Effects of aqueous extract of Fadogia agrestis stem in alloxan-induced diabetic rats
Abstract
Aqueous extract of Fadogia agrestis stem at the doses of 18, 36, and 72 mg/kg body weight was investigated for antidiabetic activity in alloxan-induced diabetic rats. The results revealed that the administration of alloxan significantly increased (p<0.05) the levels of blood and hepatic glucose, serum urea, creatinine, albumin, total cholesterol, triacylglycerol, low-density lipoprotein cholesterol, atherogenic index and very low-density lipoprotein cholesterol whereas the organ/body weight ratio, activity of hexokinase, levels of high-density lipoprotein cholesterol, packed cell volume and hemoglobin reduced significantly. In contrast, all the doses of the extract significantly reversed the levels of these biomolecules/biochemical parameters. These reversals, however, did not compare well with their respective control values by the end of exposure period. Overall, the aqueous extract of F. agrestis stem showed promise as an antidiabetic agent.
Introduction
Several management options for diabetes such as insulin releasers, insulin sensitizers, drugs (sulfonylureas and metformin) and alpha-glucosidase inhibitors are associated with serious shortcomings like limited and decreased efficacy over time, ineffectiveness against some long-term diabetic complications and low cost-effectiveness, worsening of heart diseases, increased body weight and hypoglycaemia among others (Grover et al., 2002; Dey et al., 2003). Therefore, discovery and development of novel drugs from herbs will not be out of place. Herbal drugs or their extracts such as Cajanus scarabaeoides (Pattanayak et al., 2009), Lagerstroemia speciosa (Saha et al., 2009) and Teucrium stocksianum (Alamgeer et al., 2013), are prescribed widely even when their bioactive compounds are unknown (Gupta et al., 2005). Therefore, studies with plant extracts are useful in order to determine their efficacy, mechanism of action and bioactive agent(s) that are responsible for such pharmacological effect and safety.
Fadogia agrestis Schweinf, family-Rubiaceae (Hausa: Murshikan dutse; English: Black aphrodisiac) is an erect shrub that is 1-3 feet tall. It has tomentellous stems and leaves that are yellow in color. Ethnomedicinal survey of F. agrestis reveals a variety of uses such as aphrodisiac in tropical African countries (Yakubu et al., 2005). The plant stem has been reported to contain saponins, alkaloids, flavonoids, and anthraquinones (Yakubu et al., 2005) while two monoterpene glycosides have been isolated from the leaves of F. agrestis found in Guinea (Anero et al., 2008). In vitro antiplasmodial activity has been reported for extracts from leaves collected in Burkina Faso (Sanon et al., 2003) whereas the aphrodisiac potentials of F. agrestis stem was supported with scientific evidence by Yakubu et al. (2005). The plant stem has also been reported to exhibit antimicrobial activity and cytotoxic effects (Ameen et al., 2011).
However, despite these studies that have been reported on the chemical constituents and pharmacological activities of F. agrestis, there is dearth of information in open scientific literature on the effects of this plant on diabetic rats. In the light of the aforementioned, this current study investigates the effects of aqueous extract of F. agrestis stem in alloxan-induced diabetic rats.
Materials and Methods
Plant material
The plant bought from herb sellers at a market (Kulende) in Ilorin, Nigeria, was authenticated at the Department of Horticulture and Landscape Technology, Federal School of Forestry, Jos, Nigeria, with a voucher number 2:108. A voucher specimen was deposited at the Herbarium of the Department.
Experimental animals
A total of 42 albino rats of both sexes weighing 128.4 ± 1.6 g, housed in clean aluminium cages, placed in well ventilated standard housing conditions (temperature: 28-31°C; photoperiod: 12 hours light/dark cycle; humidity: 40-45%) was used for the study. The animals were allowed free access to rat pellets (Premier Feed Mill Co. Ltd., Ibadan, Nigeria) and tap water and the cages were also cleaned on daily basis. The animals were acclimatized for two weeks before the commencement of the experiment.
Chemicals and assay kits
Alloxan monohydrate and Glibenclamide were products of Sigma Chemical Company, USA and HOVID Bhd., Ipoh, Malaysia respectively. The assay kits for glucose, triacylglycerol, total cholesterol, HDL-C, urea, creatinine and albumin were products of Randox Laboratories Ltd., UK.
Glucometer and hematological analyzer
Accu-check active glucometer and strips were products of Roche Diagnostic, Mannheim, Germany. Automated hematological analyzer SYSMEX KX21 was a product of SYSMEX Corporation, Harrier, Japan.
