Design, docking, synthesis and anticancer activity of some novel 2-(4-methylbenzenesulphonamido)pentanedioic acid amide derivatives
Abstract
In the present work few novel 2-(4-methylbenzenesulphonamido)pentanedioic acid amide derivatives and the basic compound 2-(4-methylphenylsulfonamido)pentanedioic acid have been designed, synthesized, characterized and screened for their possible antineoplastic activity both in vitro and in vivo. The modified drugs were docked against the protein histone deacetylase the energy value obtained was o-iodoanilide (-10.370504) and m-iodoanilide (-10.218276) of the titled compound. The in vitro activity was performed against five human cell lines like human breast cancer (MCF-7), leukemia (K-562), ovarian cancer (OVACAR-3), human colon adenocarcinoma (HT-29) and human kidney carcinoma (A-498). The in vivo activity was performed in female Swiss albino mice against Ehrlich Ascites Carcinoma (EAC). Among the synthesized compounds, o-iodoanilide, m-iodoanilide and p-iodoanilide derivatives of 2-(4-methyl benzene sulphonyl)-pentanedioic acid amides showed encouraging activity in both the in vitro and in vivo compared to other compounds.
Introduction
Glutamic acid (2-amino pentanedioic acid) plays an important role in the biosynthesis of purine and pyrimidine bases of DNA and RNA (Rodwell, 2000). Antagonists of L-glutamine synthetase can interfere with the metabolic role of L-glutamine and act as anticancer agents (Hartman, 1970). Azaserine and 6-diaza-5-oxo-L-norleucine antagonized the metabolic process involving L-glutamine and exhibited antitumor activity in animal models (Eidinoff, 1958). All tumor cells studied were found to have a high activity of phosphate-dependent glutaminase utilizing glutamine from the medium during long-term culture (Graff et al., 1940). Human hepatoma cells take up glutamine at rates several fold faster than the normal human hepatocytes (Petit, 1977). Beside glucose, glutamine is assumed to be the main energy source in tumor cells (Keren and Stark, 1998) and plays a central role in multiple metabolic pathways and considered to be the most essential component of tissue/cell culture media for not only as the nitrogen source but also as the carbon source (Debnath et al., 2002; Sugita, 1995). After a definite time interval, all cells start mutation in cell culture medium, which is also indicative for the role of glutamine in cancer (Rosowsky et al., 1979). Thus, the structural variants of glutamine attracted our attention to develop possible anticancer agents, which may act through glutamine and/or folic acid antagonism.
Computational biology and bioinformatics have the potential not only of speeding up the drug discovery process thus reducing the costs, but also of changing the way drugs are designed. Rational drug design helps to facilitate and speedup the drug designing process, which involves variety of methods to identify novel compounds. One such method is the docking of the drug molecule with the receptor (target). Docking is the process by which two molecules fit together in 3D space (Baskaran and Ramachandran, 2012).
Materials and Methods
Commercially available reagents and starting materials for the synthesis were obtained from E. Merck, India, CDH, s.d. Fine Chem, India and Qualigens, India. Silica gel G used for TLC was obtained from E. Merck. The reaction was monitored by TLC using on 0.25 mm E. Merck silica gel 60F252 precoated plates, which were visualized under UV light. Melting points were determined in an open glass capillary using a Kjeldahl flask containing paraffin and are uncorrected. The proton and carbon magnetic resonance spectra (1H NMR, 13C NMR) were recorded on a Bruker 400 MHz instrument (Bruker, Germany) in dimethyl sulfoxide-d6 (DMSO-d6) using tetramethylsilane as internal standard. Chemical shifts (d) are expressed in ppm and coupling constants (s) singlet, (d) doublet, (t) triplet, (m) multiplet. Position of carbons described in 13C NMR interpretation is as per general structure (Figure 1) for all the compounds. The infrared spectra of compounds were recorded in KBr on Fourier Transform (FTIR-8400S, Shimadzu, Japan) infrared spectrophotometer. Mass spectra (FAB) were recorded on LC-MS/MS (API-4000 TM, Applied BioSystems, MDS SCIEX, Canada). Elemental analyses were performed on a Perkin-Elmer model 240c analyzer (Perkin Elmer, USA). For docking study we used biological databases like PubChem, Drug Bank, PDB (Protein Data Bank) and software’s like Arguslab, Weblab viewer lite program, molinspiration, FROG ADME Tox.
Figure 1: General structure for 2-(4-methylbenzenesulphonamido)pentanedioic acid amides
Docking methodology
Bioinformatics is seen as an emerging field with the potential to significantly improve how drugs are found, brought to the clinical trials and eventually released to the marketplace. Computer-Aided Drug Design (CADD) is a specialized discipline that uses computational methods to simulate drug-receptor interactions. CADD methods are heavily dependent on bioinformatics tools, applications and databases (David et al., 2006).
Protein preparation and optimization
The crystal structure of histone deacetylase (HDAC) taken in this study was retrieved from RCSB protein databank. The missing residues were corrected and the complexes bound to receptor molecule removed using Accelrys Discovery Studio Visualizer 2.5.5. The PDB files were energy minimized using ArgusLab. The non-essential water molecules were removed and polar hydrogens were merged.
