Design and docking of novel series of hybrid xanthones as anti-cancer agent to target human DNA topoisomerase 2-alpha
Abstract
Topoisomerase (topo) II is a homodimeric protein catalyzes topological vicissitudes by adding or by soothing super coiling transpiration, occurs in human DNA during DNA replication as an outcome chromosome segregation and condensation occurs during meiosis I and recombination. To prevent the cleavage and religation activity we administered novel hybrid substituted Xanthone series of drugs. The toxicity prediction showed outstanding results which impetus to study its anti-cancer activities by targeting topoisomerase (topo) IIα. We developed the homology model of the topoisomerase (topo) IIα due to the unavailability of 3D structure in the Protein Data Bank. Structural assessment of the modeled protein and confirmed the quality of the model. The ligands were docked using AutoDock 4.2 software and binding energy was reported. The compound XM9, XN2, XM7, XLNU and XNS scored lowest binding energy and highest binding affinity. The interaction sites and the hydrogen bond were observed.
Introduction
Enzymes that catalyze topological alterations of DNA are called topoisomerase. Topoisomerase can relax or add supercoiling and is in thetop of hits for anti-cancer drugs. DNA-intercalating agents have the ability to cause lethal DNA double-strand breaks, by hindering normal functioning of topoisomerase II. Topoisomerase II requires Mg (II) and ATP hydrolysis for enzyme turn over and rapid kinetics (Champoux, 2001).
Human topoisomerases II are of two types (i) topoisomerase I, (ii) topoisomerase II. Topoisomerase II has two distinct DNA-independent binding pockets, one within the catalytic domain and second within the N-terminal ATP-binding domain (Vilain et al., 2003). Topo II plays a key role in DNA replication with main functions are chromosome segregation, chromosome condensation, arrest in meiosis I and recombination suppression (Watt and Hickson, 1994). In cancer cells, topo II concentrations are dramatically up-regulated because of rapid cell division and cell growth (Heck and Earnshaw, 1986; Woessner et al., 1991). Therefore, numbers of anti-cancer agents are designed with topo II as a potential target (Wilstermann and Osheroff, 2003; Fortune et al., 2000).
The primary mode of cytotoxicity of most DNA intercalating agents involves inhibition of religation step of action of the enzyme DNA topo II (Liu, 1989; Robinson and Osheroff, 1991). Xanthonescan also binds and shows anti-cancer activity by forming a stable binding complex with N-terminal ATP-binding domain of topo II (Jun et al., 2011) like novobiocin (Larsen et al., 2003), cyclothialidine (Boehm et al., 2000) and salvicine (Hu et al., 2006). Xanthone fights competitively with ATP to binds with the ATP binding site on topo IIα and directly hampers the energy driven rapid kinetics which lead to a higher topo II catalytic inhibitory activity.
Materials and Methods
Sequence alignment
A Gonnet protein weight matrix of CLustal W was used to study the matching between target and template sequences. Based on the sequence, pair wise and multiple sequence alignment were performed. For alignment, the alignment tools, t-coffee, CLustal W from EBI (European Bioinformatics Institute) was used to better understand resultant sequence alignment matches, its mismatches and mutations was viewed in Jalview.
Homology modeling
Homology modeling builds a neat relationship between protein primary and secondary structure. It is very clear to understand the function of protein computationally by comparative modeling of a target protein sequences with its template which is an experimental (XRD or NMR) protein structure This supposition provides an exclusive hypothesis that if the tertiary structure of two proteins are similar, they must shares high percentage of similarity scores or in other words, the tertiary structure of two proteins are similar and shares high percentage of similarity scores only, when they are similar (Dong and Berger, 2007). Modeller 9v11 software was used for protein model building. Initially 100 models were designed, among them a single model was selected based on the lower discrete optimized protein energy (DOPE) scores for further analysis.