Preparation of the extract
The method described by Yakubu et al (2005) was used. The plant stem was cut into pieces, and oven dried at 40°C for 3 days to a constant weight. The dried pieces were then pulverized using a blender (Super Master Co., Ltd, Osaka, Japan). A known weight of the powder (160 g) was extracted in 3,200 mL of distilled water with thorough shaking at regular intervals for 48 hours at room temperature. This was then filtered using filter paper (Whatman No. 1). The filtrate was concentrated on a steam bath to give 32.1 ± 0.5 g of the residue corresponding to a percentage yield of 20.1%. The resulting black residue was reconstituted in distilled water to give the equivalent dose of 18 mg/kg body weight, while higher doses of 36 and 72 mg/kg body weight were also used in this study.
Induction of diabetes
After a six hours of fast (water only), the fasting blood glucose levels of the animals were determined before the administration of alloxan by drawing blood samples (0.6 µL) from the sharply cut tail vein and placing it on the test strip that had been inserted into the glucometer. Thereafter, diabetes mellitus was induced in the animals by single intraperitoneal injection of 1 mL of 150 mg/kg body weight of alloxan monohydrate prepared in sterile physiological saline. Furthermore, 1 hour after the administration of alloxan, the animals were equally given their pellets ad libitum and 5%dextrose saline in a feeding bottle to overcome the early hypoglycaemic phase (Aruna et al., 1999). After 48 hours of alloxan administration, blood glucose was again determined to confirm the induction of diabetes. Only animals with fasting blood glucose level higher than 230.0 mg/dL were used for the study (Aruna et al., 1999).
Animal grouping
The rats were completely randomized into six groups (A-F) of 7 animals each (Table I).
Table I: Grouping and treatment of animals
Groups | Treatment |
---|---|
A | 0.5 mL of distilled water once daily |
B | 150 mg/kg body weight of alloxan + 0.5 mL of distilled water |
C | 150 mg/kg body weight of alloxan + 0.5 mL corresponding to 2.5 mg/kg body weight of glibenclamide |
D | 150 mg/kg body weight of alloxan + 0.5 mL corresponding to 18 mg/kg of the extract |
E | 150 mg/kg body weight of alloxan + 0.5 mL corresponding to 36 mg/kg of the extract |
F | 150 mg/kg body weight of alloxan + 0.5 mL corresponding to 72 mg/kg of the extract |
The extract, glibenclamide and distilled water were administered orally, once daily for 15 days between 0800-0900 hours. They were allowed free access to rat feed and water before and after their daily administration except when fasting blood glucose levels were determined.
Preparation of serum and tissue supernatants
The method described by Yakubu et al. (2008) was used for the preparation of serum and tissue supernatants. After 15 days of treatment, the animals were anesthetized in diethyl ether and sacrificed by simply incising the jugular vein; the blood was collected into heap rinized and plain sample tubes for hematological and serum analysis respectively. Blood samples were left undisturbed at room temperature for 30 min to form clot after which the samples were centrifuged at 1282 x g for 5 min. After centrifugation, the supernatant which was the serum was collected using Pasteur pipette into clean sample bottles. The serum, thus obtained were appropriately labelled and used within 12 hours of preparation for the biochemical assays. The kidney, liver and pancreas were also removed, freed from fat, blotted with tissue paper and weighed for the computation of organ-body weight ratio. The organs were separately homogenized in ice-cold 0.25M sucrose solution (1:5 w/v). The homogenates were centrifuged at 1789 x g for 10 min to obtain the supernatants which were stored frozen and used for biochemical analysis within 24 hours of preparation.
Determination of biochemical parameters
The biochemical parameters determined in the present study included hexokinase activity (Brandstrup et al., 1957), hepatic and serum glucose (Barham and Trinder, 1972), hepatic glycogen (Kemp and Kits Van Heijningen, 1954), total cholesterol (Fredrickson et al., 1967), HDL-cholesterol (Hainline et al., 1980), LDL-cholesterol (Friedewald et al., 1972), atherogenic index (Ng et al., 1997) as well as urea, triacylglycerol, creatinine and albumin (Tietz, 1995). Blood glucose was determined using an Accu-check active machine and strip while VLDL-C was calculated as 1:2.2 of triacylglycerol. The organ-body weight ratio expressed in percentage was computed using the expression described by Yakubu et al (2008). Packed cell volume (PCV) and hemoglobin were determined using Wintrobe's microhematocrit and Sahli's methods respectively (Shalm et al., 1995).
