Carvacrol enhances the sensitıvity of cetuximab in lung cancer cells through inducing apoptosis and cell cycle arrest
Abstract
The intention of this work was to analyze that carvacrol could enhance cancer-inhibiting potency of cetuximab (monoclonal antibody) in lung cancer cells. The combination of cetuximab and carvacrol was found to co-operatively suppress cell proliferation by enhancing apoptosis and inducing cell cycle arrest in A-549 and H1299 cells. Furthermore, the combination therapy triggered cell death by inducing membrane disruption. The most effective combinations were IC₁₀ cetuximab + IC₁₀ carvacrol for A-549 and IC₂₀ cetuximab + IC₁₀ carvacrol for H1299 (CI = 2.0). LDH activity increased by 101% in A-549 and 239% in H1299 (p<0.05). Caspase-3 activity rose by 1.6-fold in A-549 and 1.4-fold in H1299 (p<0.05). The combination decreased PCNA, topoisomerase II-alpha, and cyclins D1, D2, E, A, and B (p<0.05). Overall, the combination of carvacrol and cetuximab appears to enhance anti-cancer efficacy and may significantly improve therapeutic outcomes in lung cancer cells.
Introduction
Lung cancer accounts for 12.8% of cancer diagnoses and 17.8% of cancer-related deaths globally (Subak et al., 2020). Lung cancer is attributed to smoking in 94% of cases, while the remaining 6% is linked to various factors including age, race, gender, occupation, air pollution, genetics, and radiation (Alar and Şahin, 2012). Two major types of lung cancers are recognized: small cell (SCLC) and non-small cell (NSCLC).
While some standards have been established, various approaches and treatments are implemented for each type of cancer. As modern medicine has advanced, cancer patients are increasingly seeking alternative treatment methods rather than relying solely on chemotherapy, radiotherapy, and surgery (Baykara, 2016). Despite being treated with surgical methods, chemotherapy, and radiotherapy, the survival rate for patients with this type of cancer remains quite low. Consequently, it is essential to investigate the genetic development of lung cancer and to explore new treatment methods (Zamani and Zamani, 2013). A new avenue in lung cancer therapy being investigated is the synergistic use of natural compounds and conventional anti-cancer drugs.
One of the natural compounds evaluated for therapeutic purposes is carvacrol. Carvacrol [2-methyl-5-(1-methylethyl)-phenol] is an antioxidant monoterpene phenolic substance detected in the volatile oils from aromatic plants such as thymus, peppercorn and wild bergamot, which has a curative effect on various ailments (Arkali et al., 2021). The consumption of carvacrol is typically deemed to be safe. It is recognized by the Food and Drug Administration (FDA) as safe for use in food grade substances (Ultee et al., 1999). Multiple studies have focused on the antibacterial (Rattanachaikunsopon and Phumkhachorn, 2010; Ravishankar et al., 2010; Rivas et al., 2010), antifungal (Chami et al., 2010), antiviral (Pilau et al., 2011), immunomodulatory (Hashemipour et al., 2013), and antioxidant properties (Alma et al., 2003; Radonic and Milos, 2003) of carvacrol, highlighting its therapeutic benefits for a range of conditions (Arunasree, 2010; Jayakumar et al., 2012; Melo et al., 2014). Recent findings show that people who frequently consume fruits and vegetables abundant in antioxidants exhibit a reduced chance of developing ailments like cardiovascular disease and cancer (Yaman et al., 2018). It is recognized that polyphenol compounds, particularly noted for their strong properties that combat oxidative damage, fulfill a pivotal role in protecting individuals from diseases, and research in this area continues to grow daily (Njume et al., 2009). The oxidative stress-reducing properties of carvacrol have been established not only in a laboratory setting but also in preclinical models of inflammation of the pancreas, liver toxicity, and hepatocellular carcinoma. Several laboratory setting studies have indicated that carvacrol may possess strong cancer-suppressing activity. It can alleviate oxidative stress by boosting enzymatic protection against oxidative stress like superoxide dismutase, catalase, and glutathione, while also neutralizing free radicals such as superoxide and hydrogen peroxide (Lee et al., 2020). It was found that the testicular cells and liver DNA of rats treated with carvacrol water were more resistant to hydrogen peroxide oxidation compared to the control group (Boduroglu, 2022). In a trial using MDA-MB 231 cell line, obtained from breast carcinoma, carvacrol was found to exhibit anti-carcinogenic effects (Arunasree, 2010).
Cetuximab (IMC-C225, Erbitux; ImClone Systems Inc., New York) is a chimeric human-murine IgG1 monoclonal antibody that targets outer domain of epidermal growth factor receptor (EGFR) (Gill et al., 1984). Cetuximab has a binding affinity to EGFR that is ten times greater than that of endogenous ligands like EGF and Transforming Growth Factor-α (TGF-α). This binding inhibits receptor tyrosine kinase activity and the associated MAPK, PI3K/Akt, and Jak/Stat pathways (Herbst and Shin, 2002). When it binds to EGFR, various pro-apoptotic pathways are activated within the cell (Cheng et al., 2013). Laboratory studies have illustrated that cetuximab exhibits cytotoxic and antiproliferative effects. Moreover, cetuximab has been evidenced to suppress tumor progression in engrafted tumor models (Wu et al., 1995; Baselga, 2001; Overholser et al., 2000). Furthermore, studies in mice have indicated that cetuximab can improve the tumor-suppressing effects of various chemotherapeutic agents and radiotherapy (Buchsbaum et al., 2002; Bruns et al., 2000). They reported that combination therapy with 5-fluorouracil led to greater regression of tumor growth in vivo (Overholser et al., 2000). Following the completion of chemotherapy, cetuximab administration is typically continued until disease progression or unacceptable toxicity occurs. As with other EGFR-targeted therapies, the primary adverse effects include acne-like skin rash and diarrhea. Recently, numerous researchers have revealed outcomes in both laboratory conditions and living organisms cancer scientific inquiries that combining chemotherapeutic agents with herbal compounds can yield additive or synergistic anti-cancer effects. Given the harmlessness and tumor-suppressing properties of botanical ingredients like carvacrol, such phenolic monoterpene agent may offer a harmless and low-cost therapeutic approach for lung cancer patients by mitigating the adverse effects of chemotherapeutics such as cetuximab.
The intention of this study was to investigate the cell-destructive, membrane-impairing, antioxidant, apoptotic repercussions and mechanisms of intervention of cetuximab and carvacrol on lung transformed cells (A-549 and H1299) in vitro. As a result of this study, the aim was to propose a new alternative treatment strategy for cancer patients by suggesting that lower doses of cetuximab could be used for those experiencing adverse effects from the drug.
