Cytotoxicity of paeonenoide C isolated from Paeonia obovata on HT-29 human colon cancer cells
Abstract
In this study, an alternative approach to activity-based screening was employed to identify potential anti-cancer agent against colon cancer. As a result, Paeonia obovata was selected, and an active compound was isolated from its methanol extracts. Through structural and physicochemical analyses, the compound was identified as paeonenoide C, a triterpenoid. Various cell-based assays, including the MTT reduction assay, LDH release assay, and colony formation assay were conducted to assess its anti-cancer properties. Paeonenoide C exhibited cytotoxic effects in HT-29 human colon cancer cells at concentrations above 150 μM. Western blot analysis demonstrated that paeonenoide C-induced cell cycle arrest and apoptosis by suppressing the phosphorylation of retinoblastoma protein and extracellular signal-regulated kinases 1/2 while down-regulating extracellular signal-regulated kinases 1/2 expression. These findings suggest that paeonenoide C possesses potential anti-cancer activity against colon cancer cell lines.
Introduction
Cancer is a major cause of death in the human popula-tion and its occurrence rate is constantly rising because of Westernized eating habits and an aging population (Chattopadhyay et al., 2004). However, there are no anti-cancer drugs that can effectively inhibit colon cancer, and most existing drugs cause various side effects. Cancer can occur when balance is disturbed by increased cell proliferation or decreased cell death (Kerr et al., 1994). Also, cancer arises from a combination of external and internal factors. External factors include tobacco, exposure to chemicals, radiation, and infec-tious agents, while internal factors encompass inherited genetic mutations, hormones, immune system condi-tions, and mutations resulting from metabolism processes (Kozlenko et al., 2001). Among various cancer types, human colon cancer cells serve as a representa-tive model for solid tumors. These cells often experi-ence inadequate vascularization, leading to regions of hypoxia and nutrient deprivation due to an insufficient blood supply (Tomida and Tsuruo, 1999). Such condi-tions contribute to resistance against several anti-cancer drugs, posing a major challenge in cancer chemothe-rapy. It is well established that both hypoxia and hypo-glycemia contribute to resistance against several anti-cancer drugs, posing a significant challenge in cancer chemotherapy. Due to this issue, extensive research has been dedicated to developing innovative anti-cancer agents that minimize adverse effects and exhibit low toxicity in normal tissues and cells (Tsuruo et al., 2003; Brown and Giaccia, 1998).
To overcome these problems, researchers have explored natural products as potential anti-cancer therapies. Many natural products have demonstrated pharmacological properties and hold promise for use in chemotherapy (Gouaze et al., 2004). Herbal medicines have gained attention due to their relatively low toxicity and significant medicinal benefits (Kodach et al., 2006). To discover novel therapeutic agents targeting HT-29 human colon cancer cells, a screening process was conducted on 450 different herbal medicines. Through this approach, Paeonia obovata was identified as a promising candidate due to its cytotoxic effects on HT-29 cells. Further investigation led to the successful isolation and identification of an active compound within P. obovata responsible for this cytotoxicity.
P. obovata has long been cultivated as an ornamental plant and traditional herbal medicine in East Asia. Although these extracts have been reported to exert various pharmacological effects (Chang et al., 2016; Wu et al., 2021), they have not been previously reported as anti-cancer agents. By examining the structure and physicochemical properties of the isolated active com-pound using electrospray ionization mass (ESI-MS) and nuclear magnetic resonance (NMR) spectroscopy, it was identified as paeonenoide C, a triterpenoid compound (Wu et al., 2005).
In this study, the cytotoxic effects of paeonenoide C on HT-29 cells were assessed and a mechanism by which it induces cell death is proposed.
Materials and Methods
Materials
Kumkang Pharm Co. (Korea) generously provided the P. obovata. HT-29 human colon cancer cells were collected from the Korean Cell Line Bank. 1H NMR (600 MHz), 13C NMR (150 MHz), 2D NMR spectra were obtained on a JEOL JNM-ECA600 spectrometer (Japan), with CD3OD as a solvent. ESI-MS was conducted using a Shimadzu LCMS-IT-TOF mass spectrometer (Japan). Paeonenoide C was dissolved in dimethyl sulfoxide for subsequent experiments. Other organic solvents and chemicals were of analytical grade or complied with the established standards for cell culture experiments. Sigma Chemical Co provided reagents MTT (3-[4,5-dimethylthiazol-2-yl]-2,5-diphenyl tetrazolium bromide) and dimethyl sulfoxide.