Structural assessment
The models were examined both on geometric and energetic scale for quality means. The validation of modeled structures were done by using PROCHECK (Nitiss, 2009), ERRAT (Bailly, 2012) and VERIFY3D (Binaschi et al., 2001). PROCHECK rottenly used and is an important freeware tool, deals with the study of stereochemical properties which could be clearly analyzed by the quality of the Ramachandran plot, peptide bond planarity, non-bonded interactions, main chain hydrogen bond energy, Cα chiralities and overall G factor. ERRAT is a protein structure verification algorithm which analyzes the statistics of non-bonded interactions between different atom types, which in turn gives the reliability to the model. To check the compatibility of the atomic models with its own amino acid sequence, VERIFY3D was used. A high VERIFY3D profile score shows the healthier quality of model.
Preparation of protein structure
Topoisomerase II protein is big protein, having 1531 amino acid residues. Preparation of favorable binding site was done using AutoDock 4.2 Tools. Before going xyxanthones. Very lengthy or heavy alkoxy side chain does not improve interaction of basic nucleus sufficiently (X137, XMUN).
ADMET studies and drug likeness of designed 11 compounds were done by using Osiris property explorer, MedChem draw freeware. ADME profile shows that that these candidates are hydrophobic in nature and thus acted as hydrophilic neutral drug molecule by their obedience to the properties such as absorption, distribution, metabolism, and excretion (ADME) determined by using MedChem Designer draw freeware. Toxicity profile which includes tumeriogenicity, mutagenecity, in combination with failure in producing reproducible effect by compounds and drug scores were studied using Osiris property explorer, all compounds except XN2, XN2 does not passes through these filters. Three compounds X137, XM7 and XNT got highest drug likeness score of 3.9, 3.7 and 2.1 respectively (Table III).
Xyxanthones, very lengthy or heavy alkoxy side chain does not improve interaction of basic nucleus sufficiently (X137, XMUN).
ADMET studies and drug likeness of designed 11 compounds were done by using Osiris property explorer, MedChem draw freeware. ADME profile shows that that these candidates are hydrophobic in nature and thus acted as hydrophilic neutral drug molecule by their obedience to the properties such as absorption, distribution, metabolism, and excretion (ADME) determined by using MedChem Designer draw freeware. Toxicity profile which includes tumeriogenicity, mutagenecity, in combination with failure in producing reproducible effect by compounds and drug scores were studied using Osiris property explorer, all compounds except XN2, XN2 does not passes through these filters. Three compounds X137, XM7 and XNT got highest drug likeness score of 3.9, 3.7 and 2.1 respectively (Table III).
with Met762 and hydrophobic interaction with Met766 respectively. Alkoxy side chain of XM7 shows important interactions with Ser800, IIe769, Asn770 and Met760. Targeted protein topo II α, has most potential active site where the ligand could bind and interact was already previously identified and reported. The residues which were found to be taking part actively in dimensioning the active binding pocket of topo II, are reported as follows : PRO 716, ASP 720, GLY 721, LEU 722, LYS 723, GLN 726, ASN 770, LEU 771, GLN 773, PHE 775, GLY 777, SER778, ASN 779, LEU 781, LEU 783, GLY 796, LYS 798, MET 847, VAL 848, LEU 849, ILE 850, ASN 851, GLY 852, ALA 853, GLU 854, LYS 863, ILE 864, PRO 865, ASN 866, TYR 892 and ARG 929. Compound XM7 binds correctly in this pocket and was revealed by docking results. Other compounds also shows interactive binding energies against topo IIα during molecular docking studies, not in same pocket because topo II proteins composed of 5 active binding pockets where ligand/drug could bind, analyzed by Q- site finder software which is an online freeware. The binding pocket and the surface analysis of the compound XM7 was given in the (Figure 3) and The hydrophobic and hydrogen bond interaction was analyzed (Figure 4). Binding energy is a versatile tool understands the affinity of the ligand to its binding site present on protein. Five compounds XM9, XN2, XM7, XLNU, XNS showed best and lowest binding energy scores among all of the ligands having -14.3, -13.0, -12.7,-11.9, -11.3 Kcal/molrespectively. All five compounds were found to be more potent than naturally ocurring marine based triterpeneglycosides, cucumarioside A with docking score -11.1 kcal/mol followed by holothurinoside A and holothurin A with -10.5 kcal/mol, bivittoside A with -10 kcal/mol, holotoxin A with -9.7 kcal/mol as well as than etoposide with binding energy of -9.5 kcal/mol. Compound X137 got least binding energy -6.4 kcal/mol. Binding energies of all compound are presented in Table IV. The polar interacting residues forming hydrogen bonds were analyzed (Figure 5).