Statistical analysis
Results were expressed as the mean ± SEM of seven determinations. The data were analysed using Analysis of Variance and complemented with Tukey's Post hoc. The differences were considered statistically significant at p<0.05. All the analyses were done using SPSS version 20.0 software (SPSS Inc., USA).
Results
Administration of alloxan significantly (p<0.05) elevated the blood glucose level of the animals when compared with the non-diabetic animals treated with distilled water. The blood glucose level of the diabetic rats treated with distilled water continued to increase and by the 15th day of the experimental period, it has increased by 72.9%. The extract at the doses of 18, 36 and 72 mg/kg body weight significantly (p<0.05) reduced the blood glucose levels in a dose-dependent manner. The reduction by the end of the exposure period did not compare well with the non-diabetic animals treated with distilled water. In contrast, 2.5 mg/kg body weight of glibenclamide significantly (p<0.05) reduced the blood glucose levels of the diabetic animals to values that compared well with non diabetic distilled water treated control (Table II).
Table II: Blood glucose level of alloxan-induced diabetic rats administered aqueous extract of Fadogia agrestis stem
Treatment days | Blood glucose (mg/dL) | |||||
---|---|---|---|---|---|---|
Control | Diabetic rat treated with | |||||
Distilled water | Glibenclamide | F. agrestis (mg/kg body weight) | ||||
18 | 36 | 72 | ||||
0 | 75.3 ± 2.2a | 234.9 ± 30.5b | 300.4 ± 33.0b | 291.7 ± 39.4b | 298.0 ± 46.1b | 311.0 ± 31.4b |
5 | 74.3 ± 2.5a | 263.1 ± 25.4b | 275.3 ± 29.6b | 291.7 ± 35.1b | 241.7 ± 21.7b | 234.4 ± 18.6b |
10 | 74.6 ± 2.7a | 266.5 ± 19.4e | 121.8 ± 4.8b | 187.7 ± 7.8d | 166.2 ± 10.5d | 130.8 ± 16.3c |
15 | 74.4 ± 1.6a | 277.3 ± 17.9d | 76.2 ± 5.4a | 121.3 ± 4.2c | 116.5 ± 8.9bc | 92.0 ± 11.0b |
Data are mean ± SEM of seven determinations. Test values with superscripts b, c and d different from the control down the column are significantly different (p<0.05) |
There were significant increases (p<0.05) in the hepatic and serum glucose, urea and creatinine levels as well as significant decrease (p<0.05) in the activity of hexokinase and levels of glycogen, albumin, PCV, and hemoglobin of the animals following the administration of alloxan. The extract at various doses (18, 36, and 72 mg/kg body weight) significantly (p<0.05) reversed the levels of all these parameters; the reversal however did not compare favorably with their non-diabetic distilled water and glibenclamide treated diabetic animals. The glibenclamide however restored the levels of these biomolecules to values that compared favorably with their controls (Table III).
Table III: Biochemical and hematological parameters of alloxan-induced diabetic rats administered aqueous extract of Fadogia agrestis stem
Control | Diabetic rat treated with | |||||
---|---|---|---|---|---|---|
Distilled water | Glibenclamide | F. agrestis (mg/kg body weight) | ||||
18 | 36 | 72 | ||||
Serum glucose (mmol/L) | 4.1 ± 0.1a | 9.8 ± 0.0e | 4.1 ± 0.1a | 7.9 ± 0.0d | 5.1 ± 0.1c | 5.1 ± 0.1b |
Hepatic glucose (mmol/L) | 1.7 ± 0.3a | 3.4 ± 0.2d | 1.8 ± 0.6a | 2.6 ± 0.1c | 2.7 ± 0.0c | 2.1 ± 0.2b |
Hepatic glycogen (mmol/L) | 1.8 ± 0.1a | 0.5 ± 0.0e | 1.7 ± 0.0a | 1.1 ± 0.0d | 1.2 ± 0.0c | 1.4 ± 0.0b |
Hexokinase (mmol/L) | 0.5 ± 0.0a | 0.1 ± 0.0e | 0.5 ± 0.0a | 0.3 ± 0.0d | 0.3 ± 0.0c | 0.4 ± 0.0b |
Serum urea (mmol/L) | 4.3 ± 0.1a | 10.8 ± 0.1e | 4.8 ± 0.1a | 10.5 ± 0.0d | 6.5 ± 0.0c | 5.0 ± 0.0b |
Serum creatinine (mmol/L) | 61.5 ± 0.3a | 91.0 ± 0.6e | 62.0 ± 0.4a | 89.3 ± 0.3d | 78.3 ± 0.5c | 70.3 ± 0.3b |
Albumin (g/L) | 38.2 ± 0.3a | 19.7 ± 0.5e | 37.7 ± 0.5a | 30.0 ± 0.4d | 33.5 ± 0.3c | 34.5 ± 0.5b |