Isolation and purification of paeonenoide C from P. obovata extracts
Dried P. obovata was extracted with methanol at room temperature for three days. After concentrating under reduced pressure using a rotary evaporator, the remain-ing aqueous phase was extracted with diethyl ether. The organic layer was further concentrated, and the resulting dried residue was subjected to silica gel column chromatography. The column was initially elu-ted with a stepwise solvent system of chloroform-methanol (50:1 to 1:1 [v/v]). The bioactive fraction eluted at a 20:1 ratio was then purified through another silica gel column, employing a linear gradient of chloroform-methanol (20:1). Further purification was performed using reverse-phase high-performance liquid chromatography with aqueous methanol (80%) as a mobile phase, ultimately yielding the active com-pound (about 0.0003%).
Cell culture and treatment
They were cultured in RPMI1640 medium (Invitrogen, USA) that was supplemented with fetal bovine serum (10%), penicillin (100 U/mL), streptomycin (100 μg/mL), and sodium bicarbonate (2 mg/mL). The cells were maintained in an incubator with 5%CO2 at 37˚C. Paeonenoide C was added into the culture medium at various concentrations, ensuring that its volume did not exceed 1% of the total medium volume.
MTT reduction assay
An MTT assay was performed for testing cell viability (Fisher, 1984). HT-29 cells were plated in 96-well plates at a density of 1 × 104 cells/well and maintained in RPMI1640 medium for 24 hours. Subsequently, cells were exposed to different concentrations of paeone-noide C for another 24 hours. Then MTT solution [5 mg/mL, dissolved in PBS(-) buffer] was added to each well, and the plate was incubated at 37℃ for another 1 hour. The medium was carefully removed. The intracellular-formed formazan was solubilized using dimethyl sulfoxide (100 μL). The absorbance was measured using an ELISA reader (Model 680, BioRad, Hercules, USA) at 540 nm to determine the cell viability.
Lactate dehydrogenase (LDH) release assay
The cytotoxicity was assessed by measuring the release of LDH using an LDH release assay kit (Wako Pure Chemical Industries, Japan). The cells were exposed to paeonenoide C (different concentrations) and incubated for 24 hours. After incubation, the cell-free supernatant (50 μL) was mixed with potassium phosphate buffer containing sodium pyruvate and nicotinamide adenine dinucleotide in a 96-well plate, making a total volume of 100 μL. The reaction was, then, assessed using a colorimeter, where the formation of formazan salt was directly to LDH activity. The intensity of the red coloration was measured at 540 nm which reflects the level of LDH activity. The amount of formazan salt was proportional to the level of LDH activity in the sample (Decker and Lohmann-Matthes, 1988).
Colony formation assay
A colony formation assay was performed due to its higher sensitivity to other cytotoxicity evaluation methods. In brief, cells were seeded at a density of 1 × 105 cells/well in 24-well plates and incubated for 24 hours. Then, cells were exposed to different concentrations of paeonenoide C for 24 hours. After treatment, the cells were washed, resuspended in a fresh medium without paeonenoide C, and reseeded at a density of 1 x 103 cells/well in 6-well plates. They were then maintained under standard culture conditions for 7-8 days to enable colony formation. The colonies were subsequently fixed using formaldehyde (10%), stained with crystal violet (0.01%), and counted for analysis (Park et al., 2004).
Observation of morphological changes
HT-29 cells were plated at a density of 2 × 105 cells/well in 6-well plates and allowed to adhere overnight in a humidified incubator maintained at 37˚C with 5% CO2. Following incubation, the cells were exposed to differ-ent concentrations of paeonenoide C. After 24 hours of treatment, changes in cellular morphology were examined using phase-contrast microscopy (Nikon, Japan).