Ligand preparation and optimization
Using Chemsketch Software the structures of the drugs and analogs were sketched draw and generated their MOL File followed subsequent generation of their 3D structures by using tool Weblab viewer lite program a molecule format converter in to PDB. Appropriate force field applied to them and then optimization was carried out using Argus Lab 4.0.
Docking simulations
The docking analysis of glutamic acid analogs, histone deacetylase protein and all the analogs with histone deacetylase protein was carried by Argus lab docking software. Docking allows the scientist to virtually screen a database of compounds and predict the strongest binders based on various scoring functions. It explores ways in which two molecules, such as drugs and histone deacetylase protein fit together and docks to each other well. The molecules binding to a receptor inhibit its function and thus act as drug (Srivastava et al., 2008). The collection of glutamic acid analogs and receptor complexes were identified via docking and their relative stabilities were evaluated using molecular dynamics and their binding affinities, using free energy simulations. All the parameters used for Arguslab docking are selected by default.
General procedure for synthesis of 2-(4-methylbenzene sulfonamido)pentanedioic acid amide derivatives (5a-e): 2-(4-Methylbenzenesulfonamido)pentanedioic acid amide derivatives were synthesized based on following procedure.
Synthesis of 4-methylbenzene-1-sulfonyl chloride (2): Toluene (9.2 mL, 0.1 mole) was taken in a 250 mL three necked round bottom flask placed on an ice bath fitted with a mercury sealed mechanical stirrer, a calcium chloride guard tube and a 100 mL dropping funnel. Chlorosulfonic acid (25 mL, 3 equiv., 0.36 mole) was placed in the dropping funnel and was added drop wise slowly by continuous stirring. The temperature of the ice bath was maintained at 0°C. After the addition of the chlorosulfonic acid completely the mixture was allowed to come to room temperature. Then the product was poured slowly into well stirred crushed ice. The crude 4-methylbenzene-1-sulfonyl chloride (2) was filtered and washed with water to remove the excess acid. The crude product was recrystalized from water. The yield was 15.20 g (84.21%) and m.p.: 155-157°C.
Synthesis of 2-(4-methylbenzenesulfonamido)pentanedioic acid (3): This was prepared form 4-methylbenzene-1-sulfonyl chloride (2) and L-(+)-glutamic acid according to the procedure reported below.
L-(+)-glutamic acid (20 g, 0.13 mole) was taken in a 250 ml conical flask and placed on a water both, fitted with a magnetic stirrer. Sodium hydroxide solution (2N) was added slowly till all the L-glutamic acid dissolved and the mass became distinctly alkaline to phenolphthalein. The water bath was maintained at 60-80°C and 4-methylbenzene-1-sulfonyl chloride (2; 36.10 g, 0.2 mole) was added slowly with continuous stirring and simultaneous addition of sodium hydroxide solution (2N) to keep the mass alkaline. The reaction was continued till a clear homogeneous solution results. After the reaction was over, it was allowed to cool, acidified to congored with concentrated hydrochloric acid, saturated with sodium chloride, extracted with chloroform, and allowed to dry overnight with anhydrous magnesium sulfate. Chloroform was removed to yield the crude 2-(4-methylbenzenesulfonamido)pentanedioic acid (3). The crude product was recrystalized from hot water after charcoal treatment. Yield 57.89%, m.p.: 150-153°C. Rf 0.76. Neutral equivalent (found: 297.20, Calc. For C12H15NO6S: 301). IR (KBr) vmax (cm-1) 3136.95 (C-H str. of phenyl ring), 3059.14-2620.21 (O-H str. of COOH), 1667.16, (C=O str.), 1502.34 (C=C str. of phenyl ring), 1322.65 (C-O str. or O-H def of COOH), 1381.06 (S=O str. antisym of SO2N), 1267.80 (S=O str. sym of SO2N), 825 (out of plane C-H def due to p-subs in phenyl ring), 3294.93 (N-H str.), 1664.16 (N-H bending). 1H NMR (400 MHz, DMSO-d6) δ = 7.519 (d, 2H, 2', 6' H of C6H4-CH3), 7.071 (d, 2H, 3', 5' H of C6H4-CH3), 3.7 (t, 3H, -OC-C.H-CH2-), 2.152 (s, 1H, -CH3), 2.14 (s, 1H, -SO2NH-). 13C NMR (DMSO-d6) δ = 129.12 (C-1 & 5), 118.14 (C-2 & 4), 143.76 (C-3), 168.32 (C-8), 176.20 (C-18). MS (FAB; m/z): 301. [M+1]. Elemental analysis (C12H15NO6S); calcd. C, 47.83; H, 5.02; N, 4.65; found C, 47.70; H, 4.91; N, 4.59%.