PCV (%) | 49.7 ± 0.2a | 24.7 ± 0.2c | 40.6 ± 0.6a | 32.5 ± 0.5b | 33.0 ± 0.1b | 37.0 ± 1.0b |
Hb (gdL-1) | 13.9 ± 0.1a | 6.8 ± 0.1c | 13.3 ± 0.3a | 10.0 ± 0.2b | 10.3 ± 0.1b | 10.4 ± 0.4b |
Data are mean ± SEM of seven determinations. Test values with superscripts b, c,d and e different from the control, a, for each parameter are significantly different (p<0.05) |
There was significant (p<0.05) reduction in the absolute body weight and organ/body weight of rats following the administration of alloxan. Treatment of the diabetic animals with the various doses of the plant extract and glibenclamide significantly (p<0.05) increased the body weight. The increases were however more than the distilled water treated non-diabetic animals (Figure 1). Furthermore, the treatment by the extract significantly (p<0.05) increased the organ-body weight ratio but the increase was more significant in the diabetic rats treated with glibenclamide where the values compared well with that of the control animals (Figure 2).
Figure 1: Body weight of alloxan-induced diabetic rats administered aqueous extract of Fadogia agrestis stem
Figure 2: Organ/body weight ratio of alloxan-induced diabetic rats administered aqueous extract of Fadogia agrestis stem
A= Control; B= Diabetic + distilled water; C= Diabetic + 2.5 mg/kg body weight of glibenclamide; D= Diabetic + 18 mg/kg bodyweight of extract; E= Diabetic + 36 mg/kg body weight of extract; F= Diabetic + 72 mg/kg body weight of extract
Administration of alloxan significantly (p<0.05) increased the levels of the serum TC, TG, LDL-C, atherogenic index, VLDL-C and HDL-C to levels that were not significantly different from their respective controls (Table IV). All the doses of the extract significantly (p<0.05) reversed the levels of all these lipids but did not compare well with the distilled water treated non-diabetic animals by the end of the treatment period. Glibenclamide significantly (p<0.05) reversed the concentrations of VLDL-C and HDL-C to levels that were not significantly different from their respective controls (Table IV).
Table IV: Serum lipids of alloxan-induced diabetic rats administered aqueous extract of Fadogia agrestis stem
Control | Diabetic rat treated with | |||||
---|---|---|---|---|---|---|
Distilled water | Glibenclamide | F. agrestis (mg/kg body weight) | ||||
18 | 36 | 72 | ||||
Serum total cholesterol | 2.4 ± 0.0a | 4.6 ± 0.0e | 2.4 ± 0.1a | 4.01 ± 0.0d | 3.5 ± 0.0c | 2.7 ± 0.1b |
Serum triglyceride | 0.4 ± 0.0a | 0.8 ± 0.1c | 0.3 ± 0.0a | 0.8 ± 0.0c | 0.6 ± 0.1b | 0.6 ± 0.1b |
Serum HDL-C | 1.3 ± 0.0ab | 0.8 ± 0.0d | 1.4 ± 0.0a | 0.8 ± 0.0d | 1.0 ± 0.1c | 1.3 ± 0.0b |
Serum LDL-C | 0.9 ± 0.0a | 3.4 ± 0.1e | 0.9 ± 0.1a | 2.9 ± 0.0d | 2.3 ± 0.0c | 1.2 ± 0.1b |
Atherogenic index (LDL-C/HDL-C | 0.7 ± 0.0a | 4.5 ± 0.3d | 0.6 ± 0.0a | 3.7 ± 0.2d | 2.3 ± 0.1c | 1.0 ± 0.01b |
VLDL | 0.2 ± 0.0a | 0.4 ± 0.0c | 0.2 ± 0.0a | 0.4 ± 0.0c | 0.3 ± 0.0b | 0.3 ± 0.0b |
Data are mean ± SEM of seven determinations. Test values with superscripts b, c, d and e different from the control, a, for each parameter are significantly different (p<0.05) |
Discussion
Plants have been an exemplary source of drugs and many currently available drugs have been directly or indirectly obtained from medicinal plants/herbs (Yakubu et al., 2010). Several studies have revealed that a wide variety of plant extracts effectively lowered the glucose level in alloxan-induced diabetic animals (Yakubu et al., 2010; Ananda et al., 2012; Alamgeer et al., 2013; Nagappa et al., 2003). In this present study, the aqueous extract of F. agrestis exhibited the potentials of being used to reduce diabetes and its complications.