Western blot analysis
Whole-cell lysates were obtained by lysing the cells in 1 × sodium dodecyl sulfate (SDS) sample buffer (62.5 mM Tris-HCl, pH 6.8, 2% SDS, 10% glycerol, and 5% 2-mercaptoethanol) following a previously described (Ogiso et al., 2000). Protein samples were then separa-ted using SDS-polyacrylamide gel electrophoresis (SDS-PAGE) and transferred onto a nitrocellulose membrane (BioRad) for western blot analysis. Membranes were probed with primary antibodies, including a mouse monoclonal anti-retinoblastoma protein (Rb) antibody (BD Bioscience, USA) at a dilution 1:500, a rabbit monoclonal anti-ERK1/2 antibody conjugated to horseradish peroxidase (HRP) (Cell Signaling, USA) at 1:1000, a rabbit monoclonal anti-p-ERK1/2 antibody (Cell Signaling) at 1:1000, and mouse monoclonal anti-β-actin antibody (Sigma-Aldrich, USA) at 1:1000. For secondary detection, HRP-conjugated anti-mouse IgG (Amersham Pharmacia Biotech, Japan) at 1:500, and HRP-conjugated anti-rabbit IgG (Amersham) at 1:500 were used. β-actin was used as a loading control. Signal detection was performed using an enhanced chemiluminescence detection kit (Amersham Pharmacia Bio-tech) according to the manufacturer’s protocol.
Results
Structure elucidation of paeonenoide C
ESI-MS was performed in the negative mode to determine the molecular weight of paeonenoide C. The [M–H]⁻ ion was observed at m/z 441, indicating a molecular weight of 442.
To elucidate the structure of paeonenoide C, the 1H-NMR spectrum was determined. The spectrum revealed one olefinic methine proton at 5.29 ppm, one exome-thylene proton at 4.57 ppm, one oxygenated methine proton at 3.19 ppm, numerous methylene and methine protons between 0.81 and 2.74 ppm, and four methyl protons between 0.88 and 1.21 ppm.
In the 13C-NMR spectrum, four olefinic carbons were observed at 150.3, 145.2, 123.8, and 106.8 ppm; two oxygenated carbons at 76.8 and 74.9 ppm, and multiple methine, methylene, and methyl carbons in the range of 15.5–55.0 ppm.
To further determine the partial structures of paeone-noide C, a 1H–1H correlation spectroscopy (COSY) spec-trum was obtained. A cross peak between the exomethylene proton at 4.57 ppm and the methylene protons at 2.74 and 2.09 ppm indicated that these protons were positioned at a hetero-allylic site, observable via allylic coupling. To confirm the connectivity between the partial structures identified by the COSY spectrum, heteronuclear multiple bond correlation (HMBC) spectra were recorded and interpreted. The significant long-range correlations identified include those from a methylene proton at 1.65 ppm to a methine carbon at 55.0 ppm and a quaternary carbon at 37.9 ppm. A methine proton at 1.58 ppm exhibited correlations with a methine carbon at 55.0 ppm, a methylene carbon at 33.9 ppm, quaternary carbons at 40.5, 37.9, and 43.0 ppm, and a methyl carbon at 15.5 ppm. Methylene protons at 1.90 and 1.94 ppm show correlations with an olefinic quaternary carbon at 145.2 ppm. A methine proton at 2.74 ppm was correlated with an olefinic methine carbon at 123.8 ppm, an olefinic quaternary carbon at 145.2 ppm, and a methylene carbon at 24.4 ppm. A methyl proton at 1.21 ppm exhibited correlations with an oxygenated methine carbon at 76.8 ppm, an oxygenated quaternary carbon at 74.9 ppm, and a methine carbon at 55.0 ppm. Another methyl proton at 0.88 ppm was linked to a methylene carbon at 33.9 ppm and a quaternary carbon at 40.5 ppm. Similarly, a methyl proton at 1.18 ppm shows correlations with an olefinic quaternary carbon at 145.2 ppm, a quaternary carbon at 43.0 ppm, and a methylene carbon at 29.0 ppm. Lastly, an exomethylene proton at 4.57 ppm was correlated with methylene carbons at 43.1 and 31.3 ppm. These long-range correlations successfully connected the previously determined partial structures, ultimately leading to the complete elucidation of the chemical struc-ture of the paeonenoide C (Figure 1).