Figure 1: Sequence alignment between query (topoisomerase II α) of human and subject (4GFH A)
Figure 2: Ramachandran Plot showed that most of the residues of the modeled contains most of the residues in favored region (85.5%), additional region (12.9%), generously allowed region (1.2%) and only 3 residues (GLU 1102, GLU 1109 and SER 1115) i.e 0.4% in disallowed residues
Figure 3: The surface view of the protein with the ligand (XM7) binding pocket
Figure 4: Hydrophobic and hydrogen bond forming residues of the docked complex of XM7 and the model. Hydrophobic interactions are shown in red dotted line and hydrogen bonds are shown in green dotted line
Figure 5: The polar contacts of the compound XM7 with the binding site of the modeled protein. The yellow dotted line shows the polar contacts formed and were found to be ASN770 and LYS 798
Table I: Chemical structures of 11 novel hybrid substituted xanthones
Compound name | R1 | R2 | R3 |
---|---|---|---|
XM7 | -----H | -----OH | |
XM8 | -----H | -----OH | |
XM9 | -----H | -----OH | |
X137 | -----H | -----H | -----OH |
XL2 | -----H | -----H | |
XLNU | -----H | -----H | |
XN2 | R3 = OR2 | -----H | |
XNT | R3 = OR2 | -----H | |
XLS | -------C12H25" alt="" width="31" height="19" /> | -----H | -----H |
XNS | -------C12H25" alt="" width="31" height="19" /> | ![]() |
-----H |
XMUN | -------C12H25" alt="" width="31" height="19" /> | -----H | -----OH |
Table II: Molecular descriptors calculated for different novel substituted hybrid xanthones using MedChem designer software
Compound name | SlogP | S+logD | MlogP | HBDH | M_NO | TPSA | Rule of 5 | HBA |
---|---|---|---|---|---|---|---|---|
XM7 | 2.8 | 2.8 | 1.6 | 4.0 | 8.00 | 107.20 | 0.0 | 8 |
XM8 | 3.1 | 3.1 | 1.8 | 4.0 | 8.00 | 107.20 | 0.0 | 8 |
XM9 | 3.5 | 3.5 | 2.0 | 4.0 | 8.00 | 107.20 | 0.0 | 8 |
X137 | _ | _ | 3.6 | 2.0 | 5.00 | 79.9 | 0.0 | 5 |
XL2 | _ | _ | 4.0 | 1.0 | 8.0 | 100.0 | 0.0 | 8 |
XLNU | 3.4 | 3.3 | 2.1 | 2.0 | 9.0 | 121.4 | 0.0 | 7 |
XN2 | _ | _ | 2.6 | 3.0 | 15.0 | 192.4 | 2.0 | 11 |
XNT | _ | _ | 0.5 | 7.0 | 13.0 | 203.9 | 2.0 | 13 |
XLS | 8.2 | 8.2 | 4.1 | 1.0 | 4.0 | 59.7 | 0.0 | 4 |
XNS | 11.6 | 11.6 | 5.8 | 1.0 | 5.0 | 68.9 | 2.0 | 5 |
XMUN | _ | _ | 3.6 | 2.0 | 5.0 | 79.9 | 0.0 | 5 |
Result and Discussion
Topoisomerase II poisons, are efficient but produce harmful secondary effects, common with every drug follows this path, were myelosuppression, leucopoenia, gastrointestinal toxicities, alopecia, and even leukemia (Bailly, 2012). But taking safety as a principle criteria, topo II catalytic inhibitors are preferential ones over topo II poisons for designing.