Alloxan has been reported to cause massive selective destruction and reduction of beta-cells of the islets of Langerhans mediated by the formation of reactive oxygen species (Szkudelski, 2001), resulting in partial or complete loss of insulin synthesis and leading to the development of hyperglycemia (Nagappa et al., 2003). The resultant hyperglycemia may be due to induced gluconeogenesis in the absence of insulin (Yao et al., 2006). Glibenclamide, a known antidiabetic drug lowers blood glucose by various mechanisms such as binding to ATP-sensitive potassium channel, supperssing hepatic glucose production, increasing insulin sensitivity of extrapancreatic tissues and fatty acid oxidation. Others include enhancing peripheral glucose uptake, decreasing hepatic glycogeneolysis and glucogenesis as well as absorbing glucose from the gastrointestinal tract (Wadkar et al., 2008).
Fasting blood sugar in diabetic rats represents an important indicator of diabetic status. The reduction in blood and serum glucose (both used for better comparism of glucose level) by the doses of the extract might be associated with the prevention of death or restoration of the partially destroyed pancreatic β-cells or the increased uptake of glucose (Noor et al., 2008). It may also be due to increased glycolysis as evidenced by the increased liver hexokinase activity in this study. The probable mechanism of glucose-lowering action of the extract can be linked to the restoration of partially destroyed pancreatic β-cells or increased uptake of glucose via enhanced glycolysis (Noor et al., 2008) or by other mechanism such as slowing down the absorption of sugar from the guts, increasing insulin production by the pancreas from possibly regenerated β-cells, decreasing the release of glucose from or increasing glucose uptake by fat and muscle cells (Yakubu et al., 2010).
Glycogen and glucose are the two forms of sugar employed by the body as a means of storing and providing energy at the cellular level. The liver secretes glucose into the bloodstream as an essential mechanism to keep blood glucose levels constant. Glycogen is the primary intracellular storable form of glucose in various tissues and its level in such tissues especially the liver is a direct reflection of insulin activity (Sharma et al., 2010). Hepatic glycogen level is always depleted in diabetic rats (Sharma et al., 2010). The resultant depletion of liver glycogen stores in diabetic rats by alloxan in the present study could be due to inhibition of glycogen synthase activating system and or stimulation of glycogen phosphorylase activity or both (Sarabu and Tilley, 2005). Similarly, increased hepatic glucose production in diabetes mellitus is associated with impaired suppression of the gluconeogenic enzyme, fructose-1,6-bisphosphatase. The ability of the of the extract to reduce the elevated hepatic glucose level may be a consequence of an effect on glycogen phosphorylase activity or an increase in insulin that enhances glycogenesis; which is consistent with previous studies (Abdel-Moneim et al., 1997; Sharma et al., 2010). The reversed reduction of liver glycogen by the extract suggest that the compounds contained in the plant preparation could effectively attenuate the altered level of glycogen in the diabetic animals probably by promoting the synthesis of glycogen through modulatory effect on the activities of glycogen synthase and glycogen phosphorylase.
In experimental diabetes, enzymes of glucose metabolism are markedly altered (Ananda et al., 2012). Persistent hyperglycemia is a major contributor to such metabolic alterations that lead to the pathogenesis of diabetic complications especially neuropathy and micro vascular diseases (Ananda et al., 2012). One of the key enzymes in the catabolism of glucose is hexokinase, which phosphorylates glucose and converts it into glucose-6-phosphate (Pari and Rajarajeswari, 2009). The increased activity of hexokinase by the extract can enhance utilization of glucose for energy production. Furthermore, the decrease in the concentration of blood glucose of diabetic rats treated with the extract from this study suggests enhanced glycolysis as evidenced in the increased liver hexokinase activity in the present study.