Figure 1: Chemical structure of paeonenoide C
Cytotoxicity in HT-29 human colon cancer cells
Cell viability assays were conducted to evaluate the cytotoxic effects of the paeonenoide C on HT-29 cells. Initially, phase-contrast microscopy was used to examine the morphological change in HT-29 cells following paeonenoide C treatment. After 24 hours of incubation with varying concentrations of paeonenoide C (10, 50, 100, 150, and 200 μM), noticeable cytoplasmic shrin-kage, cell detachment, and floating cells were observed at concentrations exceeding 100 μM (Figure 2). The impact of paeonenoide C on cell viability and cytotoxicity was further analyzed using the MTT reduction and LDH release assays. Consistent with the morphological observations, the MTT confirmed a significant reduc-tion in cell viability at paeonenoide C concentrations of 150 and 200 μM (Figure 3A). Similarly, the LDH release assay demonstrated a marked increase in cytotoxicity, with LDH levels rising by approximately 80% compared to the control at these concentrations (Figure 3B). Additionally, a colony formation assay was performed to further assess the cytotoxic effects of paeonenoide C on HT-29 cells. The results revealed a substantial reduc-tion in colony formation at paeonenoide C concentration above 100 μM, indicating its strong cytotoxic potential (Figure 3C). Collectively, these findings confirm that paeonenoide C exhibits significant toxicity toward HT-29 cells.
Figure 2: Paeonenoide C-induced cell death in HT-29 human colon cancer cells. HT-29 cells were exposed to paeonenoide C for 24 hours (A, control; B, 10 μM; C, 50 μM; D, 100 μM; E, 150 μM; F, 200 μM). Photographs were taken at a magnification of x100. The results are representative of three independent experiments
Figure 3: Effects of paeonenoide C (PNC) on viability and cytotoxicity (A), LDH release (B), and survival rate (C) in HT-29 cells. The cells were exposed to the indicated various concentrations (10, 50, 100, 150, and 200 μM) of PNC for 24 hours. Cell viability and cell death were measured with the MTT reduction assay and LDH release assay, respectively. After the MTT reduction assay, the MTT reduction rate was calculated by setting each of the control survivals. For the results of the LDH release assay, the data were normalized to the activity of LDH released from vehicle-treated cells (100%), and expressed as a percentage of the control (obtained separate plating). Data are means ± SD of triplicate determinations
Phosphorylation of Rb protein and ERK1/2 and the expression of ERK1/2 in HT-29 cells
Since paeonenoide C exhibits a cytotoxic effect on HT-29 cells, a western blot analysis was carried out to deter-mine whether paeonenoide C influences the expression and phosphorylation of specific proteins. Whole-cell lysate from cells treated with different concentrations of paeonenoide C were subjected to western blotting. In this experiment, the hyperphosphorylated form of Rb protein was observed in paeonenoide C-treated cells at low concentrations (10, 50, and 100 μM); however, hyperphosphorylated Rb was markedly decreased, and hypophosphorylated Rb was increased by treatment with 150 and 200 μM of paeonenoide C (Figure 4). This result indicates that paeonenoide C inhibits the phos-phorylation of Rb protein and induces cell cycle arrest in the G1 phase. Paeonenoide C blocked the phospho-rylation of ERK1/2 proteins at concentrations greater than 100 μM and that the expression level of ERK1/2 was decreased by paeonenoide C treatment (150 and 200 μM). These results indicate that paeonenoide C exerts apoptotic effects on HT-29 cells by blocking the phosphorylation of ERK1/2 and down-regulating ERK1/2 proteins.
Figure 4: Paeonenoide C (PNC) inhibits phosphorylation of Rb and ERK1/2 and down-regulates expression of ERK1/2. Total protein lysates were prepared from PNC-treated HT-29 cells. The protein samples (10 μL/lane) were subjected to SDS-PAGE and immunoblotted using mouse monoclonal Rb, rabbit monoclonal ERK1/2 (HRP conjugated), rabbit monoclonal p-ERK1/2, and monoclonal anti-b-actin antibodies, respectively
Discussion
Recently, 5-fluorouracil and leucovorin have been widely used as representative chemotherapeutic drugs for colorectal cancer treatment (de Garamont et al., 2000). However, these drugs exhibit side effects on blood cells, epithelial cells, and the nervous system, highlighting the need to develop anti-cancer agents that can effectively inhibit cancer cells while minimizing adverse effects (Maroon et al., 2007). Therefore, research into the development of anti-cancer agents from natural products is actively being conducted (Kinghorn et al., 2009; Lee, 2010; da Rocha et al., 2001). In this study, a report is provided on paeonenoide C, a compound isolated from P. obovata extracts, which has demons-trated significant anti-cancer activity against colorectal cancer cells.