In cancer cells, topo II concentrations are dramatically upregulated because of rapid cell division and cell growth (Heck and Earnshaw, 1986; Woessner et al., 1991). Therefore, numbers of anti-cancer agents are designed with topo II as a potential target (Wilstermann et al., 2003; Fortune and Prog, 2000). Trihydroxyl xanthone and tetrahydroxyl xanthone exhibited the highest cytotoxic activities over other compounds. A piperidine side chain at the C-3 position is favorable with regard to improved cytotoxicity. Quinoline-containing thiosemicarbazide compound (TSC24), have potent anti-proliferative activity toward cancer cells. Nitrogen containing groups like nitro, amide and amines in chemical structure are general requirement for designing topo II inhibitors. Various alkylating agents, nitrogen mustered and natural anti-cancer molecules have nitrogen containing groups, which generally interact with DNA/base pair/protein and alkylate them or done other changes which ultimately governs their anti-cancer activity. Nitrogen containing are various moieties like triazole, thiosemicarbazoles and nitrosourea were used in this study for designing.
These previously reported results gives an idea about designing some new xanthone based anti-cancer molecules, because xanthone moiety shows promising an anti-cancer potential, proven through number of experimental studies. Hybrid based designing of new molecules against various diseases were tried and found to be much more effective, as compared to their parent molecules. Through this point of view, few new hybrid molecules were designed and docking studies were done to determine ligand-protein interactions.
Drugs aimed against topoisomerases could work by one or both of two ways (a) by hindering the ability of the enzyme to relieve tension of DNA by preventing its initial cleavage function (b) by preventing relegation of the "cleavable complex" means stabilizing the transient cleavable complex, results in enhanced strand breaks. The mode of action to show cytotoxic behavior for majority of topo inhibitors (topo poisons) is just due to account of their ability to stabilizes a transient DNA enzyme complex result DNA damage thus produces detrimental secondary effects.
The structure of xanthone generally resembles more specifically to amsacrine, which is a known branded anti-cancer drug, belongs to 9-anilinoacridines class of topoisomerase II inhibitors, having a heterotricyclic flat ring system. Xanthone may act via same mechanism as 9-anilinoacridines because of too much similarity in theirstructure. The cytotoxicity shown by these compounds, primarily results due to inhibition of topo II through formation of a ternary drug/DNA/protein complex (Liu, 1989; Robinson and Osheroff, 1991) gave a clear picture that drug design through modeling of DNA binding properties alone could be misleading. The antitumor activity of these drugs governed mostly, just because of their proper positioning and stabilization of the drug at its binding domain, than its (ligand or drug) actual affinity for DNA (Binaschi et al., 2001; Patil and Thakare, 2012). Among 11 compounds XM7 binds in same pocket and also interacts with same residues of topoisomerase IIα where cucumarioside A, holothurinoside A, holothurin A, bivittoside A and holotoxin A were found to be bounded which were founded to be more potent than etoposide as previously reported. The new hybrid compound XM7 having a simple primary structure and more potential candidate with having binding energy of about -12.7 Kcal/mol than some naturally occurring marine agents used as an anti-cancer agents with more complex chemical structures and interact with the most potential active site, considered (Patil and Thakare, 2012). However, five compounds out of 11 compound possesses lowest binding energy below -11.1 Kcal/mol. ADMET screening provided a clear cut picture about candidates under consideration and suggests for further modifications in following candidates.
Study gives an opinion that the designed molecules require further modifications to improve drug-receptor interactions. However binding energy data and docking studies revealed, a good picture of compounds affinity and fitting inside the binding pocket. Docking studies also revealed the mode of binding of new novel hybrid xanthones into the binding pocket of topo II. The designed compounds will be synthesized and evaluated for their anti-cancer potential. All designed compounds are under further modification to generate an ultimate molecule with desired activity and safety profile.