Materials and Methods
Chemical agents and pharmaceutical
Cetuximab (referred to by several names, including C225-03, IMC-C225, C225, and ch225) was supplied by Merck Serono (USA). Drugs were immediately diluted before being added to the growth substrate. Carvacrol was supplied by Sigma Chemical Co. (282197, Spain) and had 98% purity. Colorimetric caspase-3 activity assay kit was kindly provided by Elabscience Biotechnology Co., Ltd (USA). Activity of lactate dehydrogenase evaluation kit (MAK066) was supplied by Sigma-Aldrich (USA). RNeasy mini kit, utilized for RNA isolation with high purity from cells, was provided by Qiagen (USA). Titan one tube RT-PCR system kit, used for RT-PCR, was supplied by Roche Applied Science (Germany). 3-(4,5-Dimethylthiazol-2-yl)-2,5-diphenyltetrazolium bromide (MTT) was pur-chased from Gibco (USA)
Cells used in the study
H1299 and A-549 used in the study were commercially obtained from the American Type Culture Collection. Both cells used in the studies are members of the cell lines from human non-small-cell lung carcinoma (NSCLC). All tested cellular models reproduced under suitable conditions. Cells were propagated in Roswell Park Memorial Institute 1640 (RPMI 1640) medium and other medium components in appropriate proportions. After the cells reach sufficient density (more than 75%) in the culture vessel, experimental groups were created and then monoclonal antibody (cetuximab) (<IC50), and natural monoterpenic phenol (carvacrol) (<IC50), were introduced to the cell cultures for a duration of 48 hour. When comparing A-549 cells and H1299 cells in terms of p53 gene, it is known that A-549 cells have an intact p53 gene, while this situation is the opposite in H1299 cells.
Cytotoxicity assay
H1299 and A-549 cells (1 x 104) were plated per well (200 μL) in a medium. Cell viability was determined after cetuximab and carvacrol were exposed to the cells over a 48-hour period. The metabolic activity of cells, indicative of living cell count, was established at 48 hour using MTT assay. The working principle of the MTT test is dependent on the conversion of MTT into insoluble formazan by viable cells. After the test reagents were dispensed into the medium and processed for an incubation period of 2 hours at 37°C degrees, the reaction was terminated through incorporating dimethyl sulfoxide (DMSO) and incubation was carried out for another 1 hour. Afterwards, cytotoxicity was determined by taking measurements at 490 nm (Mosmann, 1983). Eight wells were replicated for each concentration. IC50 values (the concentrations that lead to 50% growth inhibition) and lower IC50 concentrations (<IC50) were calculated separately for cetuximab and carvacrol. The combination treatments were then carried out using these calculated lower IC50 values. Ultimately, the most therapeutically effective concentration pairs were identified based on cell viability results after administering cetuximab (<IC50) and carvacrol (<IC50) collectively. The combination index (CI) values were determined to investigate the nature of the interaction—additive, synergistic, or antagonistic—between cetuximab and carvacrol in cell-based assays. The formula used to calculate the CI is given below:
CI =(Ea + b)/(Ea + Eb - Ea × Eb) (Huang et al., 2014)
where, Ea + b indicates the extent of suppression observed when both carvacrol and cetuximab are administered together, Ea refers to the inhibition rate by carvacrol alone, and Eb represents the inhibition rate by cetuximab alone.
The effect of drug co-administration was classified as follows: a) additive (+) for CI values between 0.85 and 1.15; b) synergistic (++) for CI values between 1.15 and 2.0; c) subtractive (-) for CI values between 0.85 and 0.55; and d) antagonistic (—) for CI values under 0.55. Control groups consisted of cells that were not exposed to any treatment and those treated with 0.1%DMSO. Data were collected from eight independent repetitions.
Lactate dehydrogenase (LDH) enzyme activity
LDH activity was measured after treating A-549 and H1299 cells with cetuximab alone (<IC50) and a combination of carvacrol (<IC50) concentrations, which exhibited the strongest cytotoxic effects, for 48 hours. Thus, an attempt was made to reveal the destructive effect on the membrane by examining the fluctuations in LDH activity. LDH activity was measured using a commercially available kit as outlined by the application operating procedures. In this test, LDH facilitates the conversion of NAD to NADH, which is then measured colorimetrically at 450 nm. The formula used to calculate LDH activity is given below:
LDH activity = The amount of NADH that occurs between the first and last measurement (nmol) × Sample dilution factor/Reaction time × Sample volume (mL)
Box I: Glutathione peroxidase activity
Principle
Glutathione peroxidase (GPx) activity is measured spectro-photometrically by coupling the enzymatic reduction of tert-butyl hydroperoxide with the oxidation of reduced glutathione (GSH). The oxidized glutathione (GSSG) is then regenerated to GSH by glutathione reductase in the presence of NADPH. The rate of decrease in absorbance at 340 nm, due to the oxidation of NADPH to NADP+, is directly proportional to the GPx activity in the sample.
Requirements
A-549 cell; Bovine serum albumin (BSA); Bradford reagent; Carvacrol; Centrifuge; Cetuximab; Glutathione reductase; H1299 cell; NADPH; Phosphate buffered saline (PBS); Reduced glutathione; Tert-butyl hydroperoxide; Ultrasonicator; UV-Vis spectrophotometer
Procedure
Step 1: A-549 and H1299 cells were cultured and treated for 48 hours with cetuximab alone and also together with carvacrol (using the most effective combination concentration based on prior cytotoxicity assay).
Step 2: Following treatment, cells were harvested and centrifuged at 1,500 x g for 10 min at 4°C.
Step 3: The pellet was resuspended in ice-cold PBS and lysed via ultrasonication on ice (3-4 pulses of 10 sec each, with 30 sec intervals).
Step 4: The lysate was centrifuged at 12,000 x g for 15 min at 4°C to remove cell debris.
Step 5: The clear supernatant was collected and used for both GPx activity assay and total protein quantification.
Calculation
The decrease in absorbance at 340 nm was monitored for 5 min at 25°C using a UV-Vis spectrophotometer. GPx activity was calculated based on the rate of NADPH consumption. Total protein concentration of the lysates was determined using the Bradford method, with BSA as the standard. All samples were analyzed in triplicate. One unit of GPx activity is defined as the amount of enzyme that catalyzes the oxidation of 1 µmol of NADPH per min under assay conditions.
References
Bradford, 1976; Flohe and Gunzler, 1984
Caspase-3 activity
By examining the changes in caspase-3 activity, a crucial enzyme in the apoptosis process, after 48 hours of application of cetuximab alone and also in combination with carvacrol (at the concentration combination that showed the highest cytotoxic effect), the apoptotic effects of both alone and combined application on cells were tried to be explained. Apoptotic enzyme performance was measured through a commercially available colorimetric based caspase-3 activity detection kit (Elabscience), following the provided protocol. The specimens were analyzed at 405 nm with the aid of a microplate reader. The tests were conducted in three repetitions. The results are expressed as units per milligram of protein.
RNA isolation and gene transcriptional activity
Reverse transcription-based polymerase chain reaction (RT-PCR) method was implemented to reveal whether there was a change in the transcriptional levels of expression of many genes– proliferating cell nuclear antigen (PCNA) and various cyclins (D1, E, A, D2, B), topoisomerase II-alpha, Bax, and Bcl-2) in H1299 and A-549 cells.
RNA expression was analyzed after alone or in combination with carvacrol (at the most effective cytotoxic concentration) in H1299 and A-549 cells. Gene expression analysis was carried out in three separate repetitions. Total RNA was isolated after 48 hours of treatment according to the recommendation of the commercially available RNeasy mini kit supplied by Qiagen. After RNA harvesting, cDNA synthesis was undertaken using the specified steps in Roche Applied Science's Titan one tube RT-PCR system.
