In vitro study on the hypoglycemic potential of Nicotiana tabacum leaf extracts
Abstract
Inhibition of some carbohydrate metabolizing enzymes is one of the modes of action of antihyperglycemic agents. The aim of this study was to investigate the in vitro inhibition of alpha-amylase and alpha-glucosidase by extracts of N. tabacum leaf. Powdered leaves were extracted with acetone, ethanol and water, and tested for their ability to inhibit alpha-amylase from Aspergilus oryzae and alpha-glucosidase from Saccharomyces cerevisae. The results revealed that aqueous extract of the plant was most effective inhibitor of alpha-amylase (IC50 5.7 mg/mL) while acetone extract exhibited the best inhibitory potential on alpha-glucosidase (IC50 4.5 mg/mL). Kinetic studies showed that the mode of inhibition of α-amylase by aqueous extract was non-competitive while that of the acetone extract on alpha-glucosidase was competitive. The observed inhibitions of alpha-amylase and alpha-glucosidase suggest that the leaf extracts of N. tabacum may be useful in the management of Diabetes mellitus, which may due to the presence of phytochemicals.
Introduction
Hyperglycemia is a condition characterized by a rapid increase in blood glucose levels (Deshpande et al., 2009). Postprandial hyperglycemia has been linked to the onset of diabetic complications in type-2 diabetic patients due to the generation of free radicals leading to damage in the retina, renal glomerulus and peripheral nerves (Brownlee, 2005; Kwon et al., 2005a). The enzymes that are implicated in this pathological condition include pancreatic alpha-amylase and intestinal alpha-glucosidase. alpha-Amylases hydrolyze complex polysaccharides to produce oligosaccharides and disaccharides which are then hydrolyzed by alpha-glucosidase to monosaccharides and are then absorbed through the small intestines into the hepatic portal vein (Smith et al., 2005).
One of the therapeutic approaches for decreasing postprandial hyperglycemia is to retard the digestion of glucose by the inhibition of these carbohydrate hydrolyzing enzymes, alpha-glucosidases, in the digestive tract (Deshpande et al., 2009). Therefore, inhibition of these carbohydrate-hydrolyzing enzymes can significantly decrease the postprandial hyperglycemia after a mixed carbohydrate diet and can be a key strategy in the control of diabetes mellitus (Hirsh et al., 1997). Though there are synthetic drugs used in the treatment of hyperglycemia such as acarbose and miglitol but these drugs are associated with gastrointestinal side effects including abdominal discomfort, bloating, flatulence and diarrhoea (Kwon et al., 2008a) which are allegedly caused by the excessive inhibition of pancreatic alpha-amylase (Horii et al., 1987).
However, plant derived alpha-amylase and alpha-glucosidase inhibitors are reported to have lower inhibitory effects against alpha-amylase activity and stronger inhibitory activity against alpha-glucosidase (Kwon et al., 2008b). This is an indication that plant extracts and their constituents may be effective therapeutic agents for the management of postprandial hyperglycemia with lesser side effects than the synthetic drugs. Several plants have been used in the management of diabetes in traditional medicine and one of such plant is Nicotiana tabacum.
tabacum commonly known as tobacco is a perennial herbaceous plant belonging to the family Solanaceae. N. tabacum is a native of tropical and subtropical America but it is now commercially cultivated worldwide. Its leaves are commercially grown in many countries to be processed as tobacco (Ren and Timko, 2001). Ethnomedicinally, a decoction of the leaves of this plant is believed to have antispasmodic, diuretic, emetic, expectorant, sedative, anaesthetic and antimicrobial property (Ezeja and Omeh, 2010; Bakht et al., 2012). Nicotine, which is the major component of tobacco, has been demonstrated to accelerate angiogenesis and wound healing in genetically diabetic mice (Jacobi et al., 2002).
Despite the wide folkloric use of this plant in the management of several diseases and ailments, coupled with various pharmacological reports of N. tabacum leaf, there is dearth of information on its inhibitory effects on key enzymes linked to diabetes. Therefore, this study sought to evaluate the inhibitory effect of N. tabacum leaf extracts on α-amylase and α-glucosidase as a possible means of managing diabetes mellitus induced hyperglycemia.
