In silico analysis of compounds characterized from ethanolic extract of Cucurbita pepo with NF-κB-inhibitory potential
Abstract
NF-κB controls cellular growth properties of cells and its regulation is key to the management of disease like cancer. Although plant-derived bioactives have been reported to inhibit NF-κB, there is limited knowledge on the interactions between the phytochemicals and NF-κB. In this study, we identified the phytochemicals in ethanolic extract of Cucurbita pepo using Gas Chromatography-Mass Spectroscopy technique and used in silico approach to understand the interaction between the identified phytochemicals and NF-κB using Molegro Virtual Docker. The docking algorithm showed that nine phytochemicals fit well into the pocket on NF-κB. Our analysis showed that Lys144 is a prominent residue by involving in the binding of 9-octadecenoic acid (Z)-, methyl ester, hexadecanoic acid, methyl ester and octadecanoic acid, methyl ester with the moldock score of -55.5264, -57.4634 and -61.1258 respectively. Hence, the binding of these phytochemicals to NF-κB could be responsible for the anti-inflammatory and anticancer property of C. pepo.
Introduction
Nuclear factor of kappa B (NF-κB) regulates host inflammatory and immune responses and cellular growth properties by increasing the expression of cytokines and chemokines, the receptors involved in immune recognition. Cytokines that are stimulated by NF-κB, such as IL-1β and TNF-α, also directly activate the NF-κB pathway, thus establishing a positive autoregulatory loop that can amplify the inflammatory response. In addition, NF-κB is also involved in protecting cells from undergoing apoptosis in response to DNA damage or cytokine treatment resulting in the emergence of neoplasms (Karin et al., 2002).
Tumor cells in which NF-κB is constitutively active are highly resistant to anticancer drugs and inhibition of NF-κB activity in these cells greatly increases their sensitivity to such treatments (Karin et al., 2002). Hence, NF-κB is now recognized as an important target for treatment of cancer and many inflammatory diseases. Thus, attention has been paid in the last decade to the identification of compounds that can suppress the activity of NF-κB and activation of its pathway.
Plants are rich in bioactive chemicals that make them to serve as therapeutics and valuable in the treatment of disease like cancer and inflammation (Yamamoto and Gaynor, 2001; Fadeyi et al., 2013). One of such plants is Cucurbita pepo (Cucurbitaceae). It is commonly known as “pumpkin†and distributed in different regions of world. It has been employed in folk medicine to treat benign prostatic hypertrophy (Shokrzadeh et al., 2010).
This study, therefore, investigated the molecular interaction between the phytochemicals in the ethanolic extract of C. pepo and the human NF-κB.
Materials and Methods
Plant material
The leaves of C. pepo were collected from a local farm in Ilesa, Osun State, Nigeria and identification was done by morphological characteristics. These were then oven dried completely at 27 to 30°C for 1 week. The dried leaves were ground to fine powder using a local grinder. 50 g of the ground leaves was macerated in 500 mL of absolute ethanol and kept in orbital shaker for 48 hours. The extract obtained was evaporated to dryness using rotary evaporator and reconstituted to 1 mg/mL and used for gas chromatography-mass spectrometry (GC-MS) analysis.
GC-MS analysis
The GC-MS analysis was carried out using a Hewlett Packard Gas Chromatograph (Model 6890 series) equipped with a flame ionization detector and Hewlett Packard 7683 series injector, MS transfer line temperature of 250°C. The GC was equipped with a fused silica capillary column- HP-5MS (30 x 0.25 mm), film thickness 1.0 μm. The oven temperature was held at 50°C for 5 min holding time and raised from 50 to 250°C at a rate of 2°C/min, employing helium gas (99.9%) as a carrier gas at a constant flow rate of 22 cm/sec. One micron of extract at a split ratio of 1:30 was injected. MS analysis was carried out on Agilent Technology Network Mass Spectrometer (Model 5973 series) coupled to Hewlett Packard Gas Chromatograph (Model 6890 series) equipped with NIST08 Library software database. Mass spectra were taken at 70 eV/200°C, scanning rate of 1 scan/s. Identification of compounds was conducted using the database of NIST08 Library. Mass spectrum of individual unknown compound was compared with the known compounds stored in the software database Library.