A total of 30 cycles of RT-PCR were completed. In the RT-PCR protocol, denaturation step at 94°C for 1 min; annealing conducted for 1 min at temperatures between 52°C and 60°C (these temperature ranges were used according to genes) were performed for each gene. 2 min elongation was performed at 72°C, followed by a final 90 sec elongation at the same temperature.
Bcl-2 gene was amplified using Touchdown PCR, starting with an annealing temperature of 73°C, which was gradually decreased by 1°C per cycle for the first 10 cycles, and then held at 63°C for the remaining 25 repetitions. Water was used as the control group. All RT-PCR experiments were conducted in three replicates. Glyceraldehyde-3-phosphate dehydrogenase (GAPDH) was applied as the stable reference gene for expression normalization. A precise explanation of the genes and primers utilized in the current investigation can be established in Table I.
Table I: Sequence of utilized primers
Gene | F-Primer Sequence (5'-3') | R-Primer Sequence (5'-3') |
---|---|---|
PCNA | CGCGCAGAGGGTTGGTAGTT | AAGCCTTCGGAGCGCAGAGT |
Bcl-2 | TGCACCTGACGCCCT TCAC | AGACAGCCAGGAGAAATCAAACAG |
Bax | ACCAAGAAGCTGAGCGAGTGTC | ACAAAGATGGTCACGGTCTGCC |
GAPDH | TTCATTGACCTCAACTACAT | GAGGGGCCATCCACAGTCTT |
Cyclin D1 | CCG TCC ATG CGG AAG ATC | ATG GCC AGC GGG AAG AC |
Cyclin D2 | TACTTCAAGTGCGTGCAGAAGGAC | TCCCACACTTCCAGTTGCGATCAT |
Topoisomerase II-alpha | CAGATCATGGAAAATGCTGA | GCAGCATCATCTTCAGGACC |
Cyclin A | TGGAGAGTGTATGTTTGTTGTGGTG | AAACTTAACAATACAACTAAACTAC |
Cyclin B | GACATGGAAGATGAAGATCCTAA | TCAGCAGTCACCTCACCAATCT |
Cyclin E | CAGCCAAAAATGCGAGCAATTC | TTCATCTCCTGAACAAGCTCCATC |
All amplified DNA fragments were interpreted using 2.5% agar gel electrophoresis prepared using TBE buffer (containing ethidium bromide). The gel underwent electrophoresis for 30 min under a constant voltage of 120 V, utilizing a 100 bp DNA ladder used as molecular weight references. Band density was analyzed using Adobe Photoshop CS4. The intensity of PCR bands was evaluated by normalizing to the intensity of the housekeeping gene. All PCR samples were examined in relation to the control band intensities and the outcomes were given as percentage control (Bijwaard et al., 2001; Huang et al., 2003).
Statistical analysis
Replicate consequences were aggregated and displayed as mean ± SEM. Variance analysis (ANOVA) was accomplished for quantitative assessment. One-way ANOVA was conducted to assess if marked differences were identified between the central tendencies of three or more independent groups with respect to a certain variable. Tukey's test was employed for pairwise comparisons. Significant quantitative differences was recorded at p<0.05. Data analysis was performed with the aid of Minitab software, version 13.0.
Results
Influence on cell proliferation
Both A-549 and H1299 cells exhibited a concentration-dependent response to treatment with cetuximab and carvacrol, with changes in cell viability observed. After treating A-549 cells with different concentrations of cetuximab for 48 hours, IC10, IC20, IC30, IC40, and IC50 values were determined to be 174, 472, 770, 1067, and 1365 µg/mL, respectively. Similarly, for H1299 cells exposed to varying cetuximab concentrations for 48 hours, IC10, IC20, IC30, IC40, and IC50 values were determined to be 102, 369, 636, 904, and 1171 µg/mL, respectively (data not shown).
Upon treatment of A-549 cells with a range of concentrations of carvacrol across 48 hours timeframe, IC10, IC20, IC30, IC40, and IC50 values were found to be 11, 21, 31, 41, and 51 µg/mL, respectively. Similarly, for H1299 cells treated with varying concentrations of carvacrol for 48 hour, IC10, IC20, IC30, IC40, and IC50 values were determined to be 8 µg/mL, 17 µg/mL, 26 µg/mL, 35 µg/mL, and 49 µg/mL, respectively (data not shown).
This investigation was designed to determine whether lower concentrations of carvacrol, below its IC50 value, could enhance the cytotoxic effects of cetuximab (<IC50) in A-549 and H1299 cells. To identify the most effective cytotoxic response, combinations of cetuximab and carvacrol at concentrations below their IC₅₀ values were administered and evaluated (Figure 1A-B). For A-549 cells, the most effective combination was found to be IC10 cetuximab with IC10 carvacrol (Figure 1A). To evaluate the interaction between cetuximab and carvacrol, CI was computed, which indicated whether the dual treatment had combined, synergistic, or opposing outcomes. CI value of 2 confirmed the synergistic effect of the IC10 cetuximab and IC10 carvacrol combination in A-549 cells. In H1299 cells, the most effective combination was IC20 cetuximab with IC10 carvacrol (Figure 1B), and CI value of 2 again confirmed the synergistic effect of this combination. Statistical analysis showed that the impact of different cell line types and the influence of combined concentrations on cytotoxicity was significant (p<0.05). The cellular toxicity varied significantly between every cell line (p<0.05). Overall, the pairing of cetuximab and carvacrol enhanced the cytotoxic effects in both H1299 and A-549 cells relative to cetuximab intervention in isolation.
Figure 1: Cytotoxic effects of cetuximab (IC10, IC20, IC30, and IC40) combined with carvacrol at IC10, IC20, IC30 and IC40 were assessed over 48 hours period on A-549 (A) and H1299 (B) cells. Results are expressed as a viability ratio relative to the control group, which included untreated cells receiving only the medium. Values represent the mean of three independent trials, each comprising three replicates, ± SEM
Cell-killing effect
After 48 hours of exposure, changes in LDH activity were assessed in H1299 cells treated with cetuximab at IC20 concentration, both alone and in combination with IC10 carvacrol, which produced the strongest cytotoxic response. A significant elevation in LDH activity was observed in treated cells relative to the vehicle control group. Specifically, combination of cetuximab and carvacrol led to a 239% elevated in LDH activity in H1299 cells relative to the unexposed group, which consisted solely of growth medium excluding any treatment (Table II).