P. obovata is often used as a substitute for P. lactiflora and P. veitchii and its roots are dried primarily for medicinal use (Halina et al., 2002). These herbal medicines have been reported to exhibit anti-inflammatory, antiviral, antibacterial, and antioxidant effects, which are mainly attributed to the biosynthesis of compounds such as flavonoids, polyphenols, and monoterpenes (Koo et al., 2010). Studies of the biological activities of paeonenoide C isolated from P. obovata have primarily focused on their hepatoprotective and anti-inflammatory properties (Fan et al, 2024). How-ever, no studies have reported the anti-cancer activities of paeonenoide C.
Various biological experiments were conducted to evaluate the cytotoxic effect of paeonenoide C on HT-29 human colon cancer cells. Microscopic observations revealed noticeable morphological changes in treated cells. Specifically, when exposed to paeonenoide C concentrations exceeding 100 μM, the cells exhibited cytoplasmic shrinkage and either detached from neigh-boring cells or floated in the culture medium. Additionally, the MTT reduction assay was performed to assess cell viability following paeonenoide C treatment. The result demonstrated that paeonenoide C signifi-cantly reduced the viability of HT-29 cells, particularly at concentrations of 150 and 200 μM. A similar trend was observed in the LDH leakage assay, where a higher concentration of paeonenoide C led to increased LDH release into the medium. These findings suggest that paeonenoide C exerts strong cytotoxic effects on HT-29 cells in a dose-dependent manner. The cytotoxic effects of paeonenoide C were confirmed using colony-formation assays. Based on these results, paeonenoide C was found to be highly cytotoxic to HT-29 cells.
The Rb protein, first identified in retinoblastoma, plays an important inhibitory role in controlling cell cycle progression in the G1/S phase (Kamb, 1995; Lim et al., 2007). Phosphorylation of Rb results in the release of active transcription factors that stimulate the transcrip-tion of genes involved in DNA synthesis and S phase progression (Knudsen and Knudsen, 2006; Weinberg, 1995). Rb exists in a hypophosphorylated form and blocks the binding of transcription factor E2F in the G0/G1 phase (Kwon et al., 2010). ERK1/2 proteins are key regulators of various cellular processes such as proliferation, differentiation, and survival. Especially, it is widely known that phosphorylated ERK1/2 play a role as anti-apoptotic enzymes (Brown and Sacks, 2009; Roskoski, 2012). Therefore, if RB phosphorylation is inhibited, inhibitory effects on cell proliferation can be expected via cell cycle arrest, especially G1 arrest, in cancer cells (Jedinak and Sliva, 2008). Paeonenoide C exhibited a cytotoxic effect on HT-29 cells and suppe-ssed Rb protein phosphorylation, indicating its role in inducing G1 phase arrest and inhibiting HT-29 cell proliferation by preventing Rb phosphorylation. Thus, in turn, it led to the blockade of the transcription factor E2F. Western blot analysis further demonstrated that paeonenoide C treatment reduced both expression and phosphorylation levels of ERK1/2 in HT-29 cells. Given that ERK1/2 play a crucial role in regulating cell growth, differentiation, and survival (Pozzi et al., 2004; Sun and Sinicrope, 2005; Yang et al., 2006), their inhibition by paeonenoide C contributed to the suppression of HT-29 cells proliferation observed in this study.
Conclusion
The triterpenoid compound paeonenoide C exhibited potential anti-cancer properties, presenting a promising new approach for colon cancer treatment.
Ethical Issue
In the study, the guidelines about the development, acquisition, authentication, cryopreservation, and transfer of cell lines between laboratories were strictly followed. Besides, microbial contamination (commonly mycoplasma), characterization, instability, and misidentification was considered seriously.