Table III: Solubility, drug likeness and drug score accounted by Osiris property explorer and with respect to binding energies of different novel substituted hybrid xanthones
Compound name | Solubility | Drug likeness | Drug score |
---|---|---|---|
XM7 | -5.4 | 3.7 | 0.4 |
XM8 | -5.7 | 0.3 | 0.3 |
XM9 | -6.0 | -1.6 | 0.2 |
X137 | -4.0 | -0.0 | 0.4 |
XL2 | -7.2 | 3.9 | 0.2 |
XLNU | -6.0 | 0.9 | 0.1 |
XN2 | -7.7 | -8.0 | 0.0 |
XNT | -7.3 | 2.1 | 0.4 |
XLS | -7.7 | -20.4 | 0.1 |
XNS | -10.7 | -22.3 | 0.1 |
XMUN | -7.4 | -20.8 | 0.1 |
Table IV: Solubility, drug likeness and drug score accounted by Osiris property explorer and with respect to binding energies of different novel substituted hybrid xanthones
Compound name | Residues involves in hydrogen bonding interactions | Residues involves in hydrophobic interactions | Binding energy |
---|---|---|---|
XM7 | Met762 | Pro803, Ser800, IIe769, Gln773, Gly797, Gly796, Asn770, Lys798, Met766 | -12.7 |
XM8 | Gln544 | Arg672, Pro601, Leu680, Glu682, Pro593, Tyr590, Ser591, Glu542, Tyr686 | -11.0 |
XM9 | Pro593 | Try686, Gln542, Tyr590, IIe577, Ser591, Leu705, Leu592, Tyr684, Glu602, Asp683, Arg675, Lys701, Asp671, IIe704, Asn700, Phe668, Tyr686 | -14.3 |
X137 | Lys1140 | Tyr1135, Met1131, Pro1132, Leu128, Asp1130, Phe1054, IIe1055 | -6.4 |
XL2 | - | Ser756, Asp832, Tyr757, Glu702,Leu685, Gln542, Tyr686, Ser591, Pro593, Leu592, Asp543, Gly615, Lys614, IIe577 | -10.5 |
XLNU | Asp832 | Tyr757, Lys614, Gln544, Leu705,Leu685, Glu702, Ser591, Tyr686, Tyr590, Leu592, Glu542, IIe577, Ser756 | -11.9 |
XN2 | IIe577 | Leu592, His758, Ser756, Asp631, Lys614, Lue705, Phe668, Asp671, Arg675, Lys701, Ser591, Asp683, Tyr686, Gln542 | -13.0 |
XNT | _ | Asn700, Asp683, Arg675, Lys701, Ser591, Leu705, Leu685, Gln542, Tyr686, Glu702, Ser547, Pro593, Glu682, Phe668 | -9.5 |
XLS | Leu592 | Ser591, Tyr686, Asp543, His758, Gln542, Gln544, Leu685, Pro593 | -7.8 |
XNS | Lys520 | Ser527, IIe530, Asp526, Lys529, Asn433, Arg532, Gly448, IIe511, IIe435, Gly515, Tyr518, Lys519, Gln517, Leu516 | -11.3 |
XMUN | Pro593 | His758,Leu592, IIe577, Glu702, Tyr686, Leu685, Tyr684, Gln542, Ser591, Leu705, Glu682, Glu544 | -6.8 |
All 11 candidates were found to be showing excellent activity, out of which three candidates were found to be more potent than previously reported candidates as an anti-cancer agent. Compound XM7 was found to be interacting with Pro803, Ser800, IIe769, Gln773, Gly797, Gly796, Asn770, Lys798, Met766 and Met762, which was previously reported to constitute the most active site of human DNA topoisomerase II, through which various marine based anti-cancer triterpeneglycosides like cucumarioside A, holothurinoside A, holothurin A, bivittoside A, holotoxin A acts (Patil and Thakare, 2012). Thus we could conclude through these docking studies that five compoundsXM9, XN2, XM7, XLNU and XNS could be act as a potential lead to evolve a potential anti-cancer candidate against human DNA topoisomerase IIα inhibitors.
References
Bailly C. Contemporary challenges in the design of Topoisomerase II inhibitors for cancer chemotherapy. Chem Rev. 2012; 112: 3611-40.
Binaschi M, Bigioni M, Cipollone A, Rossi C, Goso C, Maggi CA, Capranico G, Animati F. Anthracyclines: Selected new developments. Curr Med Chem Anti-cancer Agents. 2001; 1: 113-30.
Boehm HJ, Boehringer M, Bur D, Gmuender H, Huber W, Klaus W, Kostrewa D, Kuehne H, Luebbers T, Meunier-Keller N, Mueller F. Novel inhibitors of DNA gyrase: 3D structure based biased needle screening, hit validation by biophysical methods, and 3D guided optimization. A promising alternative to random screening. J Med Chem. 2000; 43: 2664-74.