Molecular docking
The SD files of the structures of the identified compounds were downloaded PubChem (http://pubchem. ncbi.nlm.nih.gov/) and used as ligands. The 3D structure of p50 dimer (chain A and B) of human NF-κB was retrieved from Protein Data Bank (PDB: 1NFK) and used for this study.
The docking of the ligands with NF-κB was done on the Molegro Virtual Docker (MVD) (Thomsen and Christensen, 2006). MVD was first used to prepare the structure of the ligands and protein. MVD uses the molecular docking algorithm called MolDock, which is based on a novel search algorithm that combines differential evolution with a cavity prediction algorithm, to determine the potential binding site of the target protein and ligands. The scoring scheme (‘dock score’ refers to the approximate binding energies between protein and ligand, generally expressed in kcal/mol) was derived from Piecewise Linear Potential scoring functions (Thomsen and Christensen, 2006; Ajay Babu et al., 2012).
Result and Discussion
The result of the GC-MS analysis shows the various compounds present in the leaves of C. pepo (Table I and Figure 1). At least, 14 peaks were identified by mass spectrometry from the extracts and the identities of the compounds are listed in Table I. The most abundant of the phytochemicals are the octadecadienoic acid derivatives with retention time (min) 27.9, 29.4, 29.5, 30.4, 30.5 and 33.5. These compounds, as well as other structurally similar compounds like hexadecanoic acid, methyl strearate and phytol, have been reported to be present in the ethanolic extracts of other anti-inflammatory plants (Aparna et al., 2012; Ezhilan and Neelamegam, 2012; Saeed et al., 2012; Marimuthu et al., 2013; Silva et al., 2014). Silva et al (2014) reported that phytol inhibits inflammation by reducing cytokine production and oxidative stress while Saeed (2012) reported that the derivatives of hexadecanoic acid prevented lipopolysaccharide-induced inflammation by suppressing the production of cytokines and NF-κB. The anti-inflammatory property of this compound has been linked to their structures (Aparna et al., 2012).
Table I: Phytochemical components identified in the ethanolic extract of C. pepo leaves by GC-MS showing their RT, area, molecular weight, X log P, number of H-bond donor and number of H-bond acceptor
Sl. No | RT | Area | Compound name | Molecular weight | X logP | H- Bond donor | H- Bond acceptor |
---|---|---|---|---|---|---|---|
1 | 25.637 | 1.16 | Bicyclo[3.1.1]heptane, 2,6,6-trimethyl- | 138.24992 | 3.9 | 0 | 0 |
2 | 26.976 | 3.20 | Hexadecanoic acid, methyl ester | 270.45066 | 7.9 | 0 | 2 |
3 | 27.977 | 0.85 | Octadecanoic acid, ethyl ester | 312.5304 | 8.9 | 0 | 2 |
4 | 29.471 | 2.64 | 9,12-Octadecadienoic acid (Z,Z)-, methyl ester | 294.47206 | 6.9 | 0 | 2 |
5 | 29.545 | 8.08 | 9-Octadecenoic acid (Z)-, methyl ester | 296.48794 | 7.6 | 0 | 2 |
6 | 29.763 | 3.24 | Phytol | 296.531 | 8.2 | 1 | 1 |
7 | 29.871 | 1.67 | Methyl stearate | 298.50382 | 9.0 | 0 | 2 |
8 | 30.495 | 10.50 | 9,12-Octadecadienoic acid (Z,Z)- | 280.44548 | 6.8 | 1 | 2 |
9 | 30.587 | 1.60 | 11-Octadecenoic acid, (Z)- | 282.46136 | 6.5 | 1 | 2 |
10 | 30.632 | 1.52 | 9-Oxabicyclo[6.1.0]nonane, cis- | 126.19616 | 2.3 | 0 | 1 |
11 | 31.348 | 8.78 | Cholest-4-en-3-one | 384.63766 | 8.4 | 0 | 1 |