Synthesis of 1((4-methylbenzene)sulfonyl)-5-oxopyrrolidine-2-caroboxylic acid (4): 2-(4-Methylbenzenesulfonamido) pentanedioic acid (3; 32 g, 0.113 mole) was taken in a 500 mL round bottom flask, fitted with a reflux condenser and a calcium chloride guard tube. Acetyl chloride (70 mL) and benzene (40 mL) were added to it and refluxed in a steam bath for four hours. After refluxing, benzene was removed by distillation and the reaction mass was cooled. After that chopped ice poured in the round bottom flask with continuous stirring. It was kept overnight in freeze, when the semisolid mass solidified. It was filtered, washed with distilled water. The crude product was recrystallized from dilute ethanol after charcoal treatment. This was later used in the subsequent steps. Yield: 2.80 g (82.84%); m.p.: 121-123°C.
Synthesis of 2-(4-methylbenzenesulfonamido)pentanedioic acid amide derivatives (5a-e): The 1((4-methylbenzene)sulfonyl)-5-oxopyrrolidine-2-caroboxylic acid (4) form-ed was dissolved in dry benzene (10 mL) and the whole mass was cooled by dipping in ice water. Aniline and its derivatives, previously cooled was added to it and mixed well. The whole mass was transferred in a mortar and pestle and triturated when the product was obtained. It was then acidified with dilute hydrochloric acid. The precipitate obtained was filtered and washed with distilled water to remove excess acid. The residue was dried and recrystallized from dilute ethanol after charcoal treatment.
2-(4-Methylphenylsulfonamido)-5-((2-iodophenyl)amino)-5-oxopentanoic acid (5a): Greyish color solid; yield: 65.75%; m.p.: 202-204°C; Rf 0.87; IR (KBr) vmax (cm-1): 2962.45-2693.74 (O-H str. of COOH), 1670.71 (C=O str.), 1545.31 (C=C str. of phenyl ring), 1317.44 (S=O str. antisym of SO2N), 1232.42 (S=O str. sym of SO2N), 857.15 (out of plane C-H def due to p-subs in phenyl ring), 3374 (N-H str.), 1641 (N-H bending). 1H NMR (DMSO-d6): δ 7.72 (d, 2H, 2', 6' H of C6H4-CH3), 7.541 (d, 2H, 3', 5' H of C6H4-CH3), 8.03 (s, 1H, 6' H of C6H4-I), 8.014 (d, 2H, 3', 5' H of C6H4-I), 3.72 (t, 3H, -OC-C.H-CH2-), 2.77 (s, 1H, -CH3), 2.02 (s, 1H, -SO2NH-). 13C NMR (DMSO-d6): δ 141.87 (C-1), 128.48 (C-2 & 6), 128.33 (C-3 & 5), 134.74 (C-4), 57.74 (C-7), 27.15 (C-8), 39.27 (C-9), 122.31 (C-11), 128.77 (C-12 &14), 21.35 (C-18). MS (FAB; m/z): 502. [M+1]. Elemental analysis (C18H19IN2O5S); calcd. C, 43.04; H, 3.81; N, 5.58; found C, 43.13; H, 3.89; N, 5.60%.
2-(4-Methylphenylsulfonamido)-5-((3-iodophenyl)amino)-5-oxopentanoic acid (5b): White color solid; yield: 71.05%; m.p.: 196-198°C; Rf 0.91; IR (KBr) vmax (cm-1): 2957.14-2648.11 (O-H str. of COOH), 1677.17 (C=O str.), 1517.4 (C=C str. of phenyl ring), 1347 (S=O str. antisym of SO2N), 125.2 (S=O str. sym of SO2N), 840.14 (out of plane C-H def due to p-subs in phenyl ring), 741.74 (C-I str. of phenyl ring), 3362 (N-H str.), 1640 (N-H bending). 1H NMR (DMSO-d6): δ 7.457 (d, 2H, 2', 6' H of C6H4-CH3), 7.277 (d, 2H, 3', 5' H of C6H4-CH3), 7.644 (d, 2H, 2', 6' H of C6H4-I), 7.018 (s, 1H, 5' H of C6H4-I), 4.47 (t, 3H, -OC-C.H-CH2-), 2.41 (s, 1H, -CH3), 2.01 (s, 1H, -SO2NH-). 13C NMR (DMSO-d6): δ 140.47 (C-1), 129.44 (C-2 & 6), 128.79 (C-3 & 5), 137.32 (C-4), 57.41 (C-7), 26.15 (C-8), 36.24 (C-9), 120.14 (C-11& 15), 128.7 (C-12), 21.12 (C-18). MS (FAB; m/z): 502. [M+1]. Elemental analysis (C18H19IN2O5S); calcd. C, 43.04; H, 3.81; N, 5.58; found C, 43.07; H, 3.75; N, 5.64%.
