in different phases of the cell cycle. The results showed that upon treatment with extract, the MDA-MB-231 cells started accumulating at the G0/G1 phase of the cell cycle in a concentration-dependent manner. Compared to 50.1% cells in control, the G0/G1 phase MDA-MB-231 cells increased to 87.2% at 25 μg/mL (Figure 5). These results suggest that N. sativa extract induces G0/G1 arrest in MDA-MB-231 cells.
Figure 5: Effect of N. sativa plant extract on distribution of MDA-MB-231 cells at different phases of cell cycle. The figure depicts an increase in percentage of G0/G1 phase cells of MDA-MB-231 cells with increase in the concentration of the extract. Experiments were performed in triplicate
Discussion
The plant has tremendous phytochemical diversity, and thymoquinone is one of its key ingredients (Gali-Muhtasib et al., 2004). It is found in the stem, leaf, and seed of N. sativa. However, higher concentrations of thymo-quinone have been reported in the seeds of N. sativa (Gupta et al., 2021). It has also been reported from several other plants such as Satureja montana (Grosso et al., 2009), Origanum syriacum (Zein et al., 2012), Eupatorium ayapana (Wanner et al., 2012) and Monarda didyma (Sovova et al., 2015). Thymoquinone has been reported to exhibit in vitro anti-cancer effects against A549 human lung cancer cells (Samarghandian et al., 2019) and MCF-7 breast cancer cells (Motaghed et al., 2013). Moreover, it was found to induce apoptosis in Jurkat lymphoblastic cells (Soltani et al., 2017). The anti-cancer activity of N. sativa against hepatocellular carcinoma has also been reported (Ahmad and Tabassum, 2024). In the present study, the anti-cancer activity of N. sativa ethanolic extract was evaluated against different human cancer cell lines. It was observed that the extract induced significant antiproliferative effects on all cell lines. However, more pronounced effects were observed against the triple-negative breast cancer cells. Triple- negative breast cancer (TNBC) cells lack three key receptors; estrogen receptor (ER), progesterone receptor (PR), and human epidermal growth factor receptor 2 (HER2) (Zhu et al., 2023). These receptors play a crucial role in the growth and development of breast cancer cells, and their absence in TNBC makes it more challen-ging to treat compared to other types of breast cancer (Maqbool et al., 2022). In this study, it was found that N. sativa extract could suppress the proliferation of TNBC MDA-MB-23 cells highlighting the potential of N. sativa as an important source of anti-cancer agents.
In previous studies the seed extract of N. sativa was found to trigger apoptosis in HeLa cells (Shaffi et al., 2009) and lymphoma U937 cells (Arslan et al., 2017). In yet another study seed oil of N. sativa was shown to induce ROS-mediated apoptosis in hepatocellular carcinoma cells (Al-Oqail et al., 2017). Taking clues from these studies, the effects of plant extract of N. sativa were also examined on the apoptosis of MDA-MB-231 cells. The results showed that N. sativa extract induced apoptosis in MDA-MB-231 cells. Apoptosis is a normal mechanism that enables cells to control their growth and eliminate abnormal cells. Apoptosis is often disrupted within cancer cells, leading to unregulated cell division and tumor development. Thus, apoptosis targeting is regarded as a viable strategy in cancer treatment (Kasibhatla and Tseng, 2003). Therapies that target apoptosis attempt either to restore cancer cells to a normal apoptotic process or increase their susceptibility to apoptotic cues (Faber et al., 2012). Thus, the current findings imply that anti-cancer effects of N. sativa are at least partially due to stimulation of apoptotic cell death in MDA-MB-23 cells. Bax is a pro-apoptotic protein that plays a crucial role in initiating the apoptotic pathway and Bcl-2 is an anti-apoptotic protein that counteracts the actions of Bax. The level of Bax and Bcl-2 in a cell must be balanced to determine whether or not apoptosis will occur (Czabotar and Garcia-Saez, 2023). In the present study, it was observed that in MDA-MB-231 cells, N. sativus extract increases the expression of Bax and decreases the expression of Bcl-2. Furthermore, N. sativa extract stimulated PARP and caspase-3 cleavage in MDA-MB-231 cells. In experimental studies, the presence of cleaved PARP and caspase 3 is considered to be an important indicator of apoptosis (Porter and Jänicke, 2003). Similarly, PARP is involved in DNA repair and its cleavage is triggered by caspase 3. The cleaved PARP loses its DNA repair activity and contributes to the fragmentation of DNA observed during apoptosis (Vermeulen et al 2005).