Table II: Effects of carvacrol with cetuximab on different parameters in two cell lines
Concentrations | LDH activity (mU/mL) |
Caspase-3 activity (µmol/mg protein) |
GPx activity (U/mg protein) |
---|---|---|---|
A-549 cell lines | |||
Cetuximab (IC10) | 144.7 ± 0.4a | 17.5 ± 0.3a | 2.2 ± 0.3a |
Cetuximab (IC10) + Carvacrol (IC10) | 233.3 ± 0.4a | 24.9 ± 0.3a | 2.5 ± 1.0a |
Control | 116.0 ± 0.1a | 15.2 ± 0.4a | 1.1 ± 0.3a |
H1299 cell lines | |||
Cetuximab (IC20) | 272.7 ± 0.7a | 17.0 ± 0.4a | 2.4 ± 0.5a |
Cetuximab (IC20) + Carvacrol (IC10) | 345.5 ± 0.7a | 21.0 ± 0.4a | 2.9 ± 0.2a |
Control | 102.0 ± 0.6a | 15.5 ± 0.2a | 1.8 ± 0.9a |
Data are mean ± SEM; ap means <0.05 (statistically significant) of carvacrol with/without cetuximab in comparison to control as determined by ANOVA with Tukey's test. LDH refers to lactate dehydrogenase, and GPx represents glutathione peroxidase. Each value is the mean of three repetitions for the activities of LDH, caspase-3, and GPx |
LDH enzyme activity was assessed following the treatment of A-549 cells with IC10 cetuximab alone and in combination with IC10 carvacrol, which were found to induce the strongest cytotoxic effects, over a 48 h period (Table II). Following this incubation, a notable increase in LDH release was observed, with levels rising by approximately 25% for cetuximab alone and by 101% for the combination treatment, in relation to the baseline (p<0.05). When evaluating the results in relation to the control groups, both A-549 and H1299 cells showed substantial discrepancy (p<0.05) in LDH enzymatic activity following treatment with cetuximab alone or the cetuximab-carvacrol combination. Additionally, the combination treatment led to a greater elevation in LDH levels than cetuximab alone in both cell lines, relative to their respective control groups.
Effects of cetuximab and carvacrol on oxidative stress
Following 48 hours of treatment with cetuximab alone or together with carvacrol, there was a significant increase in the antioxidant enzyme glutathione peroxidase (GPx) levels in both A-549 and H1299 cells (Table II). Cells treated with either cetuximab alone or the cetuximab-carvacrol combination showed a marked elevation in GPx and catalase activity (p<0.05). In particular, when H1299 were subjected to the combination treatment for 48 hours, GPx activity increased approximately multiplied by 1.6 in contrast to the baseline , which consisted solely of the cellular growth medium lacking the evaluated compounds. without the test compounds. This rise was statistically significant (p<0.05). The results suggest that both cetuximab alone and in combination with carvacrol induced oxidative stress in A-549 and H1299 cells, likely via the induction of reactive oxygen species.
Impact on caspase-3 enzyme activity
The ability of cetuximab, both alone and in combination with carvacrol, to trigger apoptosis was evaluated in A-549 and H1299 cells following 48 hours of treatment. In A-549 cells, caspase-3 activity increased by 1.6-fold following treatment with the combination of IC10 cetuximab and IC10 carvacrol. In H1299 cells, caspase-3 activity was elevated by approximately 1.4-fold after exposure to the combination of IC20 cetuximab and IC10 carvacrol, in relation to the control group (Table II). Apoptosis-inducing effect induced by cetuximab-carvacrol combination was found to be 1.2 times stronger in A-549 cells than in H1299 cells, with A-549 cells showing the most pronounced increase in caspase-3 activity following treatment.
All applications, including single and combined applications, have membrane damaging, oxidative stress inducing effects and apoptosis inducing potentials showed significant differences when compared to the baseline groups (which were administered only using the culturing solution and not exposed to any test compounds) in all cell lines (p<0.05).
Effects of on cell cycle and apoptotic mechanism
RT-PCR analysis was carried out using designated primers for every target gene, using glyceraldehyde 3-phosphate dehydrogenase (GAPDH) as an internal normalization control. Cellular proliferation marker PCNA is an essential protein that plays a vital function in DNA replication and repair mechanisms, functioning as a cofactor for DNA polymerase. It is vital for regulating the cell cycle, especially during the shift in the cell cycle from the G1 phase to the S phase. Due to its increased expression in proliferating cells, PCNA is commonly used as a marker for cell division. The expression of PCNA mRNA was analyzed to assess the antiproliferative influence of cetuximab by itself and in conjunction with carvacrol in A-549 and H1299 cells. The dual intervention was observed to be enhanced efficient in lowering levels of PCNA compared to cetuximab by itself in all cell types. The most notable decrease in PCNA expression levels was observed in H1299 human lung carcinoma cells, where expression reduced by 20% with cetuximab by itself and 44% with the dual therapy, when contrasted with the unexposed group (Figure 2B).
Figure 2: Effects of cetuximab, both alone and in combination with carvacrol, on mRNA expression of PCNA, topoisomerase II-alpha, cyclin D1, cyclin D2, cyclin E, cyclin A, cyclin B, Bax, and Bcl-2 were assessed in A-549 (A) and H1299 (B) cells. Data are mean ± SEM
Topoisomerase II-alpha is a critical enzyme that serves a crucial influence in DNA replication, transcription, and chromosome segregation. It modulates DNA topology by introducing or removing supercoils in the DNA structure, which is essential for processes such as cell division, where it helps untangle DNA strands. The most significant decrease in expression of topoisomerase II-alpha transcript was observed in human lung carcinoma A-549 cells following the two conditions cetuximab by itself and combination treatments. Cetuximab alone intervention led to 38% decrease in topoisomerase II-alpha gene transcript expression in A-549 cells compared to control, whereas the combination intervention led to more substantial 75% decrease (Figure 2A).
Cyclins D1 and D2 are key regulators of the cellular proliferation cycle, especially during first growth phase. The influence of cetuximab, alone and in combination with carvacrol, on the G1 phase transition of the cell cycle was evaluated by quantifying changes in cyclin-related RNA transcript levels. In adenocarcinomic human alveolar basal epithelial A-549 cells treated with cetuximab individually, cyclin D1 transcript level diminished by 30% as opposed to the experimental baseline group. On the other hand, at the time that cetuximab was combined with carvacrol, the most significant decrease in cyclin D1 was observed in H1299 cells, where expression dropped by 62%. For cyclin D2, the greatest reduction was seen in H1299 cells, with a 32% decrease following cetuximab alone, and a 49% reduction in A-549 cells after the combined treatment. Overall, the combination treatment was more effective than cetuximab alone in lowering the levels of both cyclin D1 and D2 in both cell lines (Figure 2A-B).
Cyclin E is a key protein involved in modulating the cell proliferation cycle, especially throughout the shift beginning with G1 step to the S step. In human lung adenocarcinoma cells (A-549), cetuximab administration in isolation led to a 35% reduction in cyclin E transcription level in contrast to the experimental baseline, whereas the combination treatment contributed to extended substantial 64% diminution in cyclin E transcription level. The most significant decrease in cyclin E levels was seen in A-549 cells for both treatments (Figure 2A). Furthermore, cetuximab in conjunction with carvacrol proved to be extended impactful as opposed to cetuximab by itself in lowering the transcription levels of cyclins D1, D2, and E-proteins that regulate different stages of the G1 phase. These findings suggest that the combined treatment has a more potent effect on the initial growth phase of the cell proliferation cycle than cetuximab alone.
In H1299 cells treated with cetuximab alone, cyclin A transcription level diminished by 13% as opposed to the baseline. In A-549, cetuximab alone resulted in 20% reduction in cyclin A expression relative to the control group (Figure 2A-B). The most notable decrease in cyclin A levels, however, was detected in A-549 cells following both cetuximab on its own and combination treatments.
In A-549 cells, cetuximab treatment on its own caused to 30% decrease in cyclin B transcription level as opposed to the baseline. However, in the event that cetuximab was combined with carvacrol, there was a more pronounced 60% decline in cyclin B transcription level in contrast to the control group (Figure 2A). It has been shown that the combination application is more effective in arresting second growth phase (G2) and mitotic (M) phases of the cell proliferation cycle in both cells than the application alone.
