RU.521 protects against neutrophil extracellular traps-induced vascular endothelial injury by inhibiting cGAS/STING pathway
Abstract
This study aims to investigate the effect of RU.521 on improving vascular endothelial injury in 14 acute lung injury patients. Serum myeloperoxidase, double-stranded DNA (dsDNA), IFN-β, and TNF-α levels of patients were detected by ELISA. Neutrophil extracellular traps were used to stimulate human venous endothelial cells (HUVEC). cGAS/STING pathway protein was detected by western blot. The protective effect of RU.521 on HUVEC was evaluated by CCK-8. The effects of RU.521 on cGAS/STING pathway expression were detected by PCR and western blot. The levels of myeloperoxidase and (dsDNA) in acute lung injury patients were significantly increased (p<0.05). The expression level of IFN-β in acute lung injury patients was significantly increased (p<0.05). The expression of cGAS and STING proteins in HUVEC cells was significantly increased (p<0.05), and the expression of IFN-β was significantly increased (p<0.05). RU.521 can ameliorate vascular endothelial injury. RU.521 inhibited cGAS/SRING mRNA and protein expression (p<0.05). Thus, neutrophil extracellular traps release dsDNA activates the cGAS/STING pathway-induced vascular endothelial injury in acute lung injury patients. RU.521 protects vascular endothelial injury by inhibiting the cGAS/STING pathway.
Introduction
Acute lung injury is a common clinical syndrome. The main pathological features are diffuse pulmonary interstitial and alveolar edema caused by pulmonary capillary endothelial cells and alveolar epithelial cells injury, which leads to acute hypoxemia, and acute respiratory distress syndrome in severe cases, which is life-threatening (Qiao et al., 2024). At present, the treatment of acute lung injury mainly focuses on supportive therapy, drug therapy, and mechanical ventilation, and there is still a lack of effective treatment (Munshi et al., 2022). Therefore, finding new drugs to treat acute lung injury is of great significance for improving the survival rate and quality of life of patients.
Vascular endothelial injury plays an important role in acute lung injury (Liu et al., 2015), and neutrophils participate in vascular endothelial cell injury by releasing neutrophil extracellular traps (NETs)(Fei et al., 2024). When acute lung injury occurs, neutrophils are activated to form NETs, releasing substances such as elastase, myeloperoxidase, citrullinated histone H3, and double-stranded DNA (dsDNA). The released dsDNA and other substances will cause secondary damage to their vascular endothelial cells and aggravate the develop-ment of acute lung injury (Fei et al., 2024).
Recent studies have shown that dsDNA can participate in vascular endothelial injury through the activation of STING pathway during acute lung injury (Zhu et al., 2023). When cyclic GMP-AMP synthase (cGAS) was used to detect abnormal dsDNA in cytoplasm, cataly-zed the synthesis of the second messenger 2 '3'-cGAMP (cGAMP), which effectively activates STING in the endoplasmic reticulum. Subsequently, STING underwent a conformational change from endoplasmic reticu-lum translocation to perinuclear septum, and recruited TANK-binding kinase 1 to produce highly ordered con-tinuous phosphorylation. TANK-binding kinase 1 phosphorylates interferon (IFN) regulatory factor 3, activates interferon regulatory factor 3 dimer to enter the nucleus, induces type I interferons production, and leads to endothelial cell damage (Decout et al., 2021). Therefore, cGAS/STING pathway may be a new target for the treatment of vascular endothelial injury in acute lung injury.
RU.521 is a selective cGAS inhibitor that inhibits cGAS-mediated interferon up-regulation and suppresses dsDNA-activated reporter activity (Uthaman et al., 2024). RU.521 can improve sepsis-related organ func-tional impairment, but its specific mechanism has not been fully clarified (Li et al., 2022; Luo et al., 2024). This study intends to confirm that RU.521 can reduce endothelial cell damage by inhibiting the activation of STING pathway, dsDNA released by NETs through cell experiments.
Materials and Methods
Patients
Patients meeting acute lung injury clinical criteria were recruited from Suzhou Hospital of Integrated Traditional Chinese and Western Medicine from August to December 2023. Overall, 14 patients with acute lung injury were included, patients age ranges between 30 and 88 years old (males 10, females 4). Acute lung injury was defined according to the Berlin definition (Ranieri et al., 2012). Same number of patients without acute lung injury were matched based on age and gender during the same time.