Alloxan-induced diabetes is characterized by severe loss of weight (Ananda et al., 2012). The decrease in the body weight of diabetic rats suggests the loss or degradation of structural protein due to diabetes since proteins contribute significantly to the body weight. The ability of the extract to reverse the body weight loss could be attributed to its potential to reduce hyperglycemia (Ananda et al., 2012). This is an indication that the extract aided in amelioration of weight disorder associated with diabetes. The decrease in the organ-body weight ratio caused by alloxan in this study may be due to atrophy which is one of the consequences of diabetic condition (Szkudelski, 2001). Specifically, the reduction in the weight of the pancreas may be due to decreased secretory granules which is similar to the findings of Kumar et al. (2006) that reported a decrease in the number of secretory granules of the β-cells of the pancreas in diabetic rats. The ability of the aqueous extract of F. agrestis stem to reverse the decreased organ/body weight ratio of the diabetic experimental animals further corroborate the positive influence on weight loss normally associated with diabetes (Yakubu et al., 2010).
The characteristic features of diabetic dyslipidemia are high serum cholesterol, triglyceride (hypertriglycerolemia), LDL-cholesterol concentrations and low HDL-cholesterol (hypercholesterolemia) contents (Mooradian, 2009). Also, the high levels of LDL-C and atherogenic index by the alloxan suggests predisposition to cardiovascular risk (Teeme et al., 2002). The significant increase in cholesterol, triacylglycerol and LDL-cholesterol concentrations in the diabetic rats treated with distilled water may be due to increased mobilization of free fatty acids from the peripheral fat depots. This marked hyperlipidaemia that characterize the diabetic state may therefore be regarded as a consequence of uninhibited actions of lipolytic hormones on the fat depots (Goodman and Gilman, 1985). However, the progressive reduction in hyperlipidemic condition in all the diabetic animals treated with F. agrestis extract further corroborate the ability of the extract to attenuate deranged lipid metabolism normally associated with diabetes mellitus. The attenuation by the extract may be due to activation of lipases that are essential for the hydrolysis of lipids (Oyedemi et al., 2011). Although, the levels of insulin was not determined in the present study, the antihyperlipidemic mechanism of action of the plant extract may involve insulin like effect (Khusbaktova et al., 2005) mediated through the enhancement of lipolytic activity by inhibiting hormone–sensitive lipase or lipogenic enzymes, and/or activating lipoprotein lipase (Aly et al., 2010).
Hypercreatininemia, hypoalbuminemia and hyperuremia have been reported to occur in alloxan-induced diabetic rats (Yakubu et al., 2010), and such elevated levels of biomolecules in the present study did not only suggest disturbance in the metabolism of these substances, but also agree with other previously published works. The restoration of these biomolecules by the extract towards the control levels further corroborate the earlier position of recovery of the animals from some metabolic disorders associated with diabetes by the aqueous extract of F. agrestis stem. Furthermore, the decrease in the total hemoglobin in the diabetic animals treated with distilled water may be a consequence of reaction between excess glucose in the blood of diabetic animals with hemoglobin to form glycosylated hemoglobin (Sheela and Augusti, 1992). Reduction in hemoglobin may be accompanied by a fall in the red blood cell count and packed cell volume (Moss, 1999; Muhammad and Oloyede, 2009). The findings in the present study are similar to that reported elsewhere (Mansi, 2006). The ability of the extract to increase the levels of hemoglobin and PCV in the diabetic animals suggests that aqueous extract of F. agrestis stem has ameliorating activity on the haematological disorders arising from diabetes.
Conclusion
Overall, aqueous extract of Fadogia agrestis stem holds a promise as an antidiabetic agent and can also be used to manage complications arising from diabetes.
References
Abdel-Moneim A, El-Feki M, Salh E. Effect of Nigella sativa, fish and glicazide on alloxan diabetic rats. 1-Biochemical and histopathological studies. J Egyptian German Soc. 1997; 23: 237-65.
Alamgeer, Rashid M, Bashir S, Mushtaq MN, Khan HU, Malik MNH, Qayyum A, Muhammad Rahaman MSU. Comparative hypoglycemic activity of different extracts of Teucrium stocksianum in diabetic rabbits. Bangladesh J Pharmacol. 2013; 8: 186-93.
Aly F, Fatania H, Shamte U. The effect of a plants mixture extract on liver gluconeogenesis in streptozotocin induced diabetic rats. Diabetes Res Clin Exp. 2010; 18: 163-68.
Ameen OM, Olatunji G, Atata RF, Usman LA. Antimicrobial activity, cytotoxic test and phytochemical screening of extracts of the stem of Fadogia agrestis. Nigerian Soc Exptal Biol J. 2011; 11: 79-84.