In both H1299 and A-549 cells, treatment with cetuximab alone and in combination with carvacrol resulted in a rise in Bax mRNA levels compared to control (Figure 2A-B). In H1299 cells, cetuximab alone induced 64% increase in Bax mRNA expression, while the combination treatment with cetuximab and carvacrol caused 88% increase (Figure 2B). Regarding Bcl-2, cetuximab alone led to 20% decrease in its mRNA expression in H1299 cells, whereas the combination treatment resulted in 34% reduction (Figure 2B). The combination therapy proved to be more effective than cetuximab on its own in lowering Bcl-2 expression in both cell lines. Consequently, both treatments boosted correlation between Bax and Bcl-2 in H1299 along with A-549 cells, with the combined treatment showing a stronger effect than cetuximab alone in enhancing this ratio.
Discussion
The findings of this study demonstrate that the combination of cetuximab and carvacrol exerts a more pronounced anticancer effect than cetuximab alone in lung cancer cell lines A-549 and H1299. MTT assays confirmed a concentration-dependent inhibition of cell proliferation, with the most effective combinations being IC₁₀ cetuximab + IC₁₀ carvacrol for A-549 and IC₂₀ cetuximab + IC₁₀ carvacrol for H1299 (CI = 2.0), indicating strong synergistic interactions. LDH assays showed significantly elevated enzyme release, particularly 101.0% in A-549 and 239.0% in H1299 (p<0.05), suggesting increased membrane damage. The combination therapy also significantly increased caspase-3 activity by 1.6-fold in A-549 and 1.4-fold in H1299 (p<0.05), highlighting enhanced apoptosis. RT-PCR analysis revealed downregulation of cell cycle regulatory genes including PCNA, topoisomerase II-α, and cyclins D1, D2, E, A, and B, with the combined treatment producing stronger effects (p<0.05). Additionally, the Bax/Bcl-2 ratio was markedly increased, further supporting apoptosis induction. These results suggest that carvacrol significantly enhances the cytotoxic, oxidative, and apoptotic effects of cetuximab.
Epidermal growth factor receptor (EGFR) is known to be overexpressed across various cancer types, Such as head and neck squamous cell carcinoma (Weichselbaum et al., 1989) (HNSCC), non-small cell lung cancer (Veale et al., 1987) (NSCLC), as well as cancers of the colon, breast (Lo et al., 2006), pancreas (Yasui et al., 1988), and brain (Libermann et al., 1984). Dysregulated EGFR signaling in these malignancies is associated with poor patient prognosis. Due to the close relationship between EGFR and cancer, treatments that target this structure are being developed and used today (Pearson et al., 2018). Cetuximab is a chimeric monoclonal antibody, combining both human and mouse components, designed to target EGFR. It attaches to the extracellular region of EGFR, facilitating receptor internalization and thereby reducing EGFR expression (Prewett et al., 1996). Cetuximab has been proven to constrain the enlargement of multiple cancerous human cell lines that exhibit increased EGFR expression (Matar et al., 2004; Huether et al., 2005). Received FDA approval in 2004 for the intervention of head and neck cancers (Galizia et al., 2007), cetuximab has also shown promise in treating non-small cell lung cancer (NSCLC) (Pirker et al., 2009; Douillard et al., 2014). However, its effectiveness as a monotherapy is limited, with response rates falling below 15% in HNSCC patients (Petrelli et al., 2014). Therefore, evaluating the antitumor impacts of cetuximab in combination therapies has become crucial. Enhancing its anticancer potential and expanding its clinical applications remain key challenges. Consequently, combination therapies are being explored to improve efficacy, reduce side effects, and overcome resistance, with natural products gaining increasing attention in these strategies.
Carvacrol, a potential anticancer agent, has shown effectiveness in suppressing cell growth, causing cell cycle blockage, and triggering programmed cell death in several cancerous human cell lines (Fatima et al., 2022; Mari et al., 2021; Chiu et al., 2023). These actions contribute to enhancing the susceptibility of transfor-med cells to chemotherapy along with targeted therapies. Additionally, combining carvacrol with other treatments may improve the therapeutic outcomes of both chemotherapeutic agents and targeted drugs. Based on existing studies, this investigation aimed to determine whether carvacrol could contribute to overcoming acquired resistance to cetuximab. How-ever, the impact of carvacrol on the reaction of NSCLC cells to cetuximab, as well as the mechanisms involved, remains poorly understood. This investigation exami-nes the therapeutic impacts and underlying dynamics of combining cetuximab with carvacrol in A-549 and H1299 NSCLC cell lines.
The findings indicate that the combination of carvacrol and cetuximab significantly reduced the viability and proliferation of A-549 and H1299 cells. This study demonstrates that using lower doses of cetuximab in combination with carvacrol enhances the antitumor effects in these cell lines, showing a synergistic impact compared to cetuximab alone. Detailed analysis revealed that the underlying mechanism of this effect involves disruption of the cell membrane, induction of programmed cell death, and cell cycle arrest in both A-549 and H1299 cells.
Studies have shown that cetuximab, when used in combination with various natural anticancer agents, can enhance apoptosis in human tumor cells. As an illustration, an exploration established that cetuximab blended with genistein, a soy-derived isoflavonoid, resulted in significant antitumor effects in mice with oral squamous cell carcinoma (OSCC) xenografts (Park et al., 2010). Similarly, when cetuximab was in combination with docetaxel, A taxane-based drug gathered from European yellow tree, it effectively suppressed the growth of non-small cell lung cancer PC9/G2 cells in both laboratory and animal models (Zhang et al., 2014). Additionally, the compound guggulsterone, in a natural manner found substance implemented in conventional Indian medicine, was shown to amplify the cell growth-blocking effects of cetuximab in squamous cell carcinoma of the head and neck (HNSCC) (Leeman-Neill et al., 2009).