Cell culture
HUVEC (Human venous endothelial cells) were purchased from iCell (China). HUVEC were maintained in RPMI 1640 (Gibco, C11875500BT) media containing 10% fetal bovine serum (Bdbio, F801-050Hi). Human primary neutrophils isolation was performed using a neutrophil isolation kit (Solarbio, P9040). Human primary neutrophils were maintained in RPMI 1640 media containing 10% fetal bovine serum. All cells were grown at 37°C in the presence of 5% CO2.
Box I: Western Blot
In western blotting, proteins are separated into gels based on their molecular weight as a result of immunochromatography.
Requirements
ECL (Medicalbio, PT01001) reagent; Fat-free milk; HRP-conjugated secondary antibody (ABclonal, AS014); HUVEC cells; Image J software; Phosphatase inhibitors (Beyotime Biotechnology); Protease inhibitors (Beyotime Biotechnology); PVDF (0.45 µm) membrane; RIPA bufer with 1 mM PMSF; SDS-PAGE; STING (Abcam, ab181125), p-STING (Biodragon, BD-PP1684), cGAS (Proteintech, 26416-1-AP), anti-GAPDH (Biodragon, B1034); Tanon scan imager; TBST buffer
Procedure
Step 1: HUVEC cells were lysated in RIPA bufer with 1 mM PMSF, protease inhibitors and phosphatase inhibitors for 30 min.
Step 2: Protein sample was then determined by the BCA method (Beyotime Biotechnology).
Step 3: After 10% SDS-PAGE electrophoresis separation, the gel was transferred to PVDF membrane.
Step 4: PVDF membranes were blocked in 5% fat-free milk for 60 min at room temperature.
Step 5: Then PVDF membranes were rinsed with TBST buffer 3 times for 5 min and probed with STING (1:1000), p-STING (1:1000), cGAS (1:3000), anti-GAPDH (1:5000), at 4℃ overnight.
Step 6: After rinsing with TBST buffer 3 times for 5 min, PVDF membranes were then incubated in HRP-conjugated secondary antibody (1:7500) at room temperature for 1 hour.
Step 7: After rinsing with TBST buffer 3 times for 5 min. Finally, the membrane was imaged at Tanon scan imager after ECL reagent visualization, all bands was analyseed by Image J software.
References
Raybuck and Lee et al., 2019
References (Video)
Han et al., 2023; Jiang et al., 2022
NETs
Human primary neutrophils were isolated from the peripheral blood using a commercially available kit (Solarbio, P9040). Human primary neutrophil pellets were resuspended in DMEM supplemented with 10% fetal bovine serum, 1% glutamine, and 1% penicillin/streptomycin solution at a concentration of 1 x 106 cells/mL. Human primary neutrophils were stimulated by lipopolysaccharide to activate neutrophils to form neutrophil extracellular traps. Freshly isolated human or murine neutrophils were plated in 6-well culture plates (4 x 106 cells/mL) and stimulated with lipopolysaccharide (10 g/mL) for 3 hours. To remove the cells, the culture medium was carefully removed, and each well was washed twice with 1 mL of cold phosphate-buffered saline. It was then resuspended in fresh PBS that contained the pellet that contained neutrophil extrace-llular traps (Xie et al., 2023). Isolated NETs were then stored at -20ºC until they were used.
ELISA
Human IFN-β ELISA kit (EK1236), human TNF-α ELISA kit (EK182), and human myeloperoxidase/myeloperoxidase ELISA kit (EK1133) were purchased from Multisciences (China), and were used according to the manufacturer’s instructions. In brief, diluted samples and the standards were added to the antibody-coated orifice, then, added detection antibodies for 2 hours at room temperature. Discard the liquid, add 300 µL lotion to each hole to wash the plate, and washed 6 times. After washing the board, it was to pat it dry on absorbent paper. Add 100 µL streptavidin working solution per well for 45 min at room temperature. The absorbance was detected by TMB color rendering and enzyme-labeled instrument (Li et al., 2023).