References
Brown JM, Giaccia AJ. The unique physiology of solid tumors: Opportunities (and problems) for cancer therapy. Cancer Res. 1998; 58: 1408-16.
Brown MD, Sacks DB. Protein scaffolds in MAP kinase signaling. Cell Signal. 2009; 21: 462-69.
Chang X, Wang S, Bao YR, Li TJ, Yu XM, Meng XS. Multicomponent, multitarget integrated adjustment: Metabolomics study of qizhiweitong particles curing gastrointestinal motility disorders in mice induced by atropine. J Ethnopharmacol. 2016; 189: 14-21.
Chattopadhyay S, Bisaria VS, Panda AK, Srivastava AK. Cytotoxicity of in vitro produced podophyllotoxin from Podophyllum hexandrum on human cancer cell line. Nat Prod Res. 2004; 18: 51-57.
da Rocha AB, Lopes RM, Schwartsmann G. Natural products in anti-cancer therapy. Curr Opin Pharmacol. 2001; 1: 364-69.
Decker T, Lohmann-Matthes ML. A quick and simple method for the quantitation of lactate dehydrogenase release in measurements of cellular cytotoxicity and tumor necrosis factor (TNF) activity. J Immunol Methods. 1988; 115: 61-69.
de Gramont A, Figer A, Seymour M, Homerin M, Hmissi A, Cassidy J, Boni C, Cortes-Funes H, Cervantes A, Freyer G, Papamichael D, Le Bail N, Louvet C, Hendlern C, Morvan F, Bonetti A. Leucovorin and fluorouracil with or without oxaliplatin as first-line treatment in advanced colorectal cancer. J Clin Oncol. 2000; 18: 2938-47.
Fan Z, Liu J, Wang X, Yang S, Wang Q, Yan L, Zhang Y, Wu X. Paeoniae radix Rubra: A review of ethnopharmacology, phytochemistry, pharmacological activities, therapeutic mechanism for blood stasis syndrome, and quality control. Chem Biodivers. 2024; 21: e202401119.
Fisher B. Clinical trials for the evaluation of cancer therapy. Cancer 1984; 54: 2609-17.
Gouaze V, Yu JY, Bleicher RJ, Han TY, Liu YY, Wang H, Gottesman MM, Bitterman A, Giuliano AE, Cabot MC. Overexpression of glucosylceramide synthase and P-glycoprotein in cancer cells selected for resistance to natural product chemotherapy. Mol. Cancer Ther. 2004; 3: 633-39.
Halina E, Marta K, Agnieszka S. Paeonia × suffruticosa (Moutan Peony): A review of the chemical composition, traditional and professional use in medicine, position in cosmetics industries, and biotechnological studies. Plants 2002; 11: 3379.
Jedinak A, Sliva D. Pleurotus ostreatus inhibits proliferation of human breast and colon cancer cells through p53-dependent as well as p53-independent pathway. Int J Oncol. 2008; 33: 1307-13.
Jemal A, Siegel R, Xu J, Ward E. Cancer statistics. CA Cancer J Clin. 2010; 60: 277-300.
Kamb A. Cell-cycle regulators and cancer. Trends Genet. 1995; 11: 136-40.
Kerr JF, Winterford CM, Harmon BV. Apoptosis: Its significance in cancer and cancer therapy. Cancer 1994; 73: 2013-26.
Kinghorn AD, Chin YW, Swanson SM. Discovery of natural product anti-cancer agents from biodiverse organisms. Curr Opin Drug Discov Devel. 2009; 12: 189-96.
Knudsen ES, Knudsen KE. Retinoblastoma tumor suppressor: Where cancer meets the cell cycle. Exp Biol Med. 2006; 231: 1271-81.
Kodach LL, Bos CL, Duran N, Peppelenbosch MP, Ferreira CV, Hardwick JCH. Violacein synergistically increases 5-fluorouracil cytotoxicity, induces apoptosis and inhibits Akt-mediated signal transduction in human colorectal cancer cells. Carcinogenesis 2006; 27: 508-16
Koo YK, Kim JM , Koo JY, Kang SS, Bae KH, Kim YS, Chung JH, Yun-Choi HS. Platelet anti-aggregatory and blood anti-coagulant effects of compounds isolated from Paeonia lactiflora and Paeonia suffruticosa. Pharmazie 2010; 65: 624-28.