Materials and Methods
Sample collection: Fresh leaves of N. tabacum were collected from Ikoga-Zebbe, a community in Badagry Local Government Area of Lagos State, Nigeria in February, 2012. The plant was identified and authenticated by Dr. A. B. Kadiri of the Department of Botany, University of Lagos, Nigeria with a voucher number LUH 4718.
Chemicals and reagents: alpha-Amylase from Aspergillus oryzae, alpha-glucosidase from Saccharomyces cerevisiae and paranitrophenyl-glucopyranoside were products of Sigma-Adrich Co., St Louis, USA while starch soluble (extra pure) was obtained from J. T. Baker Inc., Phillipsburg, USA. Other chemicals and reagents were of analytical grade and water used was glass-distilled.
Preparation of plant extracts: Fresh leaves of N. tabacum were cut and washed with water to remove all contaminants; they were dried under room temperature and grounded to powder. The powdered leaves were divided into three portions and each portion was extracted with acetone, ethanol or water. They were all left to steep in covered containers for 24 hours; the resulting infusions were decanted, filtered and evaporated in a rotary evaporator (Cole Parmer SB 1100, Shangai, China). The extracts were freeze-dried using Virtis Bench Top (SP Scientific Series, USA) freeze dryer. Dried extracts were weighed and dissolved in 10% dimethylsulphoxide to yield a stock solution from which lower concentrations were prepared.
Phytochemical screening: Phytochemical compositions of the leaves were determined using the methods variously described elsewhere (Trease and Evans, 1996; Sofowara, 2006).
Test for tannins: In the test for tannins, 0.5 g of dried powdered sample was boiled in 20 mL of water in a test tube and filtered. Few drops of 0.1% ferric chloride was added and observed for brownish green or a blue black colouration as indication of tannins.
Test for saponin: Approximately 2 g of powdered material was boiled in 20 mL of distilled water in a water bath and filtered. Next, 10 mL of the filtrate was mixed with 5 mL of distilled water and shaken vigorously and observed for a stable persistent froth. The frothing was mixed with 3 drops of olive oil and shaken vigorously again and then observed for the formation of emulsion as indication of saponin.
Test for flavonoids: A portion of the powdered material was heated with 10 mL of ethyl acetate over a steam bath for 3 min. The mixture was filtered and 4 mL of the filtrate was shaken with 1 mL of dilute ammonia solution. Development of yellow coloration is an indication of the presence of flavonoids.
Test for steroids: In this test, 2 mL of acetic anhydride was added to 0.5 g of extract with 2 mL concentrated H2SO4. The color change from violet to blue or green is an indication of steroids.
Test for terpenoids: In brief, 5 mL of extract was mixed with 2 mL chloroform and 3 mL H2SO4 was carefully added to form a layer. A reddish brown coloration at the interface was an indication of terpenoids.
Test for anthraquinones: 5 mL of chloroform was added to 0.5 g of the powdered plant materials of each specimen. The resulting mixture was shaken for 5 min after which it was filtered. The filtrate was then shaken with equal volume of 10% ammonia solution. The presence of a bright pink color in the aqueous layer indicated the presence of anthraquinones.
Test for reducing sugar: To about 1 g of each sample in the test tube was added 10 mL distilled water and the mixture boiled for 5 min. The mixture was filtered while hot and the cooled filtrate made alkaline to litmus paper with 20% sodium hydroxide solution. The resulting solution was boiled with an equal volume of Benedict qualitative solution on a water bath. The formation of a brick red precipitate depicted the presence of reducing compound.