12 | 31.382 | 4.24 | Cholest-5-en-3-one | 384.63766 | 8.2 | 0 | 1 |
13 | 33.545 | 1.57 | 9-Octadecenoic acid (Z)-, 2,3-dihydroxypropyl ester | 356.5399 | 6.5 | 2 | 4 |
14 | 34.764 | 1.73 | Oxirane, tetradecyl- | 240.42468 | 7.3 | 0 | 1 |
Figure 1: GC-MS chromatogram of ethanolic extract of C. pepo leaves
The molecular docking results showed that some of these phytochemicals can also directly bind to and inhibit the activity of NF-κB (Figure 2). Figure 3A-I shows the binding of the phytochemicals into the binding pocket (Figure 2) of the human NF-κB. This binding is stabilized by hydrogens (H) bond(s) between some amino acid(s) present within the binding pocket of NF-κB and H-bond acceptors on the ligands. The MolDock scores and the atoms involved in the bond formation are shown in Table II with oxirane, tetradecyl- having the best MolDock score of -87.0015 and followed by 11-Octadecenoic acid, (Z)- with MolDock score of -80.1009. Studies have shown that the plasma level of this omega-7 fatty acid has a negative correlation with inflammation (Block et al., 2012; Djoussé et al., 2014). The H-bond donors are majorly the Æ nitrogen of Lys and the guanidine nitrogen of Arg. For the binding of 9-octadecenoic acid (Z)-, 2,3-dihydroxypropyl ester the Æ nitrogen of Arg was also involved in the formation of the H-bond. Other amino acids involved in the formation of H-bond include Leu for octadecanoic acid, methyl ester, His and Asp for phytol, Glu for 9-octadecenoic acid (Z)-, 2,3-dihydroxypropyl ester as well as Gln for 9-oxabicyclo[6.1.0]nonane, cis-.
Table II: Docking results showing MolDock score, H-bond energy, number of H-bond and H-bond donor
S/N | Ligand | MolDock score | H-Bond | Number of H-bond | H-bond donor |
---|---|---|---|---|---|
1 | 9-Octadecenoic acid (Z)-, methyl ester | -55.5264 | -1.32052 | 1 | Lys144 |
2 | 9-Oxabicyclo[6.1.0]nonane, cis- | -2.66845 | -3.29281 | 2 | Lys275 Gln306 |
3 | 11-Octadecenoic acid, (Z)- | -80.1009 | -1.51686 | 1 | Lys272 |
4 | Hexadecanoic acid, methyl ester | -57.4634 | -2.16961 | 1 | Lys144 |
5 | 9-Octadecenoic acid (Z)-, 2,3-dihydroxypropyl ester | -35.9643 | -9.65187 | 4 | Arg54 Glu60 |
6 | Octadecanoic acid, methyl ester | -61.1258 | -4.24413 | 2 | Lys144 Leu207 |
7 | Phytol | -41.2086 | -3.00898 | 3 | His141 Phe55 Asp239 |
8 | Oxirane, tetradecyl- | -87.0015 | -2.17092 | 1 | Arg54 |
9 | cis-1,2-Epoxycyclooctane | -53.1884 | -1.52727 | Arg54 |
Figure 2: Stereoview of the NF-kappaB p50 dimer chain A and B. The binding pocket is highlighted in green
Figure 3: Stereo view of the binding of ligands to NF-kappa B. The ligands are highlighted in green. (A) cis-1,2-Epoxycyclooctane; (B) 9-Oxabicyclo[6.1.0]nonane, cis-; (C) 11-Octadecenoic acid, (Z)- (D) Hexadecanoic acid, methyl ester; (E) 9-Octadecenoic acid (Z)-, 2,3-dihydroxypropyl ester; (F) 9-Octadecenoic acid (Z)-, methyl ester; (G) Phytol; (H) Octadecanoic acid, methyl ester; (I) Oxirane, tetradecyl-
Several plant-derived bioactives have been reported as potential inhibitors of NF-κB by interacting with the enzyme directly (Nam, 2006). Using in silico and in vitro techniques Piccagli et al. (2008) reported similar binding of bioactives from medicinal plants against NF-κB and concluded that the docking result is predictive of biochemical activity.