Ananda PK, Kumarappan CT, Sunil C, Kalaichelvan VK. Effect of Biophytum sensitivum on streptozotocin and nicotinamide-induced diabetic rats. Asian Pac J Tropical Biomed. 2012; 11: 31-35.
Anero RI, IXaz-Lanza E, Ollivier B, Baghdikian G, Balansard MB. Monoterpene glycosides isolated from Fadogia agrestis. Phytochem. 2008; 69: 805-11.
Aruna RV, Ramesh B, Kartha VN. Effect of beta-carotene on protein glycosylation in alloxan induced diabetic rats. Indian J Exp Biol. 1999; 37: 399-401.
Barham D, Trinder P. An improved colour reagent for the determination of blood glucose by oxidase system. Analyst 1972; 97: 142-45.
Brandstrup N, Kirk JE, Bruni C. Determination of hexokinase in tissues. J Gerontol. 1957; 12: 166-71.
Dey L, Xie JT, Wang A, Wu J, Maleckar SA, Yuan CS. Antihyperglycemic effects of ginseng: Comparison between root and berry. Phytomedicine 2003; 10: 600-05.
Fredrickson DS, Levy RI, Lees RS. Fat transport in lipoproteins: An integrated approach to mechanisms and disorders. New Engl J Med. 1967; 276: 148-56.
Friedewald WT, Levy RI, Fedrickson DS. Estimation of the concentration of low-density lipoprotein cholesterol in plasma, without use of the preparative ultracentrifuge. Clin Chem. 1972; 18: 499-502.
Goodman LS, Gilman A. The pharmacological basis of therapeutics. 7th ed. New York, MacMillan, 1985, pp 1490-510.
Grover JK, Yadav S, Vats V. Medicinal plants of India with antidiabetic potential. J Ethnopharmacol. 2002; 81: 81-100.
Gupta R, Gabrielsen B, Ferguson FM. Nature’s Medicines: Traditional knowledge and intellectual property management. Case studies from the National Institutes of Health (NIH). USA. Curr Drug Discov Technol, 2005; 2: 203-19.
Hainline AJR, Cooper GR, Olansky AS. CDC Survey of high density lipoprotein cholesterol measurement: A report. Atlanta Lipid Standardization Program. Center for Disease Control, 1980, p 3.
Kemp A, Kits Van Heijningen AJM. A colorimeter micro-method for the determination of glycogen in tissues. Biochem J. 1954; 6: 646-48.
Khushbaktova KM, Ozsoy-Sacan O, Bolkent S, Yanardag R. Effect of glurenorm on immunohistochemical changes in pancreatic β-cells of rats in experimental diabetes. Indian J Exp Biol. 2005; 43: 268-71.
Kumar GPS, Arulselvan P, Kumar DS, Subramanian SP. Antidiabetic activity of fruits of Terminalia chebula on streptozotocin-induced diabetic rats. J Health Sci. 2006; 52: 283-91
Mansi KMS. Effects of administration of alpha-melanocyte stimulating hormone (α-MSH) on some hematological values of alloxan-induced diabetic rats. Am J Pharmacol Toxicol. 2006; 1: 5-10.
Mooradian AD. Dyslipidemia in type 2 diabetes mellitus. Nature Clin Practice Endocrinol Metab. 2009; 5: 150-59.
Moss PP. Blood banking: Concepts and applications. Philadelphia, W.B. Saunders Co., 1999, pp 12-34.
Muhammad NO, Oloyede OB. Haematological parameters of broiler chicks fed Aspergillus niger - fermented Terminalia catappa seed meal-based diet. Global J Biotechnol Biochem. 2009; 4: 179-83.
Nagappa AN, Thakurdesai PA, Venkat RN, Singh J. Antidiabetic activity of Terminalia catappa Linn fruits. J Ethnopharmacol. 2003; 88: 45-50.
Ng TKW, The CB, Vidyadaran MK, Tee ES, Thong ML, Kandiah M, Ehalid AH. A critical evaluation of high density lipoprotein cholesterol as an index of coronary artery disease risk in Malaysians. Malaysian J Nutr. 1997; 3: 61-70.
Noor A, Gunasekaran S, Manickam AS, Vijayalakshmi MA. Antidiabetic activity of Aloe vera and histology of organs in streptozotocin-induced diabetic rats. Curr Sci. 2008; 94: 1070-76.