The pairing of cetuximab and oridonin, an active compound derived from Rabdosia rubescens, has demonstrated combined antitumor effects against laryngeal squamous cell carcinoma (LSCC) in both laboratory and animal models. Notably, this combination resulted in enhanced anti-proliferative activity in vitro, significantly inhibiting p-EGFR expression. In vivo, the combination treatment led to a marked reduction in p-EGFR levels within tumor tissues and promoted tumor regression in HEp-2 xenografts, outperforming treatments with either cetuximab or oridonin alone. Throughout the study, no notable side effects were observed in the treatment groups. The results demonstrated that combining oridonin with cetuximab not only inhibited EGFR phosphorylation but also triggered considerable apoptosis in both laboratory and animal models. LSCC cells treated with both oridonin and Cetuximab treatment resulted in higher levels of Fas, FasL, and activated caspase-8 compared to such treated with oridonin by itself. In HEp-2 tumor-bearing mice, the combination therapy led to substantial apoptosis and a substantial enhancement in stimulated caspase-8 as well as caspase-3, indicating that Fas-mediated extrinsic apoptotic pathway is essential in the tumor cell death triggered by oridonin and cetuximab (Cao et al., 2016). In a separate study, the combination of honokiol, a natural substance extracted from the tree's outer layer and foliage of the Chinese magnolia tree, combined with cetuximab, was established to improve the susceptibility of KRASG13D mutant colorectal cancer (CRC) cells in laboratory as well as animal models. Honokiol was found to interfere with SNX3-retromer-dependent vesicular transport, resulting in disrupted proteolytic activity in lysosomes and suppression of autophagy and macropinocytosis. Honokiol was shown to disrupt SNX3-retromer-mediated trafficking, leading to impaired lysosomal proteolytic function and inhibition of autophagy and macropinocytosis. Additionally, honokiol blocked the activation of RAS through GDP to GTP exchange, which further enhanced responsiveness of KRASG13D mutant CRC cells response to cetuximab treatment. This combination therapy improved cetuximab efficacy by targeting the SNX3 retromer in a preclinical model of KRASG13D mutant colorectal cancer. These results offer a promising approach for enhancing targeted therapy in individuals with KRAS mutant colorectal cancer (Zhu et al., 2024). In another study, the findings indicate that honokiol could be a potent natural compound for defeating resistance developed to cetuximab. When honokiol was combined with cetuximab, significant reductions were observed in the quantities of pHER2, pHER3, pMAPK, pAKT, and pC-RAF (Pearson et al., 2018), suggesting a potential mechanism for improving treatment outcomes in resistant cancer cases.
It is believed that an increase in reactive oxygen species (ROS) within cells could trigger apoptosis through elevated oxidative stress (Pan et al., 2014). On the other hand, cancers, such as those associated with tobacco use and driven by ROS, often show heightened MAPK activation. This includes oral and lung cancers, as well as cancers linked to viruses like Epstein-Barr virus and hepatitis C. In these cancers, ROS play a key role in regulatory signaling networks that may play a role in tumor persistence and progression (Bonner and Arbiser, 2014). One of the findings from the study showed that combining honokiol with cetuximab led to a reduction in ROS production (Pearson et al., 2018). This decrease in ROS was associated with lower cell proliferation and survival, a halt in the initial growth phase (G1) of the cell proliferation cycle, and delayed tumor growth in patient-derived xenograft models from cetuximab-refractory HNSCC (Pearson et al., 2018).
Another study demonstrated a synergistic impact when 125 μg/mL of β-elemene was used in conjuction with 25 μg/mL of cetuximab in colorectal cancer (CRC) cells with KRAS mutations. In vitro, this combination induced the accumulation of iron-mediated reactive oxygen species (ROS), depletion of glutathione (GSH), increased lipid degradation, and enhanced expression of HO-1 and transferrin. The combination of β-elemene and cetuximab also led to the downregulation of key proteins associated with ferroptosis, such as GPX4, SLC7A11, FTH1, glutaminase, and SLC40A1, in CRC cells harboring KRAS mutations. This approach also suppressed cell displacement and reduced the manifestation of mesenchymal markers, including Vimentin, N-cadherin, Slug, Snail, and MMP-9, while promoting the manifestation of epithelial marker E-cadherin. In animal models, the pairing of β-elemene and cetuximab significantly suppressed tumor progression and lymphatic tissues metastasis in KRAS mutant models (Chen et al., 2020). A study examined the impact of combining withaferin-A, a steroidal lactone originating from the Indian Ayurvedic herb Withania somnifera (widely referred to as Ashwagandha), with 5-fluorouracil in colorectal cancer (CRC) cells. The results showed that this combination induced both autophagy and apoptosis through the activation of endoplasmic reticulum stress, leading to a reduction in cell proliferation potential and enhancing The pharmaceutical's effectiveness opposed to CRC. Furthermore, the pairing therapy reduced overactivation of critical proteins in the Wnt/β-catenin signaling pathway by removing phosphate groups from β-catenin and GSK3β at Ser675 and Ser9, respectively, ultimately causing cellular division process arrest during the G2/M phase (Alnuqaydan et al., 2020).
This study has several limitations. Reliance on A-549 and H1299 cell lines may not capture the heterogeneity of human lung tumors, limiting generalizability. The lack of in vivo validation leaves efficacy, safety, and pharmacokinetics unassessed. MTT and LDH assays, used to assess viability and cytotoxicity, are prone to inaccuracies due to metabolic interference and serum-derived LDH, respectively. Moreover, the molecular mechanisms underlying observed biomarker changes remain unexplored. Finally, the 48-hour treatment period may not reflect long-term effects or resistance mechanisms, which are critical for evaluating therapeutic sustainability and informing future treatment strategies.
Conclusion
Combining cetuximab with carvacrol at low concentrations effectively inhibits lung cancer cell proliferation by inducing apoptosis, oxidative stress, and cell cycle arrest, highlighting its potential as a synergistic and less toxic therapeutic strategy.
Ethical Issue
Cell lines derived from the expansion of primary cell cultures in vitro were not relevant material, as all the original cells were divided and so the cell line had been created outside of the human body. The storage and use of cell lines created from primary human tissue, for research purposes, did not require ethical approval.
References
Alar T, Sahin EM. Akciger kanseri: Birinci basamakta tanı, tedavi ve korunma. Smyrna Tıp Dergisi. 2012; 2: 68-74.
Alma MH, Mavi A, Yildirim A, Digrak M, Hirata T. Screening chemical composition and in vitro antioxidant and antimicrobial activities of the essential oils from Origanum syriacum L. growing in Turkey. Biol Pharm Bull. 2003; 26: 1725-29.
Alnuqaydan AM, Rah B, Almutary AG, Chauhan SS. Syner-gistic antitumor effect of 5-fluorouracil and withaferin-A induces endoplasmic reticulum stress-mediated autophagy and apoptosis in colorectal cancer cells. Am J Cancer Res. 2020; 10: 799-815.
Arkali G, Aksakal M, Kaya SO. Protective effects of carvacrol against diabetes‐induced reproductive damage in male rats: Modulation of Nrf2/HO‐1 signaling pathway and inhibition of Nf‐kB‐mediated testicular apoptosis and inflammation. Andrologia 2021; 53: e13899.
Arunasree KM. Antiproliferative effects of carvacrol on a human metastatic breast cancer cell line, MDA-MB 231. Phytomedicine 2010; 17: 581-88.
Baselga J. The EGFR as a target for anticancer therapy-focus on cetuximab. Eur J Cancer. 2001; 37: 16-22.
Baykara O. Kanser tedavisinde guncel yaklaşımlar. Balıkesir Saglık Bilimleri Dergisi. 2016; 5: 154-65
Bijwaard KE, Aguilera NS, Monczak Y, Trudel M, Taubenberger JK, Lichy JH. Quantitative real-time reverse transcription-PCR assay for cyclin D1 expression: Utility in the diagnosis of mantle cell lymphoma. Clin Chem. 2001; 47: 195-201.
Boduroglu B, Investigation of the effect of carvacrol against arsenic induced oxidatıve stress in rats. Master's thesis, Afyon Kocatepe University. Gazlıgol Yolu, 2022, pp 1-64.
Bonner MY, Arbiser JL. The antioxidant paradox: what are antioxidants and how should they be used in a therapeutic context for cancer. Future Med Chem. 2014; 6: 1413-22.
Bradford MM. A rapid and sensitive method for the quantitation of microgram quantities of protein utilizing the principle of protein-dye binding. Anal Biochem. 1976; 72: 248-54.