alpha-Amylase inhibitory assay: This assay was carried out using a modified procedure of McCue and Shetty (2004). A total of 250 µL of extract was placed in a tube and 250 µL of 0.02 M sodium phosphate buffer (pH 6.9) containing alpha-amylase solution was added. This solution was pre-incubated at 25°C for 10 min, after which 250 µL of 1% starch solution in 0.02 M sodium phosphate buffer (pH 6.9) was added at timed intervals and then further incubated at 25°C for 10 min. The reaction was terminated by adding 500 µL of dinitrosalicylic acid (DNS) reagent. The tubes were then incubated in boiling water for 5 min and cooled to room temperature. The reaction mixture was diluted with 5 mL distilled water and the absorbance was measured at 540 nm using a spectrophotometer (Spectrumlab S23A, Globe Medical England). A control was prepared using the same procedure replacing the extract with distilled water. The α-amylase inhibitory activity was calculated as percentage inhibition.
% Inhibition = [(Abscontrol - Absextracts)/Abscontrol] x 100
Concentrations of extracts resulting in 50% inhibition of enzyme activity (IC50) were determined graphically.
Mode of alpha-amylase inhibition: The mode of inhibition of the alpha-amylase by the leaf extracts was conducted using the extract with the lowest IC50 according to the modified method described by Ali et al. (2006). Briefly, 250 uL of the (5 mg/mL) extract was pre-incubated with 250 uL of alpha-amylase solution for 10 min at 25ºC in one set of tubes. In another set of tubes α-amylase was pre-incubated with 250 uL of phosphate buffer (pH 6.9). 250 µL of starch solution at increasing concentrations (0.3-5.0 mg/mL) was added to both sets of reaction mixtures to start the reaction. The mixture was then incubated for 10 min at 25°C, and then boiled for 5 min after addition of 500 µL of DNS to stop the reaction. The amount of reducing sugars released was determined spectrophotometrically using a maltose standard curve and converted to reaction velocities. A double reciprocal plot (1/v versus 1/[S]) where v is reaction velocity and [S] is substrate concentration was plotted. The mode of inhibition of α-amylase activity by the crude extract was determined by analysis of the double reciprocal (Lineweaver-Burk) plot using Michaelis-Menten kinetics.
alpha-Glucosidase inhibitory assay: The effect of the plant extracts on alpha-glucosidase activity was determined according to the method described by Kim et al. (2005), using alpha-glucosidase from Saccharomyces cerevisiae. The substrate solution p-nitropheynyl glucopyranoside (pNPG) was prepared in 20 mM phosphate buffer, pH 6.9. 100 µL of alpha-glucosidase (E.C. 3.2.1.20) was pre-incubated with 50 µL of the different concentrations of the extracts (acetone, ethanol and water) for 10 min. Then 50 µL of 3.0 mM (pNPG) as a substrate dissolved in 20 mM phosphate buffer (pH 6.9) was added to start the reaction. The reaction mixture was incubated at 37°C for 20 min and stopped by adding 2 mL of 0.1 M Na2CO3. The alpha-glucosidase activity was determined by measuring the yellow colored para-nitrophenol released from pNPG at 405 nm. The results were expressed as percentage of the blank control.
Percentage inhibition calculated as
% Inhibition = [(Abscontrol - Absextract)/Abscontrol] x 100
Concentrations of extracts resulting in 50% inhibition of enzyme activity (IC50) were determined graphically.
Mode of alpha-glucosidase inhibition: The mode of inhibition of α-glucosidase by the extracts was determined using the extract with the lowest IC50 according to the modified method described by Ali et al. (2006). Briefly, 50 uL of the (5 mg/mL) extract was pre-incubated with 100 uL of alpha-glucosidase solution for 10 min at 25°C in one set of tubes. In another set of tubes alpha-glucosidase was pre-incubated with 50 uL of phosphate buffer (pH 6.9). 50 µL of PNPG at increasing concentrations (0.6-2.0 mg/mL) was added to both sets of reaction mixtures to start the reaction. The mixture was then incubated for 10 min at 25°C, and 500 µL of Na2CO3 was added to stop the reaction. The amount of reducing sugars released was determined spectrophotometrically using a paranitrophenol standard curve and converted to reaction velocities. A double reciprocal plot (1/v versus 1/[S]) where v is reaction velocity and [S] is substrate concentration was plotted. The mode of inhibition of alpha-glucosidase activity by the crude extract was determined by analysis of the double reciprocal (Lineweaver-Burk) plot using Michaelis-Menten kinetics.