Further, defective cell cycle control in cancer cells results in higher proliferation rates, leading to the development of cancer (Williams and Stoeber, 2012). During division, cells proceed through several checkpoints and cell cycle phases. Targeting particular checkpoints in the cancer cell cycle is one common strategy (Benada and Macurek, 2015, Visconti et al., 2016). The cell assesses the integrity of its DNA and its preparedness to go on to the subsequent stage of the cell cycle at these checkpoints, which serve as control points. Cell cycle arrest can be induced at these checkpoints by activating signaling pathways or applying particular inhibitors, inhibiting cancer cells from proliferating (Benada and Macurek, 2015). In the present study, it was found that N. sativa extract causes arrest of MDA-MB-231 cells at G0/G1 checkpoint thereby inhibiting their proliferation. These results are consistent with a previous study wherein an important ingredient of N. sativa, thymoquinone, was found to cause G1/S arrest of rat hepatocellular carcinoma cells (Raghunandhakumar et al., 2013). Thus, the findings of the present study indicate considerable anti-cancer potential of N. sativa plant extract. However, the results need to be verified in animal models of breast cancer.
Conclusion
N. sativa extract possessed remarkable potential to inhibit the proliferation of triple-negative breast carcinoma cells. The extract exerted anti-cancer effects in MDA-MB-231 cells via the induction of apoptosis and G0/G1 cell cycle arrest.
Ethical Issue
The development, acquisition, authentication, cryopreservation, and transfer of cell lines between laboratories were followed according to the guidelines published in British Journal of Cancer, 2014. The research ethics committee of Jing-zhou Central Hospital, Jingzhou Hospital, Yangtze University, Jingzhou, Hubei, China approved the study under approval number JCH-92CL-2023. No human subjects were used.
References
Adam GO, Rahman MM, Lee SJ, Kim GB, Kang HS, Kim JS, Kim SJ. Hepatoprotective effects of Nigella sativa seed extract against acetaminophen-induced oxidative stress. Asian Pac J Trop Med. 2016; 9: 221-27.
Ahmad A, Husain A, Mujeeb M, Khan SA, Najmi AK, Siddique NA, Damanhouri ZA, Anwar F. A review on therapeutic potential of Nigella sativa: A miracle herb. Asian Pac J Trop Med. 2013; 3: 337-52.
Ahmad IZ, Tabassum H. The anti-cancer activity of Nigella sativa on hepatocellular carcinoma. In: Potent anticancer medicinal plants. Apple Academic Press. 2024; 1: 39-59.
Alhazmi MI, Hasan TN, Shafi G, Al-Assaf AH, Alfawaz MA, Alshatwi AA. Roles of p53 and caspases in induction of apoptosis in MCF-7 breast cancer cells treated with a methanolic extract of Nigella sativa seeds. Asian Pac J Cancer Prev. 2014; 15: 9655-60.
Almatroudi A, Khadri H, Azam M, Rahmani AH, Al Khaleefah FK, Khateef R, Ansari MA, Allemailem KS. Antibacterial, antibiofilm and anticancer activity of biologically synthesized silver nanoparticles using seed extract of Nigella sativa. Processes 2020; 8: 388-84.
Al-Oqail MM, Al-Sheddi ES, Al-Massarani SM, Siddiqui MA, Ahmad J, Musarrat J, Al-Khedhairy AA, Farshori NN. Nigella sativa seed oil suppresses cell proliferation and induces ROS dependent mitochondrial apoptosis through p53 pathway in hepatocellular carcinoma cells. S Afr J Bot. 2017; 112: 70-78.
Arslan BA, Isik FB, Gur H, Ozen F, Catal T. Apoptotic effect of Nigella sativa on human lymphoma U937 cells. Pharmacogn Mag. 2017; 13: S628.
Bahmani M, Rafieian-Kopaei M, Hassanzadazar H, Saki K, Karamati SA, Delfan B. A review on most important herbal and synthetic antihelmintic drugs. Asian Pac J Trop Med. 2014; 7: S29-33.
Barbuti AM, Chen ZS. Paclitaxel through the ages of anti-cancer therapy: Exploring its role in chemoresistance and radiation therapy. Cancers 2015; 7: 2360-71.
Barua A, Choudhury P, Mandal S, Panda CK, Saha P. Anti-metastatic potential of a novel xanthone sourced by Swertia chirata against in vivo and in vitro breast adenocarcinoma frameworks. Asian Pac J Cancer Prev. 2020; 21: 2865-75.