Double-stranded DNA (dsDNA) determination
Determination of dsDNA was quantified using the dsDNA quantitation assay kit (Medicalbio, MR0013) according to the manufacturer’s instructions. Allow the kit to equilibrate at room temperature prior to use. 10 uL samples were added to 190 µL detection buffer and mixed, incubated at room temperature away from light for 3 min. Following the addition of the dye reagent to each well and subsequent incubation for a duration of 15 min at room temperature, the measurement of emi-ssion wavelength at 520 nm, in conjunction with an excitation wavelength of 480 nm, was conducted (Ray-buck et al., 2019). Synergy LX Multi-Mode Reader (USA) was used for detection.
RT-qPCR
Total RNA was extracted from cell samples using MolPure cell/tissue total RNA kit (Yeasen, 19221ES50) and then reverse-transcribed into cDNA with HS RT SuperMix (Medicalbio, MR0110) according to the instructions. SYBR qPCR master mix (Medicalbio, MR0321) was designed according to the instructions. The 20 µL reaction mixture contained 0.4 µL each of upstream and downstream primers, 10 µL of SYBR Green master mix, 1 µL template cDNA, and 8.2 uL nuclease-free water. The 2−ΔΔCT method was used for the data analysis. GAPDH was used as an internal control (Raybuck et al., 2019). The primers were synthesized by Sangon Biotech Corporation. The sequences of primers used are the following:
cGAS-F:CACGAAGCCAAGACCTCCG,cGAS-R:GTCGCACTTCAGTCTGAGCA, STING-F:CCAGAGCACACTCTCCGGTA,STING-R:CGCATTTGGGAGGGAGTAGTA, GAPDH-F:TGTGGGCATCAATGGATTTGG, GAPDH-R:ACACCATGTATTCCGGGTCAAT.
Statistical analysis
Data were expressed as mean ± SEM. The analysis was performed using Graphpad Prism 5 (Graphpad Software, USA). To determine whether the two groups differed statistically, a t-test was used. Significant results were determined by a p-value less than 0.05.
Results
Myeloperoxidase and dsDNA levels
To verify the expression of NETs in acute lung injury patients, the serum myeloperoxidase and dsDNA levels were assessed. Patients who did not develop acute lung injury as the control group. Compared with the control group, the levels of myeloperoxidase and dsDNA in acute lung injury patients were significantly increased, the difference was statistically significant (p<0.05; Figure 1). These results suggest that acute lung injury patients had NETs and high levels of dsDNA.
Figure 1: The levels of myeloperoxidase (A) and double-stranded DNA (dsDNA) (B) in control and acute lung injury groups. Data are expressed as mean ± SEM. Compared to the control group, ap<0.05
dsDNA-mediated vascular endothelial injury through activation of the STING pathway
It has been reported that the release of excessive dsDNA from NETs can activate STING pathway to damage vascular endothelial cells and aggravate lung injury. To verify this conclusion, the expression level of IFN-β in the blood of acute lung injury patients was detected, and found that the expression levels of IFN-β and TNF-α in acute lung injury patients was significantly increased (p<0.05l Figure 2 A-B), suggesting that STING pathway may be activated in acute lung injury patients. Further experiments were conducted using NETs to stimulate HUVEC cells in vitro. The results showed that after NETs stimulation, the expression of cGAS and STING proteins in HUVEC cells was significantly increased (p<0.05; Figure 2 D-E), and the expression of IFN-β was significantly increased (p<0.05; Figure 2C). Thus, NETs release dsDNA would activate the STING pathway.
Figure 2: The levels of IFN-β (A) and TNF-α (B) between control group and acute lung injury group. The expression of IFN-β (C) in HUVEC cells stimulated with NETs was quantified between two groups (C). The expression levels of cGAS and p-STING/STING were analyzed between two groups (D-F)
Vascular endothelial through inhibiting STING pathway
To verify the effect of RU.521 on vascular endothelial injury, CCK-8 detected HUVEC in different groups. HUVEC survival decreased after the addition of NETs, while HUVEC injury was reversed after the use of 700 nM RU.521 (p<0.05; Figure 3A). To investigate whether RU.521 can ameliorates vascular endothelial injury by STING pathway, western blot, and PCR experiments were conducted, and the PCR results indicated that cGAS and STING mRNA expressions were significantly decreased in RU.521 group compared with NETs group (p<0.05; Figure 3 B-C). The results of the western blot indicated that RU.521 could significantly inhibit the expression of cGAS and p-STING/STING (p<0.05; Figure D-E). Therefore, RU.521 ameliorates vascular endothelial injury by inhibiting the cGAS/STING pathway.