Statistical analysis: Statistical analysis was performed using GraphPad Prism 5 statistical package (GraphPad Software, USA). The data were analyzed by one-way analysis of variance (ANOVA) followed by Bonferroni test. All the results were expressed as mean ± SE for triplicate determinations.
Results
Table I shows the %yield of the acetone, ethanolic and aqueous extract of N. tabacum leaves. It was observed that the acetone extract has the highest percentage yield (18.6%) when compared to other extracts. A screening of the phytochemical composition as presented in Table II showed the presence of reducing sugars in all the extracts while none of the extracts possess anthraquinones and steroids. Flavonoids and tannins were detected in both acetone and aqueous extract only while saponins were only found in aqueous extract.
Table I: The percentage yield of different extracts of N. tabacum leaf
Extracts | Initial weight (g) | Final weight (g) | % yield |
---|---|---|---|
Acetone | 32.9 | 6.1 | 18.6 |
Ethanol | 33.6 | 3.3 | 9.9 |
Water | 38.7 | 2.3 | 6.0 |
Table II: The phytochemical constituent of different extracts of N. tabacum leaf
Phytochemicals | Inference | ||
---|---|---|---|
Acetone | Ethanol | Water | |
Anthraquinones | - | - | - |
Flavonoids | + | - | + |
Reducing sugar | + | + | + |
Saponins | - | - | + |
Steroids | - | - | - |
Tannins | + | - | + |
Terpenoids | - | + | - |
+ signifies presence, - signifies not detected |
Figure 1 showed the result of alpha-amylase inhibitory activities of the acetone, ethanolic and aqueous extracts of the plant investigated. At concentrations of 1.3 and 2.5 mg/mL, there were no significant differences (p>0.05) among the three extracts tested but at higher concentrations (5 and 10 mg/mL), aqueous extract showed a significantly higher (p<0.05) percentage inhibition of alpha-amylase when compared with acetone and ethanolic extracts. Aqueous extract of N. tabacum had the lowest IC50 (5.7 mg/mL) towards the inhibition of alpha-amylase (Table III). Kinetic analysis of the alpha-amylase inhibition by aqueous extract of N. tabacum using Lineweaver-burke plot shows that it displayed a non-competitive mode of inhibition (Figure 2).
Figure 1: Percentage inhibition of alpha-amylase by different extracts of N. tabacum. Bars carrying different letters at the same concentration are significantly different
Figure 2: Mode of inhibition of alpha-amylase by aqueous extract of N. tabacum
Table III: IC50 values of various extracts of N. tabacum against alpha-amylase and α-glucosidase
Extracts | IC50 (mg/mL) | |
---|---|---|
alpha-Amylase | alpha-Glucosidase | |
Acetone | 12.2 ± 1.3a | 4.5 ± 0.1a |
Ethanol | 18.3 ± 1.1b | 10.1 ± 0.6b |
Water | 5.7 ± 0.2c | 5.3 ± 0.2a |
Test values down the columns carrying different superscripts are significantly different (p<0.05) |
Figure 3 showed the alpha-glucosidase inhibitory activities of the N. tabacum extracts at different concentrations (0.6-5.0 mg/mL). alpha-Glucosidase inhibition by the different extracts was found to be dose dependent. At lower concentrations (0.6 and 1.3 mg/mL), there was no significant difference in the inhibition of the enzyme by the three extracts but at higher concentrations, significant differences (p<0.05) were noted among the extracts. The acetone extract of N. tabacum displayed the lowest IC50 (4.5 mg/mL) for the inhibition of alpha-glucosidase (Table III). The mode of inhibition of alpha-glucosidase by the acetone extract of N. tabacum obtained from Lineweaver-Burke plot was competitive (Figure 4).