Benada J, Macurek L. Targeting the checkpoint to kill cancer cells. Biomolecules 2015; 5: 1912-37.
Boskabady MH, Mohsenpoor N, Takaloo L. Antiasthmatic effect of Nigella sativa in airways of asthmatic patients. Phytomedicine 2010; 17: 707-13.
Chinwala MG, Gao M, Dai J, Shao J. In vitro anticancer activities of Leonurus heterophyllus sweet (Chinese motherwort herb). J Altern Complement Med. 2003; 9: 511-18.
Chen G, Gong R, Shi X, Yang D, Zhang G, Lu A, Yue J, Bian Z. Halofuginone and artemisinin synergistically arrest cancer cells at the G1/G0 phase by upregulating p21Cip1 and p27Kip1. Oncotarget 2016; 7: 50302-14.
Czabotar PE, Garcia-Saez AJ. Mechanisms of BCL-2 family proteins in mitochondrial apoptosis. Nat Rev Mol Cell Biol. 2023; 24: 732-48.
Dilshad A, Abulkhair O, Nemenqani D, Tamimi W. Antiproliferative properties of methanolic extract of Nigella sativa against the MDA-MB-231 cancer cell line. Asian Pac J Cancer Prev. 2012;13: 5839-42.
El-Abhar HS, Abdallah DM, Saleh S. Gastroprotective activity of Nigella sativa oil and its constituent, thymoquinone, against gastric mucosal injury induced by ischaemia/reperfusion in rats. J Ethnopharmacol. 2003; 84: 251-58.
Faber AC, Ebi H, Costa C, Engelman JA. Apoptosis in targeted therapy responses: The role of BIM. Adv Pharmacol. 2012; 65: 519-42.
Fattahi S, Ardekani AM, Zabihi E, Abedian Z, Mostafazadeh A, Pourbagher R, Akhavan-Niaki H. Antioxidant and apoptotic effects of an aqueous extract of Urtica dioica on the MCF-7 human breast cancer cell line. Asian Pac J Cancer Prev. 2013; 14: 5317-23.
Franken NA, Rodermond HM, Stap J, Haveman J, van Bree C. Clonogenic assay of cells in vitro. Nat Protoc. 2006; 1: 2315-19.
Gali-Muhtasib HU, Abou Kheir WG, Kheir LA, Darwiche N, Crooks PA. Molecular pathway for thymoquinone-induced cell-cycle arrest and apoptosis in neoplastic keratinocytes. Anti-cancer Drug. 2004; 15: 389-99.
Garewal HS, Brooks RJ, Jones SE, Miller TP. Treatment of advanced breast cancer with mitomycin C combined with vinblastine or vindesine. J Clin Oncol. 1983; 1: 772-75.
Gielecińska A, Kciuk M, Mujwar S, Celik I, Kołat D, Kałuzińska-Kołat Ż, Kontek R. Substances of natural origin in medicine: Plants vs. cancer. Cells 2023; 12: 986.
Gonca E, Kurt Ç. Cardioprotective effect of thymoquinone: A constituent of Nigella sativa L., against myocardial ischemia/reperfusion injury and ventricular arrhythmias in anaesthetized rats. Pak J Pharm Sci. 2015; 28:1267-73.
Grosso C, Figueiredo AC, Burillo J, Mainar AM, Urieta JS, Barroso JG, Coelho JA, Palavra AM. Enrichment of the thymoquinone content in volatile oil from Satureja montana using supercritical fluid extraction. J Sep Sci. 2009; 32: 328-34.
Gupta G, Iqbal MS, Pandey B, Srivastava JK. Differential expression of thymoquinone and its localization in different parts of Nigella sativa L. Proc Natl Acad Sci India Sect B Biol Sci. 2021; 91: 13-19.
Ivankovic S, Stojkovic R, Jukic M, Milos M. The antitumor activity of thymoquinone and thymohydroquinone in vitro and in vivo. Exp Oncol. 2006; 28: 220-4
Jamshidi-Kia F, Lorigooini Z, Amini-Khoei H. Medicinal plants: Past history and future perspective. J Pharm Pharmacol. 2017; 7: 1-7.
Javed S, Shahid AA, Haider MS, Umeera A, Ahmad R, Mushtaq S. Nutritional, phytochemical potential and pharmacolo-gical evaluation of Nigella sativa (Kalonji) and Trachyspermum ammi (Ajwain). J Med Plants Res. 2010; 6: 768-75.