Figure 3: Effects of RU.521 on cell viability (A), cGAS/GAPDH mRNA (B), STING/GAPDH mRNA vascular endothelial injury. The levels of cGAS and p-STING/STING were analyzed by western blotting within the three groups (D-F). Data are expressed as mean ± SEM. Compared to the control group, ap<0.05.Compared to the NETs group, bp<0.05
Discussion
In this study, the findings were as follows: acute lung injury patients have NETs and a large amount of dsDNA exists in serum, and the appearance of dsDNA may be related to the formation of NETs. The expression levels of IFN-β and TNF-α in acute lung injury patients were significantly increased, suggesting that cGAS/STING might be activated. In vitro experiments confirmed that dsDNA release from NETs could activate the cGAs/STING pathway of vascular endothelial cells, resulting in vascular endothelial damage. RU.521 can improve the survival rate of HUVEC, and in vitro experiments showed that RU.521 can improve vascular endothelial injury by inhibiting cGAS/STING pathway.
Acute lung injury is a common disease in ICU, about general sepsis patients will be complicated with acute lung injury, and the mortality of patients with acute lung injury is significantly increased (Xie et al., 2020). Vascular endothelial injury is an important pathogenesis of acute lung injury. Studies have found that NETs can directly damage vascular endothelial cells and aggravate the development of acute lung injury (Fei and Huang et al., 2024). cGAS/STING pathway activation may be one of the pathogenesis of endothelial injury caused by NETs. Activation of cGAS-STING pathway inhibits endothelial cell proliferation and vascular repair in inflammatory lung injury (Zhu et al., 2023). NETs released dsDNA is a powerful stimula-tory signal of the cytoplasmic cGAS-STING pathway. Recent studies have found that dsDNA activates the cytoplasmic cGAS-STING pathway, leading to lung tissue inflammation and injury, and participating in a variety of lung diseases including allergic asthma and COPD (Zhang et al., 2023; Li et al., 2024). Some scholars have found that H-151 successfully alleviated inflam-matory lung injury in LPS-induced ALI by cGAS-STING pathway (Zhao et al., 2023). Therefore, inhibition of cGAS-STING pathway is a potential target for treating acute lung injury.
It has been confirmed that the release of dsDNA by NETs can activate the STING pathway and cause tissue damage (Wei et al., 2024), and it has also been confirmed that RU.521 can improve the cardiac damage caused by sepsis (Li et al., 2022). However, there are few reports on RU.521 treating acute lung injury or improving vascular endothelial damage. Different from other studies, the purpose of this study is to investigate the effect of RU.521 on improving vascular endothelial injury, which is a potential drug for the treatment of acute lung injury.
However, there are some limitations in the study. First, the study examined only the literature-reported endothelial damage in vascular endothelial cells and did not study alveolar epithelium, macrophages, and other cells. Second, due to the limited research conditions, animal models were not used to verify findings.
Conclusion
NETs release dsDNA activates the cGAS/STING pathway induced vascular endothelial injury in acute lung injury patients. RU.521 protects vascular endothelial injury by inhibiting the cGAS/STING pathway.
References
Decout A, Katz JD, Venkatraman S, Ablasser A. The cGAS-STING pathway as a therapeutic target in inflammatory diseases. Nat Rev Immunol. 2021; 21: 548-69.
Fei Y, Huang X, Ning F, Qian T, Cui J, Wang X, Huang X. NETs induce ferroptosis of endothelial cells in LPS-ALI through SDC-1/HS and downstream pathways. Biomed Pharmacother. 2024; 175: 116621.
Han Y, Eom YS, Shah FH, Kim SJ. Destabilising microtubule polymerization regulates chondrocyte dedifferentiation and inflammation via nuclear factor kappa b and β-catenin pathway. Bangladesh J Pharmacol. 2023; 18: 8-16.
Li G, Liu S, Chen Y, Zhao J, Xu H, Weng J, Yu F, Xiong A, Udduttula A, Wang D, Liu P, Chen Y, Zeng H. An injectable liposome-anchored teriparatide incorporated gallic acid grafted gelatin hydrogel for osteoarthritis treatment. Nat Commun. 2023; 14: 3159.
Li J, Lu Y, Lin G. Blocking cGAS/STING signaling protects against sepsis-associated acute liver injury. Int Immunopharmacol. 2022; 113: 109276.