Figure 3: Percentage inhibition of alpha-glucosidase by different extracts of N. tabacum. Bars carrying different letters at the same concentration are significantly different
Figure 4: Mode of inhibition of alpha-glucosidase by acetone extract of N. tabacum
Discussion
Hyperglycemia, a condition characterized by an abnormal postprandial increase of blood glucose level has been linked to the onset of type 2 diabetes mellitus and associated vascular complications (Di carli et al., 2003; Kwon et al., 2005). Drugs that reduce postprandial hyperglycemia by suppressing hydrolysis of starch have been found useful in the control of diabetes mellitus (Tundis et al., 2010; Kazeem et al., 2013a). Many herbal extracts have been reported for their antidiabetic activities and are currently being used in traditional medicines for the treatment of diabetes. However, such medicinal plants have not yet gained much importance as medicines due to lack of sustained evidence (Sudha et al., 2011).
Recent studies on N. tabacum indicated that it is used as an indigenous traditional medicine for various illnesses globally. It has already been reported to possess diverse bioactivities such as antioxidant (Ru et al., 2012), antimicrobial (Bakht et al., 2012), antinociceptive, antihelminthic and cardiovascular system activities (Ezeja and Omeh, 2010). However to our knowledge, despite its usage in traditional medicine as antidiabetic plant, there was no scientific report on the hypoglycemic effect of N. tabacum.
The results of this study showed that the aqueous extract of N. tabacum displayed the most effective inhibition of alpha-amylase activity with an IC50 of 5.7 mg/mL. This pointed to the fact that aqueous extract of N. tabacum is a mild inhibitor of alpha-amylase. This is in conformity with earlier reports that mild inhibitors of alpha-amylase are desired to be used as antidiabetic agents because excessive pancreatic alpha-amylase inhibition results in the abnormal bacterial fermentation of undigested carbohydrates in the colon leading to gastrointestinal side effects (Kwon et al., 2008b). The non-competitive inhibition displayed by the aqueous extract of N. tabacum towards alpha-amylase implies that the active components in the extract binds to a site other than the active site of the enzyme and combines with either free enzyme or the enzyme-substrate complex, possibly interfering with the action of both (Mayur et al., 2010; Kazeem et al., 2013b).
The acetone extract of N. tabacum possessed the lowest IC50 (4.5 mg/mL) for alpha-glucosidase which implies that it displayed the most effective inhibition towards this enzyme. The curve obtained for the Lineweaver-Burke plot showed that the mode of inhibition of alpha-glucosedase by the acetone extract of N. tabacum is competitive. This implies that the active component of the extract resembles the actual substrate of the enzyme structurally thereby binding to the active site of the enzyme and preventing the actual substrate from binding (Shai et al., 2010). Consequently, the extract prevents the digestion of disaccharides by α-glucosidase and thereby reduces glucose absorption in the intestine (Kazeem et al., 2013c).
Phytochemicals are secondary metabolites of plants origin and are important in providing potential antioxidant benefits for managing oxidative stress-related chronic diseases such as diabetes and cardiovascular diseases (Kwon et al., 2008b). The inhibitory potential of the extracts of N. tabacum against the activities of alpha-amylase and α-glucosidase may be due to the presence of phytochemicals such as tannins, saponins and flavonoids. Studies have shown that tannins possess multiple biological activities including antioxidant, anti-cancer and antimicrobial activities (Gin et al., 1999; Amarowicz., 2007). Tannins have also been reported to have health benefits in Alzheimer and diabetes (Ono et al., 2004). Tannins induced phosphorylation of the insulin receptors as well as translocation of glucose transporters 4 (GLUT-4) , the protein factor involved in the signaling pathway of insulin-mediated glucose transport and further demonstrated the inhibition of the expression of key gene for adipogenesis thereby helping to reduce blood glucose level without increasing adiposity (Liu et al., 2005).
Flavonoids, on the other hand, are phenolic compounds that also possess antioxidant and antidiabetic potentials due to the presence of hydroxyl groups that confer scavenging ability on them (Mayur et al., 2010). They are known as powerful chain breaking antioxidants and are also involved in the prevention of lipid peroxidetion. According to Song et al. (2005), flavonoids may preserve beta-cell function by reducing oxidative stress-induced tissue damage and therefore protect against the progression of insulin resistance to type 2 diabetes.