2-(4-Methylphenylsulfonamido)-5-((4-iodophenyl)amino)-5-oxopentanoic acid (5c): White colour solid; yield: 68.79%; m.p.: 180-182°C; Rf 0.84; IR (KBr) vmax (cm-1): 2923.42-2551.41 (O-H str. of COOH), 1677 (C=O str.), 1524.21 (C=C str. of phenyl ring), 1342.15 (S=O str. antisym of SO2N), 1217.12 (S=O str. sym of SO2N), 874.21 (out of plane C-H def due to p-subs in phenyl ring), 749.17 (C-I str. of phenyl ring), 3247.35 (N-H str.), 1647 (N-H bending). 1H NMR (400 MHz, DMSO-d6): d 7.424 (d, 2H, 2', 6' H of C6H4-CH3), 7.271 (d, 2H, 3', 5' H of C6H4-CH3), 7.841 (d, 2H, 2', 6' H of C6H4-I), 7.481 (d, 2H, 3', 5' H of C6H4-I), 4.14 (t, 3H, -OC-C.H-CH2-), 2.341 (s, 1H, -CH3), 2.34 (q, 4H,-H2C-H2C-), 2.12 (s, 1H, -SO2NH-). 13C NMR (DMSO-d6): d 140.63 (C-1), 127.78 (C-2 & 6), 127.57 (C-3 & 5), 134.63 (C-4), 57.24 (C-7), 27.07 (C-8), 36.23 (C-9), 121.85 (C-11& 15), 128.97 (C-12 &14), 21.71 (C-18). MS (FAB; m/z): 502. [M+1]. Elemental analysis (C18H19IN2O5S); calcd. C, 43.04; H, 3.81; N, 5.58; found C, 42.92; H, 3.76; N, 5.49%.
2-(4-Methylphenylsulfonamido)-5-((2-methylphenyl)amino)-5-oxopentanoic acid (5d): Yellow color solid; yield: 61.74%; m.p.: 217-219°C; Rf 0.74; IR (KBr) vmax (cm-1): 3054.14-2741.14 (O-H str. of COOH), 1561.12 (C=O str.), 1527.12 (C=C str. of phenyl ring), 1377.5 (S=O str. antisym of SO2N), 1241.17 (S=O str. sym of SO2N), 880.45 (out of plane C-H def due to p-subs in phenyl ring), 1581.47 (C-NO2), 3297.31 (N-H str.), 1617 (N-H bending). 1H NMR (400 MHz, DMSO-d6): d 7.737 (d, 2H, 2', 6' H of C6H4-CH3), 7.412 (d, 2H, 3', 5' H of C6H4-CH3), 7.93 (s, 1H, 6' H of C6H4-Methyl), 7.814 (d, 2H, 3', 5' H of C6H4-Methyl), 3.414 (t, 3H, -OC-C.H-CH2-), 2.43 (s, 1H, -CH3), 2.11 (s, 1H, -SO2NH-). 13C NMR (DMSO-d6): d 140.17 (C-1), 127.13 (C-2 & 6), 128.33 (C-3 & 5), 137.75 (C-4), 57.21 (C-7), 27.30 (C-8), 36.74 (C-9), 121.35 (C-11), 123.83 (C-12 &14), 21.46 (C-18). MS (FAB; m/z): 390. [M+1]. Elemental analysis (C19H22N2O5S); calcd. C, 58.45; H, 5.68; N, 7.17; found C, 58.38; H, 5.62; N, 7.24%.
2-(4-Methylphenylsulfonamido)-5-((3-methylphenyl)amino)-5-oxopentanoic acid (5e): White color solid; yield: 78.24%; m.p.: 271-273°C; Rf 0.85; IR (KBr) vmax (cm-1): 2951.72-2687.24 (O-H str. of COOH), 1641.71 (C=O str.), 1577.44 (C=C str. of phenyl ring), 1319.24 (S=O str. antisym of SO2N), 1212.19 (S=O str. sym of SO2N), 874.24 (out of plane C-H def due to p-subs in phenyl ring), 3373 (N-H str.), 1677.4 (N-H bending). 1H NMR (400 MHz, DMSO-d6): d 7.701 (d, 2H, 2', 6' H of C6H4-CH3), 7.523 (d, 2H, 3', 5' H of C6H4-CH3), 7.211 (d, 2H, 2', 6' H of C6H4-Methyl), 7.11 (d, 2H, 3', 5' H of C6H4-Methyl), 3.52 (t, 3H, -OC-C.H-CH2-), 2.427 (s, 1H, -CH3), 2.17 (s, 1H, -SO2NH-). 13C NMR (DMSO-d6): d 142.61 (C-1), 128.32 (C-2 & 6), 127.68 (C-3 & 5), 137.16 (C-4), 57.74 (C-7), 26.07 (C-8), 36.38 (C-9), 121.72 (C-11& 15), 127.17 (C-12), 21.7 (C-18). MS (FAB; m/z): 390. [M+1]. Elemental analysis (C19H22N2O5S); calcd. C, 58.45; H, 5.68; N, 7.17; found C, 58.52; H, 5.58; N, 7.11%.