Kozlenko M, Vol A, Zusman I. Stability-variability conflict as a reason for cancer: Physical and social aspects. Med Hypotheses. 2001; 57: 648-54.
Kwon HJ, Hong YK, Park C, Choi YH, Yun HJ, Lee EW, Kim BW. Widdrol induces cell cycle arrest, associated with MCM down-regulation, in human colon adenocarcinoma cells. Cancer Lett. 2010; 290: 96-103.
Lee KH. Discovery and development of natural product-derived chemotherapeutic agents based on a medicinal chemistry approach. J Nat Prod. 2010; 73: 500-16.
Lim do Y, Jeong Y, Tyner AL, Park JH. Induction of cell cycle arrest and apoptosis in HT-29 human colon cancer cells by the dietary compound luteolin. Am J Physiol Gastrointest Liver Physiol. 2007; 292: G66-75.
Maroun JA, Anthony LB, Blais N, Burkes R, Dowden SD, Dranitsaris G, Samson B, Shah A, Thirlwell MP, Vincent MD, Wong R. Prevention and management of chemotherapy-induced diarrhea in patients with colorectal cancer: A consensus statement by the Canadian working group on chemotherapy-induced diarrhea. Curr Oncol. 2007; 14: 13-20.
Ogiso Y, Tomida A, Lei S, Omura S, Tsuruo T. Proteasome inhibition circumvents solid tumor resistance to topoisomerase ІІ-directed drugs. Cancer Res. 2000; 60: 2429-34.
Park HR, Tomida A, Sato S, Tsukumo Y, Yun J, Yamori T, Hayakawa Y, Tsuruo T, Shinya K: Effect on tumor cells of blocking survival response to glucose deprivation. J Natl Can Inst 2004; 96: 1300-10.
Pozzi A, Yan X, Macias-Perez I, Wei S, Hata AN, Breyer RM, Morrow JD, Capdevila JH. Colon carcinoma cell growth is associated with prostaglandin E2/EP4 receptor-evoked ERK activation. J Biol Chem. 2004; 279: 29797-804.
Roskoski R Jr. ERK1/2 MAP kinases: Structure, function, and regulation. Pharmacol Res. 2012; 66: 105-43.
Sun Y, Sinicrope FA. Selective inhibitors of MEK1/ERK44/42 and p38 mitogen-activated protein kinases potentiate apoptosis induction by sulindac sulfide in human colon carcinoma cells. Mol Cancer Ther. 2005; 4: 51-59.
Tomida A, Tsuruo T. Drug resistance mediated by cellular stress response to the microenvironment of solid tumors. Anticancer Drug Des. 1999; 14: 169-77.
Tsuruo T, Naito M, Tomida A, Fujita N, Mashima T, Sakamoto H, Haga N. Molecular targeting therapy of cancer: Drug resistance, apoptosis and survival signal. Cancer Sci. 2003; 93: 15-21.
Weinberg RA. The retinoblastoma protein and cell cycle control. Cell 1995; 81: 323-30.
Wu L, Nie L, Wang Q, Xu Z, Wang Y, He C, Song J, Yao H. Comparative and phylogenetic analyses of the chloroplast genomes of species of Paeoniaceae. Sci Rep. 2021; 11: 14643.
Wu, S.H., Yang, S.M., Wu, D.G., Cheng, Y.W., Peng, Q. 2005. Three novel 24,30-dinortriterpenoids, paeonenoides A-C, from Paeonia veitchii. Helv. Chim. Acta. 2005; 88: 259-65.
Yang SA, Paek SH, Kozukue N, Lee KR, Kim JA. Alpha-chaconine, a potato glycoalkaloid, induces apoptosis of HT-29 human colon cancer cells through caspase-3 activation and inhibition of ERK1/2 phosphorylation. Food Chem Toxicol. 2006; 44: 839-46.
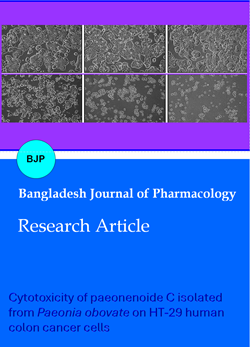