Oyedemi SO, Yakubu MT, Afolayan AJ. Antidiabetic activities of aqueous leaves extract of Leonotis leonurus in streptozotocin induced diabetic rats. J Med Plants Res. 2011; 5: 119-25.
Pari L, Rajarajeswari N. Efficacy of coumarin on hepatic key enzymes of glucose metabolism in chemical induced type 2 diabetic rats. Chem Biol Interaction. 2009; 181: 292-96.
Pattanayak S, Nayak SS, Panda D, Shende V. Hypoglycemic of Cajanus scarabaeoides in glucose overloaded and streptozotocin-induced diabetic rats. Bangladesh J Pharmacol. 2009; 4: 131-35.
Saha BK, Bhuiyan NH, Mazumder K, Haque KMF. Hypoglycemic activity of Lagerstroemia speciosa L. extract on streptozotocin-induced diabetic rat: Underlying mechanism of action. Bangladesh J Pharmacol 2009; 4: 79-83.
Sanon S, Olliver E, Azas N, Mahiou V, Gassquet M, Quattara CT, Nebie I, Traore AS, Esposito F, Balansard G, Timon-David P, Fumoux F. Ethnobotanical survey and in vitro antiplasmodial activity of plant used in traditional medicine in Burkina Faso. J Ethnopharmacol. 2003; 86: 143-47.
Sarabu R, Tilley J. Recent advances in therapeutic approaches to type 2 diabetes. In: Annual reports in medicinal chemistry. Doherty AM (ed). Elsevier, Academic Press, 2005; 40: 167-81.
Shalm OW, Jain NC, Carroll EJ. Veterinary haematology. 3rd ed. Philadelphia, Lea and Fabiger, 1995, pp 498-512.
Sharma N, Garg V, Paul A. Antihyperglycemic, antihyperlipidemic and antioxidative potential of Prosopis cineraria bark. Indian J Clin Biochem. 2010; 25: 193-200.
Sheela CG, Augusti KT. Antidiabetic effects of S-allyl cysteine sulphoxide isolated from garlic Allium sativum Linn. Indian J Exp Biol. 1992; 30: 523-26.
Szkudelski T. The mechanism of alloxan and streptozotocin action on β-cells of the rat pancreas. Physiol Res. 2001; 50: 536-46.
Temme E, Van Hoydonck PG, Schouten EG, Kesteloot H. Effect of plant sterol-enriched spread on serum lipids and lipoproteins in mildly hypercholesterolaemic subjects. Acta Cardiol. 2002; 57: 111-15.
Tietz NW. Clinical guide to laboratory tests. 3rd ed. Philadelphia, W. B. Saunders Co., 1995.
Wadkar KA, Magdun CS, Patil SS, Naikwade NS. Antidiabetic potential and Indian medicinal plants. J Herbal Med Toxicol. 2008; 2: 45-50.
Yakubu MT, Akanji MA, Oladiji AT. Aphrodisiac potentials of the aqueous extract of Fadogia agrestis (Schweinf. Ex Heirn) stem in male albino rats. Asian J Androl. 2005; 7: 399-404.
Yakubu MT, Akanji MA, Oladiji AT. Alterations in serum lipid profile of male rats by oral administration of aqueous extract of Fadogia agrestis stem. Res J Med Plant. 2008; 2: 66-73.
Yakubu MT, Akanji MA, Nafiu MO. Antidiabetic activity of aqueous of Cochlospermum planchonii root in alloxan-induced diabetic. Cameroon J Exp Biol. 2010; 6: 91-100.
Yao XH, Chen L, Nyomba BL. Adult rats prenatally exposed to ethanol have increased gluconeogenesis and impaired insulin response of hepatic gluconeogenic genes. J Appl Physiol. 2006; 100: 642-48.
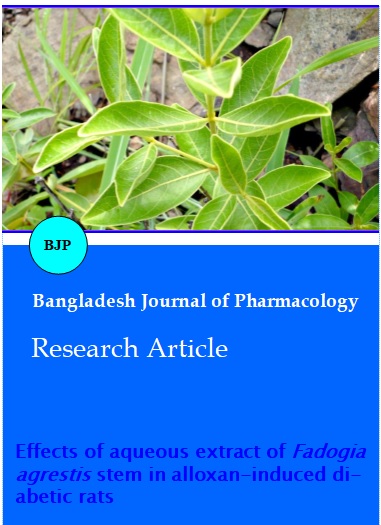