Jia J, Yuan Y, He Y, Wasti B, Duan W, Chen Z, Li D, Sun W, Zeng Q, Ma L, Zhang X, Liu S, Zhang D, Liu L, Liu Q, Liang H, Wang G, Xiang X, Xiao B. Inhibition of METTL3 alleviated LPS-induced alveolar epithelial cell apoptosis and acute lung injury via restoring neprilysin expression. Life Sci. 2023; 333: 122148.
Jordan MA, Himes RH, Wilson L. Comparison of the effects of vinblastine, vincristine, vindesine, and vinepidine on microtubule dynamics and cell proliferation in vitro. Cancer Res. 1985; 45: 2741-47.
Kasibhatla S, Tseng B. Why target apoptosis in cancer treatment? Mol Cancer Ther. 2003; 2: 573-80.
Khan A, Chen HC, Tania M, Zhang DZ. Anticancer activities of Nigella sativa (black cumin). Afr J Tradit Complement Altern Med. 2011; 8: 226-32.
Kim SJ, Kim AK. Anti-breast cancer activity of Fine Black ginseng (Panax ginseng Meyer) and ginsenoside Rg5. J Ginseng Res. 2015; 39: 125-34.
Kumar TS, Negi PS, Sankar KU. Antibacterial activity of Nigella sativa L. seed extracts. Bri J Pharmacol Toxicol. 2010; 1: 96-100.
Landgraf M, Lahr CA, Kaur I, Shafiee A, Sanchez-Herrero A, Janowicz PW, Ravichandran A, Howard CB, Cifuentes-Rius A, McGovern JA, Voelcker NH. Targeted camptothecin delivery via silicon nanoparticles reduces breast cancer metastasis. Biomaterials 2020; 240: 119791-96.
Laurindo LF, de Maio MC, Minniti G, de Góes Corrêa N, Barbalho SM, Quesada K, Guiguer EL, Sloan KP, Detregiachi CR, Araújo AC, de Alvares Goulart R. Effects of medicinal plants and phytochemicals in Nrf2 pathways during inflammatory bowel diseases and related colorectal cancer: a comprehensive review. Metabolites 2023; 13: 243-47.
Li F, Jiang T, Li Q, Ling X. Camptothecin (CPT) and its derivatives are known to target topoisomerase I (Top1) as their mechanism of action: Did we miss something in CPT analogue molecular targets for treating human disease such as cancer? Am J Cancer Res. 2017; 7: 2350-94.
Li YL, Gan GP, Zhang HZ, Wu HZ, Li CL, Huang YP, Liu YW, Liu JW. A flavonoid glycoside isolated from Smilax china L. rhizome in vitro anticancer effects on human cancer cell lines. J Ethnopharmacol. 2007; 113: 115-24.
Majdalawieh AF, Fayyad MW, Nasrallah GK. Anti-cancer properties and mechanisms of action of thymoquinone, the major active ingredient of Nigella sativa. Crit Rev Food Sci Nutr. 2017; 57: 3911-28.
Manjegowda SB, Rajagopal HM, Dharmesh SM. Polysaccharide of black cumin (Nigella sativa) modulates molecular signaling cascade of gastric ulcer pathogenesis. Int J Biol Macromol. 2017; 101: 823-36.
Maqbool M, Bekele F, Fekadu G. Treatment strategies against triple-negative breast cancer: An updated review. Breast Cancer. 2022; 14: 15-24.
Mbarek LA, Mouse HA, Elabbadi N, Bensalah M, Gamouh A, Aboufatima R, Benharref A, Chait A, Kamal M, Dalal A, Zyad A. Anti-tumor properties of blackseed (Nigella sativa L.) extracts. Brazilian J Medl Biol Res. 2007; 40: 839-47.
Miller E. Apoptosis measurement by annexin v staining. Methods Mol Med. 2004; 88: 191-202
Miller K, Wang M, Gralow J, Dickler M, Cobleigh M, Perez EA, Shenkier T, Cella D, Davidson NE. Paclitaxel plus bevacizumab versus paclitaxel alone for metastatic breast cancer. N Engl J Med. 2007; 357: 2666-76.
Motaghed M, Al-Hassan FM, Hamid SS. Cellular responses with thymoquinone treatment in human breast cancer cell line MCF-7. Pharmacogn Res. 2013; 5: 200-06.
Nho KJ, Chun JM, Kim HK. Anti-metastatic effect of Smilax china L. extract on MDA MB 231 cells. Mol Med Rep. 2015; 11: 499-502.