It can be concluded from this study that aqueous and acetone extract of N. tabacum inhibited the activities of alpha-amylase and alpha-glucosidase respectively. Therefore, their activities will slow down the hydrolysis of carbohydrates to glucose thereby preventing hyperglycemia. This biological activity of this plant may be attributed to the synergistic potential of the phytochemicals present in it especially tannins and flavonoids.
References
Ali H, Houghton PJ, Soumyanath A. Alpha-amylase inhibitory activity of some Malaysian plants used to treat diabetes with particular reference to Phyllanthus amarus. J Ethnopharmacol. 2006; 107: 449-55.
Amarowicz R. Tannins: The new natural antioxidants. Eur J Lipid Sci Tech. 2007; 109: 549-51.
Bakht J, Azra, Shafi M. Antimicrobial activity of Nicotiana tabacum using different solvents extracts. Pak J Bot. 2012; 44: 459-63.
Brownlee M. The pathobiology of diabetic complications: A unifying mechanism. Diabetes 2005; 54; 1615-25.
Deshpande MC, Venkateswarlu V, Babu RK, Trivedi RK. Design and evaluation of oral bioadhesive controlled release formulations of miglitol, intended for prolonged inhibition of intestinal alpha-glucosidases and enhancement of plasma glycogen like peptide-1 levels. Int J Pharm. 2009; 380: 16-24.
Di carli MF, Janisse J, Grunberger G, Ager J. Role of chronic hyperglycemia in the pathogenesis of coronary microvascular dysfunction in diabetes. J Am Coll Cardiol. 2003; 41: 1387-93.
Ezeja MI, Omeh YS. Anti-nociceptive activities of the methanolic leaf extract of Nicotiana tabacum (Linn). Continental J Pharmacol Toxicol Res. 2010; 3: 5-10.
Gin H, Rigalleau V, Caubet O, Masquelier J, Aubertin J. Effects of red wine, tannic acid, or ethanol on glucose tolerance in non-insulin dependent diabetic patients and on starch digestibility in vitro. Metabolism. 1999; 48: 1179-83.
Hirsh AJ, Yao SY, Young JD, Cheeseman CI. Inhibition of glucose absorption in the rat jejunum: A novel action of alpha-D-glucosidase inhibitors. Gastroenterol. 1997; 113: 205-11.
Horii S, Fukasse K, Matsuo T, Kameda K, Asano N, Matsui Y. Synthesis and α-D-glucosidase inhibitory activity of N-substituted valiolamine derivatives as potent oral antidiabetic agents. J Med Chem. 1987; 29: 1038-46.
Jacobi J, Jag JJ, Sundram U, Dayoub H, Farjado LF, Cooke JP. Nicotine accelerates angiogenesis and wound healing in genetically diabetic mice. Am J Pathol. 2002; 161: 97-104.
Kazeem MI, Abimbola SG, Ashafa AOT. Inhibitory potential of Gossypium arboreum Linn leaf extracts on diabetes key enzymes (α-amylase and α-glucosidase). Bangladesh J Pharmacol. 2013a; 8: 149-55.
Kazeem MI, Dansu TV, Adeola SA. Inhibitory effect of Azadirachta Indica A. Juss Leaf Extract on the activities of α-amylase and α-glucosidase. Pak J Biological Sci. 2013b; 16: 1358-62.
Kazeem MI, Oyedapo BF, Raimi OG, Adu OB. Evaluation of Ficus exasperata Vahl leaf extracts in the management of Diabetes mellitus in vitro. J Medical Sci. 2013c; 13: 269-75.
Kim YM, Jeong YK, Wang MH, Lee WY, Rhee HI. Inhibitory effects of pine bark extract on alpha-glucosidase activity and postprandial hyperglycemia. Nutrition 2005; 21: 756-61.