Conclusion
Phytochemicals could account for account the anti-inflammatory and anticancer property of C. pepo.
References
Ajay Babu P, Colluru VT, Anaparthy N. In silico characterization of ECE-1 inhibitors. Comput Biol Med. 2012; 42: 446-57.
Aparna V, Dileep KV, Mandal PK, Karthe P, Sadasivan C, Haridas M. Anti-inflammatory property of n-hexadecanoic acid: Structural evidence and kinetic assessment. Chem Biol Drug Des. 2012; 80: 434-39.
Block R, Kakinami L, Liebman S, Shearer GC, Kramer H, Tsai M. Cis-vaccenic acid and the Framingham risk score predict chronic kidney disease: The multiethnic study of Atherosclerosis (MESA). Prostaglandins Leukot Essent Fatty Acids. 2012; 86: 175-82.
Djoussé L, Matsumoto C, Hanson NQ, Weir NL, Tsai MY, Gaziano JM. Plasma cis-vaccenic acid and risk of heart failure with antecedent coronary heart disease in male physicians. Clin Nutr. 2014; 33: 478-82.
Ezhilan BP, Neelamegam R. GC-MS analysis of phytocomponents in the ethanol extract of Polygonum chinense L. Pharmacognosy Res. 2012; 4: 11-14.
Fadeyi SA, Fadeyi OO, Adejumo AA, Okoro C, Myles EL. In vitro anticancer screening of 24 locally used Nigerian medicinal plants. BMC Complement Altern Med. 2013; 13: 79.
Karin M, Cao Y, Greten FR, Li ZW. NF-kappaB in cancer: From innocent bystander to major culprit. Nat Rev Cancer. 2002; 2: 301-10.
Marimuthu S, Padmaja B, Nair S. Phytochemical screening studies on Melia orientalis by GC-MS analysis. Pharmacog Res. 2013; 5: 216-18.
Nam NH. Naturally occurring NF-kappaB inhibitors. Mini Rev Med Chem. 2006; 6: 945-51.
Piccagli L, Fabbri E, Borgatti M, Bezzerri V, Mancini I, Nicolis E, Dechecchi MC, Lampronti I, Cabrini G, Gambari R. Docking of molecules identified in bioactive medicinal plants extracts into the p50 NF-kappaB transcription factor: correlation with inhibition of NF-kappaB/DNA interactions and inhibitory effects on IL-8 gene expression. BMC Struct Biol. 2008; 8: 38.
Saeed NM, El-Demerdash E, Abdel-Rahman HM, Algandaby MM, Al-Abbasi FA, Abdel-Naim AB. Anti-inflammatory activity of methyl palmitate and ethyl palmitate in different experimental rat models. Toxicol Appl Pharmacol. 2012; 264: 84-93.
Shokrzadeh M, Azadbakht M, Ahangar N, Hashemi A, Saeedi Saravi SS. Cytotoxicity of hydroalcoholic extracts of Cucurbita pepo and Solanum nigrum on HepG2 and CT26 cancer cell lines. Pharmacog Mag. 2010; 6: 176-79.
Silva RO, Sousa FB, Damasceno SR, Carvalho NS, Silva VG, Oliveira FR, Sousa DP, Aragão KS, Barbosa AL, Freitas RM, Medeiros JV. Phytol, a diterpene alcohol, inhibits the inflammatory response by reducing cytokine production and oxidative stress. Fundam Clin Pharmacol. 2014; 28: 455-64.
Thomsen R, Christensen MH. MolDock: A new technique for high-accuracy molecular docking. J Med Chem. 2006; 49: 3315-21.
Yamamoto Y, Gaynor RB. Therapeutic potential of inhibition of the NF-kappaB pathway in the treatment of inflammation and cancer. J Clin Invest. 2001; 107: 135-42.
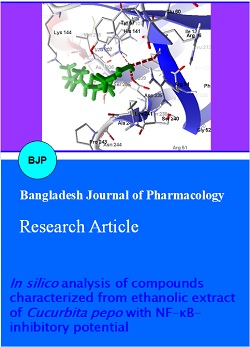