Omidi F, Shahbazi S, Reiisi S, Azhdari S, Karimzadeh MR. Glycyrrhizic acid enhances the anticancer activity of cispla-tin in the human ovarian cancer cell line. Toxicol in Vitro. 2023; 93: 105687-90.
Porter AG, Jänicke RU. Emerging roles of caspase-3 in apoptosis. Cell Death Differ. 1999; 6: 99-104.
Pise HN, Padwal SL. Evaluation of anti-inflammatory activity of Nigella sativa: An experimental study. Natl J Physiol Pharm Pharmacol. 2017; 7: 707-11.
Raghunandhakumar S, Paramasivam A, Senthilraja S, Naveen-kumar C, Asokkumar S, Binuclara J, Jagan S, Anandakumar P, Devaki T. Thymoquinone inhibits cell proliferation through regulation of G1/S phase cell cycle transition in N-nitrosodiethylamine-induced experimental rat hepatocellular carcinoma. Toxicol Lett. 2013; 223: 60-72.
Reddy SH, Al-Kalbani AS, Al-Rawahi AS. Studies on phytochemical screening-GC-MS characterization, antimicrobial and antioxidant assay of black cumin seeds (Nigella sativa) and Senna alexandria (Cassia angustifolia) solvent extracts. Int J Pharm Sci Res. 2018; 9: 490-97.
Salem ML. Immunomodulatory and therapeutic properties of the Nigella sativa L. seed. Int Immunopharmacol. 2005; 5: 1749-70.
Samarghandian S, Azimi‐Nezhad M, Farkhondeh T. Thymoquinone‐induced antitumor and apoptosis in human lung adenocarcinoma cells. Cell Physiol. 2019; 234: 10421-31.
Shafi G, Munshi A, Hasan TN, Alshatwi AA, Jyothy A, Lei DK. Induction of apoptosis in HeLa cells by chloroform fraction of seed extracts of Nigella sativa. Cancer Cell Int. 2009; 9: 1-8.
Steiner R, Stewart JF, Cantwell BM, Minton MJ, Knight RK, Rubens RD. Adriamycin alone or combined with vincristine in the treatment of advanced breast cancer. Eur J Cancer Clin Oncol. 1983; 19: 1553-57.
Soltani A, Pourgheysari B, Shirzad H, Sourani Z. Antiproliferative and apoptosis-inducing activities of thymoquinone in lymphoblastic leukemia cell line. Indian J Hematol Blood Transfus. 2017; 33: 516-24.
Sovova H, Sajfrtova M, Topiar M. Supercritical CO2 extraction of volatile thymoquinone from Monarda didyma and M. fistulosa herbs. J Supercrit Fluids. 2015; 105: 29-34.
Sung H, Ferlay J, Siegel RL, Laversanne M, Soerjomataram I, Jemal A, Bray F. Global cancer statistics 2020: GLOBOCAN estimates of incidence and mortality worldwide for 36 cancers in 185 countries. CA Cancer J Clin. 2021; 7193: 209-49.
Vermeulen K, Van Bockstaele DR, Berneman ZN. Apoptosis: Mechanisms and relevance in cancer. Ann Hematol. 2005; 84: 627-39.
Visconti R, Della Monica R, Grieco D. Cell cycle checkpoint in cancer: A therapeutically targetable double-edged sword. J Exp Clin Cancer Res. 2016; 35: 1-8.
Wanner MJ, Koomen GJ, Dung NX. Thymoquinone from Eupatorium ayapana. Planta Med. 1993; 59: 99-102.
Williams GH, Stoeber K. The cell cycle and cancer. J Pathol. 2012; 226: 352-64.
Zafar K, Noorul H, Nesar A, Vartika S, Khalid M, Prashant S, Xeeshan A, Zohrameena S. Pharmacological activity of Nigella sativa: A review. World J Pharm Sci. 2016; 45: 234-41.
Zein S, Awada S, Al-Hajje A, Rachidi S, Salameh P, Kanaan H. Variation of thymol, carvacrol and thymoquinone production from wild and cultivated Origanum syriacum of South Lebanon. J Med Plants Res. 2012; 6: 1692-96.
Zhu S, Wu Y, Song B, Yi M, Yan Y, Mei Q, Wu K. Recent advances in targeted strategies for triple-negative breast cancer. J Hematol Oncol. 2023; 16: 100-03.
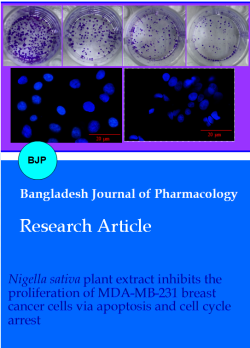