In vitro anti-cancer assay
Human breast cancer (MCF-7), leukemia (K-562), ovarian cancer (OVCAR-3), human colon adenocarcinoma (HT-29) and human kidney carcinoma (A-498) tumor cells were obtained from National Centre for Cell Sciences (Pune, India). The cultures were maintained in Dulbecco's Modified Eagles Medium (DMEM) containing 10% heat inactivated fetal bovine serum (FBS), penicillin (100 units/mL) and streptomycin (100 µg/mL) at 37°C in 5% CO2. Cells were grown in 25 cm2 tissue cultures flask until confluent and used for cytotoxicity assays.
MTT assay
The MTT (3-(4,5-dimethylthiazole-2-yl)-2,5 diphenyltetrazolium bromide) assay developed by Mosmann (Mosmann, 1983) was modified and used to determine the inhibitory effects of test compounds on cell growth in vitro. In brief, the trypsinized cells from T-25 flask were seeded in each well of 96-well flat bottomed tissue culture plate at a density of 5 x 103 cells/well in growth medium and cultured at 37°C in 5% CO2 to adhere. After 48 hours incubation, the supernatant was discarded and the cells were pretreated with growth medium and were subsequently mixed with different concentrations of both standard (tamoxifen) and test compounds 5a-e (8, 16, 32, 64, 128 and 256 µg/mL) in triplicates to achieve a final volume of 100 µL and then cultured for 48 hours. The compound was prepared as 1.0 mg/mL concentration stock solutions in PBS. Culture medium and solvent are used as controls. Each well then received 5 µL of fresh MTT (0.5 mg/mL in PBS) followed by incubation for 2 hours at 37°C. The supernatant growth medium was removed from the wells and replaced with 100 µL of DMSO to solubilize the colored formazan product. After 30 min incubation, the absorbance (OD) of the culture plate was read at a wavelength of 570 nm on an ELISA reader, Anthos 2020 spectrophotometer. Both standard and test maintained in triplicate. The IC50 value refers to the drug concentration that produces a 50% reduction in cellular growth when compared to untreated control cells (Holbeck, 2004).
In vivo anticancer assay
The animal experiments were performed following the approval of study protocols by the Institutional Animal Ethics Committee (BCRCP/IAEC/7/2012). The synthesized compounds were biologically screened against Ehrlich ascites carcinoma (EAC) in female Swiss albino mice using tumor weight and cell count as activity parameters. Amongst various evaluation systems in vogue, this method has been standardized and numbers of screening results have been reported earlier (De and Pal, 1975; De and Pal, 1977; Ray and De, 2009). Two groups of mice, each containing five healthy mice of the same sex (female in this case), approximately of same age and body weight (18-20 g), were selected at random and kept in two different cages under identical condition. One of these two groups served as control while the other as test. Ehrlich Ascites Carcinoma (EAC) cells were collected from the donor mice and were suspended in sterile isotonic solution (0.9% w/v NaCl). The numbers of tumor cells per mL of this suspension were counted under microscope with the help of hemocytometer. A definite number (about 2 x 106/0.2 mL) of these living viable cells was injected or implanted into the peritoneal cavity of each mouse. In this instance, the tumor cells multiplied relatively freely with in the peritoneal cavity and ascites developed. A day of incubation was allowed to establish the disease in the body before the administration of synthesized compounds. From the second day of transplantation up to the eight day a suitable dose (0.2 mmol/kg body weight) of the drug solution/suspension in sterile phosphate buffer (pH 7.2) was injected intraperitoneally to each mouse in the test group at 24 hours interval. Thus, seven doses of the drug were administered to each mouse in the test group. On the ninth day food and water were withheld or withdrawn 6 hours before the testing operation started. The weight of all the animals was recorded before they were sacrificed. The peritoneal cavity was dissected and the ascites fluid was drawn by a syringe to a suitable volume with sterile ice cold saline and preserved in ice bath. The total number of living cells per mL in the peritoneal fluid of the three mice in a group was calculated. The fluid was sucked by absorbent cotton. The weight of the five mice after sacrifice was recorded. The evaluation of the test drug was made by comparing the cell count and ascetic fluid weight of the test with that of the control. The percentage inhibition of ascetic cell count and ascetic fluid weight was obtained by the following expressions.
Percentage inhibition of ascitic cell = (1 - T/C) x 100
Percentage inhibition of ascitic fluid = (1 - T/C) x 100
Where, T= Average No. of ascitic cells per mL in test animals, C= Average no. of ascitic cells per mL in control animals, T = Average weight of ascitic fluid in test animals and C = Average weight of ascitic fluid in control animals
Mitomycin-C (1 mg/kg body weight) in sterile phosphate buffer (pH 7.2) was used as standard.