Bruns, C.J, Harbison MT, Davis DW, Portera CA, Tsan R, McConkey DJ., Evans DB, Abbruzzese JL, Hicklin DJ, Radinsky R. Epidermal growth factor receptor blockade with C225 plus gemcitabine results in regression of human pancreatic carcinoma growing orthotopically in nude mice by antiangiogenic mechanisms. Clin Cancer Res. 2000; 6: 1936-48.
Buchsbaum DJ, Bonner JA, Grizzle WE, Stackhouse MA, Carpenter M, Hicklin DJ, Bohlen P, Raisch KP. Treatment of pancreatic cancer xenografts with erbitux (IMC-C225) anti-EGFR antibody, gemcitabine, and radiation. Int J Radiat Oncol Biol Phys. 2002; 54: 1180-93.
Cao S, Xia M, Mao Y, Zhang Q, Donkor PO, Qiu F, Kang N. Combined oridonin with cetuximab treatment shows synergistic anticancer effects on laryngeal squamous cell carcinoma: Involvement of inhibition of EGFR and activation of reactive oxygen species-mediated JNK pathway. Int J Oncol. 2016; 49: 2075-87.
Chami N, Chami F, Bennis S, Trouillas J, Remmal A. Antifungal treatment with carvacrol and eugenol of oral candidiasis in immunosuppressed rats. Brazil J Infect Dis. 2004; 8: 217-26.
Chen P, Li X, Zhang R, Liu S, Xiang Y, Zhang M, Chen X, Pan T, Yan L, Feng J, Duan T, Wang D, Chen B, Jin T, Wang W, Chen L, Huang X, Zhang W, Sun Y, Li G, Kong L, Chen X, Li Y, Yang Z, Zhang Q, Zhuo L, Sui X, Xie T. Combinative treatment of β-elemene and cetuximab is sensitive to KRAS mutant colorectal cancer cells by inducing ferroptosis and inhibiting epithelial-mesenchymal transformation. Theranostics 2020; 10: 5107-19.
Cheng YD, Yang H, Chen GQ, Zhang ZC. Molecularly targeted drugs for metastatic colorectal cancer. Drug Des Devel Ther. 2013; 7: 1315-22.
Chiu KW, Chen HY, Chen CL, Hsieh CP, Huang YF. Attenuation of endoplasmic reticulum stress enhances carvacrol-induced apoptosis in osteosarcoma cell lines. Life 2023; 13: 744-.
Douillard JY, Pirker R, O'Byrne KJ, Kerr KM, Storkel S, von Heydebreck A, Grote HJ, Celik I, Shepherd FA. Relationship between EGFR expression, EGFR mutation status, and the efficacy of chemotherapy plus cetuximab in FLEX study patients with advanced non-small-cell lung cancer. J Thorac Oncol. 2014; 9: 717-24.
Fatima K, Luqman S, Meena A, Carvacrol arrests the proliferation of hypopharyngeal carcinoma cells by suppressing ornithine decarboxylase and hyaluronidase activities. Front Nutr. 2022; 9: 857256.
Flohe L, Gunzler WA. Assays of glutathione peroxidase. Methods Enzymol. 1984; 105: 114-20.
Galizia G, Lieto E, De Vita F, Orditura M, Castellano P, Troiani T, Imperatore V, Ciardiello F. Cetuximab, a chimeric human mouse anti-epidermal growth factor receptor monoclonal antibody, in the treatment of human colorectal cancer. Oncogene. 2007; 26: 3654-60.
Gill GN, Kawamoto T, Cochet C, Le A, Sato JD, Masui H, McLeod C, Mendelsohn J. Monoclonal anti-epidermal growth factor receptor antibodies which are inhibitors of epidermal growth factor binding and antagonists of epidermal growth factor-stimulated tyrosine protein kinase activity. J Biol Chem. 1984; 259: 7755-60.
Hashemipour H, Kermanshahi H, Golian A, Veldkamp T. Metabolism and nutrition: Effect of thymol and carvacrol feed supplementation on performance, antioxidant enzyme activities, fatty acid composition, digestive enzyme activities, and immune response in broiler chickens. Poult Sci. 2013; 92: 2059-69.
Herbst RS, Shin DM. Monoclonal antibodies to target epidermal growth factor receptor–positive tumors. Cancer 2002; 94: 1593-611.
Huang F, Wu XN, Chen JIE, Wang WX, Lu ZF. Resveratrol reverses multidrug resistance in human breast cancer doxorubicin-resistant cells. Exp Ther Med. 2014; 7: 1611-16.
Huang ST, Yang RC, Yang LJ, Lee PN, Pang JHS. Phyllanthus urinaria triggers the apoptosis and Bcl-2 down-regulation in Lewis lung carcinoma cells. Life Sci. 2003; 72: 1705-16.
Huether A, Hopfner M, Baradari V, Schuppan D, Scherubl H. EGFR blockade by cetuximab alone or as combination therapy for growth control of hepatocellular cancer. Biochem Pharmacol. 2005; 70: 1568-78.
Jayakumar S, Madankumar A, Asokkumar S, Raghunandhakumar S, Gokula dhas K, Kamaraj S, Divya MG, Devaki T. Potential preventive effect of carvacrol against diethylnitrosamine-induced hepatocellular carcinoma in rats. Mol Cell Biochem. 2012; 360: 51-60.
Lee B, Yeom M, Shim I, Lee H, Hahm D. Inhibitory effect of carvacrol on lipopolysaccharide-induced memory impairment in rats. Korean J Physiol Pharmacol. 2020; 24: 27-37.
Leeman-Neill RJ, Wheeler SE, Singh SV, Thomas SM, Seethala RR, Neill DB, Panahandeh MC, Hahm ER, Joyce SC, Sen M, Cai Q, Freilino ML, Li C, Johnson DE, Grandis JR. Guggulsterone enhances head and neck cancer therapies via inhibition of signal transducer and activator of transcription-3. Carcinogenesis 2009; 30: 1848-56.
Libermann TA, Razon N, Bartal AD, Yarden Y, Schlessinger J, Soreq H. Expression of epidermal growth factor receptors in human brain tumors. Cancer Res. 1984; 44: 753-60.
Lo HW, Hsu SC, Hung MC. EGFR signaling pathway in breast cancers: from traditional signal transduction to direct nuclear translocalization. Breast Cancer Res Treat. 2006; 95: 211-18.
Kohrt HE, Colevas AD, Houot R, Weiskopf K, Goldstein MJ, Lund P, Mueller A, Sagiv-Barfi I, Marabelle A, Lira R, Troutner E, Richards L, Rajapaska A, Hebb J, Chester C, Waller E, Ostashko A, Weng WK, Chen L, Czerwinski D, Fu YX, Sunwoo J, Levy R. Targeting CD137 enhances the efficacy of cetuximab. J Clin Invest. 2014; 124: 2668-82.
Mari A, Mani G, Nagabhishek SN, Balaraman G, Subramanian N, Mirza FB, Sundaram J, Thiruvengadam D. Carvacrol promotes cell cycle arrest and apoptosis through PI3K/AKT signaling pathway in MCF-7 breast cancer cells. Chin J Integr Med. 2021; 27: 680-87.