Li X, Wang Y, Chen Y, Lu Z, Sun Y, Zhong C, Lv Z, Pan H, Chen J, Yao D, Huang X, Yu C. Icariside II alleviates lipopolysaccharide-induced acute lung injury by inhibiting lung epithelial inflammatory and immune responses mediated by neutrophil extracellular traps. Life Sci. 2024; 346: 122648.
Lim HS, Jang Y, Moon BC, Park G. NF-κB signaling contributes to the inhibitory effects of Bombyx batryticatus on neuroinflammation caused by MPTP toxicity. Bangladesh J Pharmacol. 2021; 16: 96-102.
Liu H, Yu X, Yu S, Kou J. Molecular mechanisms in lipopolysaccharide-induced pulmonary endothelial barrier dysfunction. Int Immunopharmacol. 2015; 29: 937-46.
Munshi L, Mancebo J, Brochard LJ. Noninvasive respiratory support for adults with acute respiratory failure. N Engl J Med. 2022; 387: 1688-98.
Qiao X, Yin J, Zheng Z, Li L, Feng X. Endothelial cell dynamics in sepsis-induced acute lung injury and acute respiratory distress syndrome: Pathogenesis and therapeutic implications. Cell Commun Signal. 2024; 22: 241.
Ranieri VM, Rubenfeld GD, Thompson BT, Ferguson ND, Caldwell E, Fan E, Camporota L, Slutsky AS. Acute respiratory distress syndrome: The Berlin definition. JAMA. 2012; 307: 2526-33.
Raybuck AL, Lee K, Cho SH, Li J, Thomas JW, Boothby MR. mTORC1 as a cell-intrinsic rheostat that shapes development, preimmune repertoire, and function of B lymphocytes. FASEB J . 2019; 33: 13202-15.
Uthaman S, Parvinroo S, Mathew AP, Jia X, Hernandez B, Proctor A, Sajeevan KA, Nenninger A, Long MJ, Park IK, Chowdhury R, Phillips GJ, Wannemuehler MJ, Bardhan R. Inhibiting the cGAS-STING pathway in ulcerative colitis with programmable micelles. ACS Nano. 2024; 18: 12117-33.
Wei C, Huang Q, Zeng F, Ma L, Bai X, Zhu X, Gao H, Qi X. Cyclic guanosine monophosphate-adenosine monophosphate synthetase/stimulator of interferon genes signaling aggravated corneal allograft rejection through neutrophil extracellular traps . Am J Transplant. 2024: S1600-6135(24)00281-8.
Xie J, Zhu CL, Wan XJ, Zhao ZZ, Meng Y, Li P, Guo Y, Liu Q, Bian JJ, Deng XM, Wang JF. GSDMD-mediated NETosis promotes the development of acute respiratory distress syndrome. Eur J Immunol. 2023; 53: e2250011.
Xie J, Wang H, Kang Y, Zhou L, Liu Z, Qin B, Ma X, Cao X, Chen D, Lu W, Yao C, Yu K, Yao X, Shang H, Qiu H, Yang Y. Chinese Epidemiological Study of Sepsis (CHESS) study investigators. The epidemiology of sepsis in Chinese ICUs: A national cross-sectional survey. Crit Care Med. 2020; 48: e209-18.
Zhang Y, Li Z, Hong W, Hsu S, Wang B, Zeng Z, Du S. STING-dependent sensing of self-DNA driving pyroptosis contributes to radiation-induced lung injury. Int J Radiat Oncol Biol Phys. 2023; 117: 928-41.
Zhao J, Zhen N, Zhou Q, Lou J, Cui W, Zhang G, Tian B. NETs promote inflammatory injury by activating cGAS-STING pathway in acute lung injury. Int J Mol Sci. 2023; 24: 5125.
Zhu S, Yu Y, Qu M, Qiu Z, Zhang H, Miao C, Guo K. Neutrophil extracellular traps contribute to immunothrombosis formation via the STING pathway in sepsis-associated lung injury. Cell Death Discov. 2023; 9: 315.
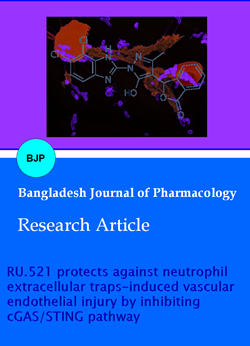