Champoux JJ. DNA topoisomerases: Structure, function, and mechanism. Annu Rev Biochem. 2001; 70: 369-413.
Dong KC, Berger JM. Structural basis for gate-DNA recognition and bending by type IIA topoisomerases. Nature 2007; 450: 1201-05.
Fortune JM, Prog ON. Topoisomerase II as a target for anti-cancer drugs: When enzymes stop being nice. Prog Nucleic Acid Res Mol Biol. 2000; 64: 221-53.
Heck MM, Earnshaw WC. Topoisomerase II: A specific marker for cell proliferation. J Cell Biol. 1986; 103: 2569-81.
Hu CX, Zuo ZL, Xiong B, Ma JG, Geng MY, Lin LP, Jiang HL, Ding J. Salvicine functions as novel topoisomerase II poison by binding to ATP pocket. Mol Pharmacol. 2006; 70: 1593-601.
Jun KY, Lee EY, Jung MJ, Lee OH, Lee ES, Park Choo HY, Na Y, Kwon Y. Synthesis, biological evaluation, and molecular docking study of 3-(30-heteroatom substituted-20-hydroxy-10-propyloxy) xanthone analogues as novel Topoisomerase IIa catalytic inhibitor. Eur J Med Chem. 2011; 46: 1964-71.
Larsen AK, Escargueil AE, Skladanowski A. Catalytic topoisomerase II inhibitors in cancer therapy. Pharmacol Ther. 2003; 99: 167-81.
Liu LF. DNA topoisomerase poisons as antitumor drugs. Ann Rev Biochem. 1989; 58: 351-75.
Nitiss JL. Targeting DNA topoisomerase II in cancer chemotherapy. Nat Rev Cancer. 2009; 9: 338-50.
Patil TD, Thakare SV. In silico evaluation of selected triterpene glycosides as a human DNA topoisomerase II alpha (α) inhibitor. Int J Pharm Pharm Sci. 2012; 4: 201-04.
Robinson MJ, Osheroff N. Effects of antineoplastic drugs on the post-strand-passage DNA cleavage/religation equili-brium of topoisomerase II. Biochemistry 1991; 30: 1807-13.
Shen R, Wang P, Tang N. Cytotoxic Activity and DNA-binding Properties of Xanthone Derivatives. J Fluoresc. 2010; 20: 1287-97.
Su QG, Liu Y, Cai YC, Sun YL, Wang B, Xian LJ. Anti-tumour effects of xanthone derivatives and the possible mechanisms of action. Invest New Drugs. 2011; 29: 1230-40.
Vilain N, Tsai-Pflugfelder M, Benoit A, Gasser SM, Leroy D. Modulation of drug sensitivity in yeast cells by the TP-binding domain of human DNA topoisomerase IIa. Nucleic Acids Res. 2003; 31: 5714-22.
Watt PM, Hickson ID. Structure and function of type II DNA topoisomerases. Biochem J. 1994; 303: 681-95.
Wilstermann AM, Osheroff N. Stabilization of eukaryotic topoisomerase II-DNA cleavage complexes. Curr Top Med Chem. 2003; 3: 321-38.
Woessner RD, Mattern MR, Mirabelli CK, Johnson RK, Drake FH. Proliferation- and cell cycle-dependent differences in expression of the 170 kilodalton and 180 kilodalton forms of topoisomerase II in NIH-3T3 cells. Cell Growth Differ. 1991; 2: 209-14.
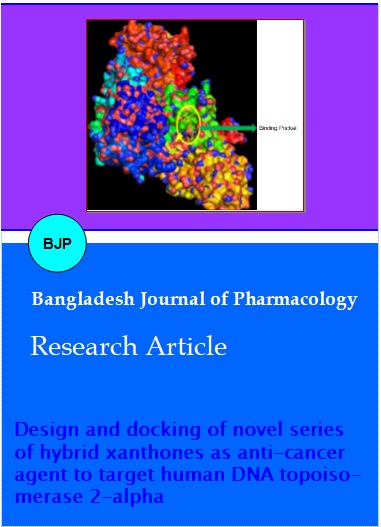