Matar P, Rojo F, Cassia R, Moreno-Bueno G, Di Cosimo S, Tabernero J, Guzmán M, Rodriguez S, Arribas J, Palacios J, Baselga J. Combined epidermal growth factor receptor targeting with the tyrosine kinase inhibitor gefitinib (ZD1839) and the monoclonal antibody cetuximab (IMC-C225): Superiority over single-agent receptor targeting. Clin Cancer Res. 2004; 10: 6487-501.
Melo JO, Fachin AL, Rizo WF, Jesus HC, Arrigoni-Blank MF, Alves PB, Marins MA, França SC, Blank AF. Cytotoxic effects of essential oils from three Lippia gracilis Schauer genotypes on HeLa, B16, and MCF-7 cells and normal human fibroblasts. Genet Mol Res. 2014; 13: 2691-97.
Mosmann T. Rapid colorimetric assay for cellular growth and survival: application to proliferation and cytotoxicity assays. J Immunol Methods. 1983; 65: 55-63.
Njume C, Afolayan AJ, Ndip RN. An overview of antimicrobial resistance and the future of medicinal plants in the treatment of Helicobacter pylori infections. Afr J Pharm Pharmacol. 2009; 3: 685-99.
Overholser JP, Prewett MC, Hooper AT, Waksal HW, Hicklin DJ. Epidermal growth factor receptor blockade by antibody IMC-C225 inhibits growth of a human pancreatic carcinoma xenograft in nude mice. Cancer 2000; 89: 74-82.
Pan J, Zhang Q, Liu Q, Komas SM, Kalyanaraman B, Lubet RA, Wang Y, You M. Honokiol inhibits lung tumorigenesis through inhibition of mitochondrial function. Cancer Prev Res (Phila). 2014; 7: 1149-59.
Park SJ, Kim MJ, Kim YK, Kim SM, Park JY, Myoung H. Combined cetuximab and genistein treatment shows additive anti-cancer effect on oral squamous cell carcinoma. Cancer Lett. 2010; 292: 54-63.
Pearson HE, Iida M, Orbuch RA, McDaniel NK, Nickel KP, Kimple RJ, Arbiser JL, Wheeler DL. Overcoming resistance to cetuximab with honokiol, A small-molecule polyphenol. Mol Cancer Ther. 2018; 17: 204-14.
Petrelli F, Coinu A, Riboldi V, Borgonovo K, Ghilardi M, Cabiddu M, Lonati V, Sarti E, Barni S. Concomitant platinum-based chemotherapy or cetuximab with radiotherapy for locally advanced head and neck cancer: A systematic review and meta-analysis of published studies. Oral Oncol. 2014; 50: 1041-48.
Pilau MR, Alves SH, Weiblen R, Arenhart S, Cueto AP, Lovato LT. Antiviral activity of the Lippia graveolens (Mexican oregano) essential oil and its main compound carvacrol against human and animal viruses. Braz J Microbiol. 2011; 42: 1616-24.
Pirker R, Pereira JR, Szczesna A, von Pawel J, Krzakowski M, Ramlau R, Vynnychenko I, Park K, Yu CT, Ganul V, Roh JK, Bajetta E, O'Byrne K, de Marinis F, Eberhardt W, Goddemeier T, Emig M, Gatzemeier U; FLEX Study Team. Cetuximab plus chemotherapy in patients with advanced non-small-cell lung cancer (FLEX): An open-label randomised phase III trial. Lancet 2009; 373: 1525-31.
Prewett M, Rockwell P, Rockwell RF, Giorgio NA, Mendelsohn J, Scher HI, Goldstein NI. The biologic effects of C225, a chimeric monoclonal antibody to the EGFR, on human prostate carcinoma. J Immunother Emphasis Tumor Immunol. 1996; 19: 419-27.
Radonic A, Milos M, Chemical composition and in vitro evaluation of antioxidant effect of free volatile compounds from Satureja montana L. Free Radic Res. 2003; 37: 673-79.
Rattanachaikunsopon P, Phumkhachorn P. Assessment of factors influencing antimicrobial activity of carvacrol and cymene against Vibrio cholerae in food. J Biosci Bioeng. 2010; 110: 614-19.
Ravishankar S, Zhu L, Reyna-Granados J, Law B, Joens L, Friedman M. Carvacrol and cinnamaldehyde inactivate antibiotic-resistant Salmonella enterica in buffer and on celery and oysters. J Food Prot. 2010; 73: 234-40.
Rivas L, McDonnell MJ, Burgess CM, O'Brien M, Navarro-Villa A, Fanning S, Duffy G. Inhibition of verocytotoxigenic Escherichia coli in model broth and rumen systems by carvacrol and thymol. Int J Food Microbiol. 2010; 139: 70-78.
Subak M, Aksel N, Kılıç Ozturk Y. Incidence of anemia in cases with lung cancer at the time of diagnosis and relation between cancer types. Forbes J Med. 2020; 1: 11-14.
Ultee A, Kets EPW, Smid EJ. Mechanisms of action of carvacrol on the food-borne pathogen Bacillus cereus. Appl Environ Microbiol. 1999; 65: 4606-10.
Veale D, Ashcroft T, Marsh C, Gibson GJ, Harris AL. Epidermal growth factor receptors in non-small cell lung cancer. Br J Cancer. 1987; 55: 513-16.
Weichselbaum RR, Dunphy EJ, Beckett MA, Tybor AG, Moran WJ, Goldman ME, Vokes EE, Panje WR. Epidermal growth factor receptor gene amplification and expression in head and neck cancer cell lines. Head Neck. 1989; 11: 437-42.
Wu X, Fan Z, Masui H, Rosen N, Mendelsohn J. Apoptosis induced by an antiepidermal growth factor receptor monoclonal antibody in a human colorectal carcinoma cell line and its delay by insulin. J Clin Invest. 1995; 95: 1897-905.
Yaman S, Ozdemir Z, Sit M, Ozer B, Catal O. Kekik yagı karvakrolun insan saglıgına etkileri. SETSCI Conference Indexing System. 2018; 2: 391-92.
Yasui W, Sumiyoshi H, Hata J, Kameda T, Ochiai A, Ito H, Tahara E. Expression of epidermal growth factor receptor in human gastric and colonic carcinomas. Cancer Res. 1988; 48: 137-41.
Zamani AG, Zamani A. MikroRNA’lar ve akciger kanseri. Tuberk Toraks. 2013; 61: 57-62.
Zhang L, Li XF, Tand L, Zhao YM, Zhou CC. The effects of cetuximab in combination with docetaxel for the acquired resistance to EGFR-TKI in non-small cell lung cancer cells. Tumor. 2014; 34: 584-90.
Zhu Q, Zhang R, Gu X, Zhao Z, Gao Q, Chen M, Wu Q, Xie T, Sui X. Honokiol enhances the sensitivity of cetuximab in KRASG13D mutant colorectal cancer through destroying SNX3-retromer complex. Theranostics 2024; 14: 5443-60.
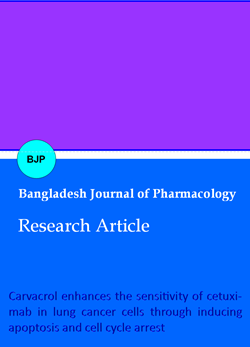