Result and Discussion
Docking results tabulated between histone deacetylase protein and the five better conventional glutamic acid analogs (Table I) as well as with the structure of protein and modified analogs are shown in Figure 2 and 3. Based on the literature it has been shown clearly that glutamic acid analogs have been used to target the histone deacetylase protein. Out of 90 glutamic acid analogs on docking with histone deacetylase protein produced an energy value ranges from -10.370504 to -0.421512. In all glutamic acid analogs, it was observed using RasMol that the amide groups present in the analogs was the site of binding to the receptor and methyl group present in the analogs, which resulted in a decrease in the energy values. These modifications were made using Chemsketch and the energy values were calculated using Arguslab. This way the pharmacophoric part of the drug was partially identified. Docking results of the drug and its derivatives via Arguslab docking software reveals that the e-value of glutamic acid compound 5a (-10.370504) is better as compared to that of the other glutamic acid analogs. All the analogs were prepared virtually using Chemsketch. This particular compound 5b and 5c showed an increase in the energy values (-10.218276 and -9.126992) which means these analogs were more compatible with the receptor and required less energy to binding with the receptor. However, the binding site of the analog was similar to that of its other analogs, which means that functional groups involved were the same and by preparing the analog only the steric compatibility was increased.
Table I: Docking results of histone deacetylase receptor with glutamic acid analogs
Compound | Docking score | Glide score | Glide metal | Glide E-model | Glide energy |
---|---|---|---|---|---|
5a | -10.4 | -10.4 | -2.3 | -73.1 | -54.6 |
5b | -10.2 | -10.2 | -2.3 | -45.2 | -48.2 |
5c | -9.1 | -9.1 | -2.3 | -55.4 | -45.4 |
5d | -8.3 | -8.3 | -2.3 | -28.4 | -16.8 |
5e | -8.1 | -8.1 | -2.3 | -70.6 | -52.6 |
Figure 2: Structure of histone deacetylase protein
Figure 3: Docking of compound 5a with histone deacetylase protein
The synthetic strategies followed for the preparation of the substituted 2-(4 methylbenzenesulfonamido)pentanedioic acid amide derivatives 5a-e are depicted in Scheme 1. Synthesis was started with chlorosulphonation of toluene, to get 4-methylbenzene-1-sulfonyl chloride 2. This sulphonyl halide proved to be versatile synthon in the subsequent step in the preparation of substituted sulphonyl glutamic acids. With the application of Schotton-Bauman reaction, substituted sulphonyl glutamic acids 3 were prepared by one-step condensation of 4-methylbenzene-1-sulfonyl chloride 2 with L-glutamic acid. In this reaction alkaline medium was maintained to remove the hydrochloric acid which was formed during condensation. Reaction of the resulting intermediate sulphonyl glutamic acids 3 with acetyl chloride afforded cyclized acid intermediates substituted sulphonyloxopyrrolidine carboxylic acids 4. Aminolysis of the intermediates 4 with various amines afforded the corresponding glutamines 5a-e.
Scheme 1: Synthesis of 2-(4-methylbenzenesulfonamido)pentanedioic acid amide derivatives
Formation of 2-(4-methylbenzenesulphonamido)pentanedioic acid amide derivatives 5a-e were confirmed by recording their IR, NMR, mass spectra and elemental analyses. The IR spectra of compounds 5a-e revealed the presence of absorption bands from 3012.54 to 2542 cm-1 that indicate the presence of OH str. of COOH, from 1696.07 to 1570.8 cm-1 for C=O str., from 1507 to 1577.31 cm-1 for C=C str. of phenyl ring, from 3287.32 to 3397 cm-1 for N-H str., from 1593-1645 cm-1 for N-H bending, from 1280.84-1354.5 cm-1 for S=O str. antisymmetric of SO2N and 1209-1310.18 cm-1 for S=O str. symmetric of SO2N vibrations. In addition to proton signals of the functional groups and both aromatic ring present in the respective molecule 1H NMR spectra of these compounds contained one proton singlet from d 2.0 to 2.203 ppm which was assigned to -SO2NH- proton and from 2.21 to 2.604 ppm for -CH3 proton. The 1H NMR spectra of compounds 5a-e showed doublets from d 7.201 to 7.98 ppm for aromatic protons and triplets from d 3.54 to 4.1 ppm for -OC-CH-CH2- proton confirming the formation of compounds 5a-e. The 13C NMR spectra of compounds 5a-e showed peaks from d 118.57 ppm to 156.38 ppm for aromatic protons, from δ 20.12 ppm to 22.31 ppm for -CH3 carbon confirming the formation of compounds 5a-e. The mass spectra of compounds 5a-e showed molecular ion peaks [M+1] at m/z corresponding to their respective molecular masses, which is in consistency with their respective molecular formulas. For the compound 5a, molecular weight 502 is consistent with the molecular formula C18H19IN2O5S. The values for the remaining compounds have been presented under the experimental part.