Kwon YI, Apostolidis E, Shetty K. In vitro studies of eggplant (Solanum melongena) phenolics as inhibitors of key enzymes relevant for type 2 diabetes and hypertension. Bioresource technol. 2008a; 99: 2981-88.
Kwon YI, Apostolidis E, Shetty K. Inhibitory potential of wine and tea against α-amylase and α-glucosidase for management of hyperglycemia linked to type 2 diabetes. J Food Biochem. 2008b; 32: 15-31.
Kwon YI, Vattem DA, Shetty K. Evaluation of clonal herbs of Lamiaceae species for management of diabetes and hypertension. Asia Pac J Clin Nutr. 2005; 15: 107-18.
Liu X, Kim J, Li Y, Li J, Liu F, Chen X. Tannic acid stimulates glucose transport and inhibits adipocyte differentiation in 3T3-Li cells. J Nutr. 2005; 135: 165-71.
Mayur B, Sandesh S, Shruti S, Sung-Yum S. Antioxidant and α-glucosidase inhibitory properties of Carpesium abrotanoides L. J Medicinal Plant Res. 2010; 4: 1547-53.
Mccue PP, Shetty K. Inhibitory effects of rosmarinic acid extracts on porcine pancreatic mylase in vitro. Asia Pac J Clin Nutr. 2004; 13: 101-106.
Ono K, Hasegawa K, Naiki H, Yamada M. Anti-amyloidogenic activity of tannic acid and its activity to destabilize Alzheimers β-amyloid fibrils in vitro. Biochem Biophys Acta. 2004; 1690: 193-202.
Ren N, Timko M. AFLP analysis of genetic polymorphism and evolutionary relationship among cultivated and wild Nicotiana species. Genome 2001; 44: 559-71.
Ru Q, Wang L, Li W, Wang J, Ding Y. In vitro antioxidant properties of flavonoids and polysaccharides extract from tobacco (Nicotiana tabacum L.) leaves. molecules. 2012; 17: 11281-91.
Shai LJ, Masoko P, Mokgotho MP, Magano SR, Mogale AM, Boaduo N, Eloff JN. Yeast alpha glucosidase inhibitory and antioxidant activities of six medicinal plants collected in Phalaborwa, South Africa. South Afr J Bot. 2010; 76: 465-70.
Smith C, Marks AD, Lieberman M. Mark’s basic medical biochemistry: A clinical approach. 2nd ed. USA, Lipincott Williams and Wilkins, Maryland, 2005, pp 2120-36.
Sofowara A. Medical plants and traditional medicine in Africa. Rep. edition, Ibadan, Spectrum Books Ltd., 2006, pp 191-289.
Song W, Wang H, Bucheli P, Zhang P, Wei D, Lu Y. Phytochemical profiles of different Mulberry (Morus. sp.) species from China. J Agric Food Chem. 2009; 57: 9133-40.
Sudha P, Zinjarde SS, Bhargava SY, Kumar AR. Potent α-amylase inhibitory activity of Indian Ayurvedic medicinal plants. BMC Compl Alternative Med. 2011; 11: 1-10.
Trease GE, Evans WC. Pharmacognosy. 4th ed. USA, W.B. Saunders, 1996, pp 243-83.
Tundis R, Loizzo MR, Menichini F. Natural products as α-amylase and α-glucosidase inhibitors and their hypoglycemic potential in the treatment of diabetes: An update. Minirev Med Chem. 2010; 10: 315–31.
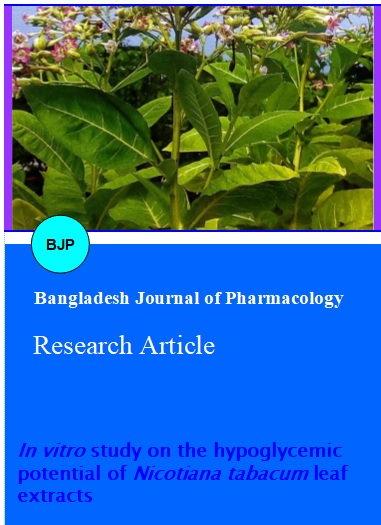