In vitro anticancer assay
The cytotoxicity of the synthesized compounds 5a-e and the intermediate compound No. 3 were studied using the MTT assay in five human cancer cell lines, including human breast cancer (MCF-7), leukemia (K-562), ovarian cancer (OVCAR-3), human colon adenocarcinoma (HT-29) and human kidney carcinoma (A-498). The results are listed in Table II. Compounds 2-(4-methylphenylsulfonamido)-5-((2-methylphenyl)amino)-5-oxopentanoic acid 5d and 2-(4-methylphenylsulfona-mido)-5-((3-methylphenyl)amino)-5-oxopentanoic acid 5e showed low cytotoxic effects on all the cell lines. Compounds 2-(4- methyl-phenylsulfonamido)pentanedioic acid 3, 2-(4-methyl-phenylsulfonamido)-5-((2-iodophenyl)amino)-5-oxopentanoic acid 5a, 2-(4-methylphenylsulfonamido)-5-((3-iodophenyl)amino)-5-oxopentanoic acid 5b and 2-(4-methylphenylsulfonamido)-5-((4-iodophenyl)amino)-5-oxopentanoic acid 5c showed high cytotoxicity in all cell lines with IC50 concentrations lines, except for the Human colon adenocarcinoma (HT-29) cell line as compare to standard drug, tamoxifen. The primary antitumor activity of tamoxifen by inhibition protein kinase C (Gelman, 1997) and also ability to facilitate the apoptosis in cancer cell not expressing estrogen receptor is due to generation of oxidative stress resulting in thiol depletion and activation of the transcriptional factor NF-kappaB. Many clinical studies explain the tamoxifen application in various kinds of malignant diseases (Ferlini et al., 1999; NCI, 1999).
Table II: In vitro anti-cancer activity (IC50) of synthesized compounds against human breast cancer (MCF-7), Leukemia (K-562), ovarian cancer (OVCAR-3), human colon adenocarcinoma (HT-29) and human kidney carcinoma (A-498)
Compound | Human breast cancer (MCF-7) |
Leukemia (K-562) | Ovarian cancer (OVCAR-3) |
Human colon adeno carcinoma (HT-29) | Human kidney carcinoma (A-498) |
---|---|---|---|---|---|
Compound | Human breast cancer (MCF-7) |
Leukemia (K-562) | Ovarian cancer (OVCAR-3) |
Human colon adeno carcinoma (HT-29) | Human kidney carcinoma (A-498) |
3 | 14.7 ± 0.0* | 13.2 ± 0.0a | 13.3 ± 0.0a | 26.4 ± 0.0a | 24.4 ± 0.0a |
5a | 12.2 ± 0.0* | 11.2 ± 0.0a | 13.3 ± 0.0a | 24.2 ± 0.0a | 27.3 ± 0.0a |
5b | 17.2 ± 0.0* | 24.1 ± 0.0a | 18.2 ± 0.0a | 30.2 ± 0.0a | 28.2 ± 0.0a |
5c | 15.2 ± 0.0* | 32.1 ± 0.0a | 15.5 ± 0.0a | 34.6 ± 0.0a | 31.2 ± 0.0a |
5d | 81.2 ± 0.0* | 67.2 ± 0.0a | 58.3 ± 0.0a | 71.2 ± 0.0a | 70.1 ± 0.0a |
5e | 88.2 ± 0.4 | 94.2 ± 0.2 | 87.2 ± 0.4 | >100 | 77.2 ± 0.4 |
Tamoxifen (standard) |
8.2 ± 0.0* | 10.4 ± 0.0a | 9.3 ± 0.0a | 14.2 ± 0.0a | 12.2 ± 0.0a |
Compounds with IC50 >100 µM were considered not active; ap< 0.05 compared to tamoxifen |
In vivo anti-cancer assay
All the newly synthesized final compounds 5a-e along with compound No. 3 were screened for their anti-cancer activity against Ehrlich Ascites Carcinoma (Table III) together with standard drug mitomycin-C. Among the synthesized compounds, the o-iodoanilide 5a, m-iodoanilide 5b and p-iodoanilide 5c derivatives showed encouraging activity in both the parameter, viz., ascetic fluid weight (93.25% for 5a, 91.73% for 5b and 92.33% for 5c) and ascetic cell count (96.20% for 5a, 95.29% for 5b and 93.04% for 5c) respectively as compare to mitomycin-C (100% in both the parameters). Hence, a detailed and prolonged study is necessary to establish their activity in other models.
Table III: In vivo anti-cancer activity of synthesized compounds against Ehrlich ascites carcinoma
Compound | AA | BB | CC | DD | EE | FF | GG |
---|---|---|---|---|---|---|---|
3 | 5 | 72.5 | 7.0 ±0.0a | 90.3 | 2.8 | 0.6 ± 0.1a | 76.2 |
5a | 5 | 72.5 | 2.7 ± 0.0a | 96.2 | 2.8 | 0.1 ± 0.0a | 93.3 |
5b | 5 | 72.5 | 3.4 ± 0.1a | 95.3 | 2.8 | 0.2 ± 0.0a | 91.7 |
5c | 5 | 72.5 | 5.0 ± 0.0a | 93.0 | 2.8 | 0.2 ± 0.0a | 92.3 |
5d | 5 | 72.5 | 11.5 ± 0.0a | 84.1 | 2.8 | 1.1 ± 0.1a | 58.6 |
5e | 5 | 72.5 | 12.7 ± 0.3 | 82.4 | 2.8 | 1.0 ± 0.2 | 64.3 |
Mitomycin-C (sandard) |
5 | 72.5 | 0.00 | 100.0 | 2.8 | 0.00 | 100.0 |
AA= Number of animals in each group; BB= Average No. of ascitic cells per mL in control, C (x 106 cells/mL); CC= Average no. of ascitic cells per mL in test ± SD, T (X 106 cells/mL); DD=Percent inhibition of ascitic cells (1-T/C) x 100; EE=Average weight of ascitic fluid in control C (g); FF= Average weight of ascitic fluid in test ± SD, T (g); GG= Percent inhibition of ascitic fluid (1-T/C) x 100; ap<0.05 compared to mitomycin-C |
Thus, five new compounds were designed, synthesized and characterized by chemical and instrumental methods and biologically screened for in vitro and in vivo antitumor activity along with an intermediate compound 3. It was noticed that final derivative compounds showing better activity than the parent compound and it may be due to the substituents present in those compounds.
References
Baskaran C, Ramachandran M. Computational molecular docking studies on anticancer drugs. Asian Pac J Trop Dis. 2012; 2: S734-38.
David SW, Knox C, Guo AC, Shrivastava S, Hassanali M. Drug Bank: A comprehensive resource for in silico drug discovery and exploration. Nucleic Acids Res. 2006; 34: D668-72.
De AU, Pal D. Possible antineoplastic agents I. J Pharm Sci. 1975; 64: 262-66.
De AU, Pal D. Possible antineoplastic agents II. J Pharm Sci. 1977; 66: 232-35.
Debnath B, Srikanth K, Banerjee S, Jha T. 1,5-N,N´-Disubstituted-2-(substituted benzenesulphonyl)-glutamamides as antitumor agents. Part 2. Synthesis, biological activity and QSAR study. Internet Electron J Mol Des. 2002; 1: 488-502.
Eidinoff ML. Pyrimidine studies: I. Effect of DON (6-Diazo-5-oxo-L-norleucine) on incorporation of precursors into nucleic acid pyrimidines. Cancer Res. 1958; 18: 105-09.
Ferlini C, Scambia G, Marone M, Distefano M, Gaggini C, Ferrandina G, Fattotossi A, Isola G, Benedetti Panici P, Mancuso S. Tamoxifen induces oxidative stress and apotosis in oestrogen receptor-negative human cancer cell line. Br J Cancer. 1999; 79: 257-63.
Gelman EP. Tamoxifen for the treatment of malignancies other breast and endometrial carcinoma. Semin Oncol. 1997; Suppl 1.24: 165-70.
Graff S, Rittenberg D, Foster GL. The glutamic acid of malignant tumors. J Biol Chem. 1940; 133: 745-52.
Hartman SC. Metabolic Pathways. New York, Academic Press, 1970, pp 1-4.
Holbeck SL. Update on NCI in vitro drug screen utilities. Eur J Cancer, 2004; 40: 785-93.
Keren R, Stark AA. Gamma-glutamyl transpeptidase-dependent mutagenicity and cytotoxicity of gamma-glutamyl derivatives: A model for biochemical targeting of chemotherapeutic agents. Environ Mol Mutagen. 1998; 32: 377-86.
Mosmann T. Rapid colorimetric assay for cellular growth and survival: Application to proliferation and cytotoxicity assays. J Immunol Methods. 1983; 65: 55-63.
NCI PDQ Clinical trial Search Bethesda 1999 (USA: National Cancer Institute).
Petit GR. Biosynthetic products for cancer chemotherapy. Vol. 1, New York, Plenum Press, 1977, pp 158-62.
Ray S, De AU. Synthesis and biological activity of succinimido benzene sulfonyl oxopyrrolidine analogs as possible antineoplastic agent. Asian J Chem. 2009; 21: 379-87.
Rodwell VW. Metabolism of purine and pyrimidine nucleotides. 25th ed. Stamford, Connecticut, Appleton and Lange, 2000, pp 386-401.
Rosowsky A, Wick MM, Kin SH. Structural analogs of L-glutamic acid-(4-hydroxyanalide) and -(3,4-dihydroxyanalide) as potential agents against melanoma. J Med Chem. 1979; 22: 1034-37.
Srivastava V, Kumar A, Mishra BN, Siddiqi MI. Molecular docking studies on DMDP derivatives as human DHFR inhibitors. Bioinformation 2008; 4: 180-88.
Sugita Y. The effect of antineoplaston, a new antitumor agent on malignant brain tumors. Kurume Med J. 1995; 42: 133-40.
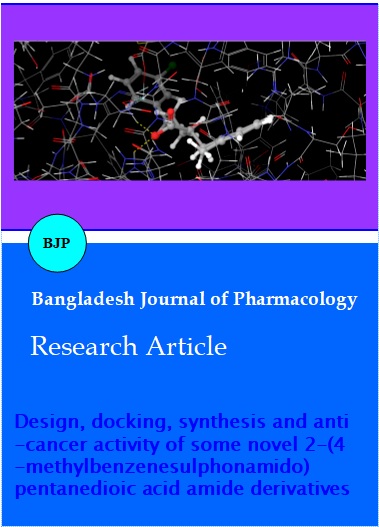