Daucosterol derived from Cordyceps (a Traditional Chinese Medicine) as a possible lead compound for allergic transfusion reactions
Abstract
Allergic transfusion reaction is an anomaly that develops within several hours of transfusion which is usually treated by antihistamine. In the present study, we are looking into daucosterol, an extract of Traditional Chinese Medicine (TCM) Cordyceps, as a potential inhibitor of histidine decarboxylase. The approach of molecular docking and Molecular Dynamics Simulation (MDS) on a traditional anti-inflammatory Chinese medicine gave an atomic insight of the inhibition. The daucosterol was binding with a ΔG of -3.17 Kcal/mol, to inhibit histamine synthesis by binding to histidine decarboxylase, possibly via the flexible catalytic loop. Such inhibitors that were designed to block the flexibility of the catalytic loop are potential candidates for further drug development program.
Introduction
Allergic transfusion reaction is one of the two most common forms of transfusion reaction, the other being febrile non-hemolytic transfusion reactions (Ezidiegwu et al., 2004; Patterson et al., 2000). Allergic transfusion reaction is classified as mild or severe based on the symptoms that develop within several hours of transfusion, mild allergic reactions cause erythematous circumscribed lesions, with or without pruritus while severe allergic reactions (anaphylaxis) causes systemic symptoms of dyspnoea, wheezing, hypotension, tachycardia, loss of consciousness, shock, and in rare cases death (Tobian et al., 2007). The pathophysiology of allergic transfusion reaction is little known (Heddle, 1999). For their prevention anti-inflammatory drugs with pre-transfusion medication are used, with its efficacy and safety in question (MartÃ-Carvajal et al., 2010). Three kinds of drugs are commonly used for this pre-transfusion medication, either alone or in combination (Patterson et al., 2000). United States Physicians use acetaminophen and diphenhydramine as effective therapies for fever and allergy, respectively (Geiger and Howard, 2007). Diphenhydramine is a first-generation antihistamine drug used for treating allergic transfusion reaction and causes drowsiness, affecting alertness, and impairing cognitive performance (Banerji et al., 2007). The other drug that is commonly used against anaphylaxis is hydrocortisone (cortisol), its anti-inflammatory and immunosuppressive property makes it a potent drug (Brunton et al., 2008).
However, these medications are not standardized and there is controversy about their effectiveness (Patterson et al., 2000). So in this study we are using computer-aided drug designing platform to find new leads against allergic transfusion reaction. Computer-aided drug designing techniques have become common in drug discovery (Kirchmair et al., 2008) and are very commonly used to design new leads against drug targets (Chikan et al., 2013). We are using some known anti-inflammatory Traditional Chinese Medicine (TCM) against the active site of histidine decarboxylase. The present study uses a crystal structure of the catalytic site of histidine decarboxylase, publicly available in Protein Data Bank (PDB) bearing accession code of 4E1O (Komori et al., 2012).
The major aim of this study is to explore novel histidine decarboxylase inhibitor compounds of natural origin. For which we structure-based molecular docking of anti-inflammatory TCM compound daucosterol (a constituent of Cordyceps) (Jiang et al., 2000; Li et al., 2003; Xu, 2002), using crystallographic structure of catalytic site of histidine decarboxylase. The final step involved Molecular Dynamics Simulation MDS of the TCM compound for 10 nanosec (ns).
Materials and Methods
Protein preparation: The 3D structure of histidine decarboxylase enzyme is available in Protein Data Bank. The structure of this chemical mediator of allergic reactions can be used to design novel drugs that prevent histamine biosynthesis. The selected protein 3D structure is having six structures, hence only structure A was isolated from the structure and used for docking by using SPDB Viewer. The SER 354 residue of histidine decarboxylase was targeted for designing novel inhibitor.
Molecular docking: Autodock 4.2 (Morris et al., 1998) was used for the Molecular docking studies of the daucosterol with the receptor protein i.e. histidine decarboxylase. The software uses binding free energy evaluation to find the best binding mode. The energy values are calculated by the characterization of internal energy of ligand, torsional free energy and intermolecular energy that consist of van der Walls energy, hydrogen bonding energy, desolvation energy, and electrostatic energy.
Molecular dynamics simulation: MDS approach using GROMACS 4.5.6 was used to study protein lead interaction. In our case we used the software to check the stability of the protein-drug complex over time. The complex was subjected to OPLS force field followed by addition of water molecules using a simple point charge (SPC216) model using pdb2gmx tool. The whole system was ensured to be overall neutral by charge of the simulated system using genion tool. Energy minimization process and position restraint procedure was performed with NVT and NPT ensembles (Berendsen et al., 1995; Berendsen et al., 1997; van Gunsteren et al., 1996; Hess et al., 1996) using mdrun tool, For NVT ensemble a constant temperature of 300 K was maintained with a coupling constant of 0.1ps with time duration of 100 ps. After stabilization of temperature an NPT ensemble was performed with same parameters. A final MD run is performed for 10ns for the Drug-enzyme complex. All the visualizations were carried out using Pymol, VMD tools and graphs were plotted using XM grace Program (DeLano, 2009; Humphrey et al., 1996). The trajectories were analyzed using inbuilt GROMACS distribution tools.
Result and Discussion
The three dimensional structure of histidine decarboxylase retrieved from PDB database was modified in SPDB Viewer to single structure, as shown in Figure 1. The possible conformation of the refined protein was obtained using procheck analysis, visualized with the aid of Ramachandran plot by checking the dihedral Phi and Psi angles of amino acid residues. This refined structure of histidine decarboxylase (PDB ID: 4E1O) was subjected for performing the docking studies using AUTODOCK 4.2 software. The TCM compound daucosterol was docked into the optimized catalytic binding site (Figure 2) of the HDC and the results were analyzed using Discovery Studio 3.5 client software. In Table I we have shown the results generated. The top two interactions based on binding energy of ducosterol were found to form hydrogen bond with the HDC (Table II).
Figure 1: The transition of 4E01 to Chain A using SPDB viewer
Figure 2: Interaction of daucosterol with the catalytic loop of HDC represented in solid ribbon and surface
Table I: Gibbs free energy of top five poses of daucosterol with HDC
Rank | ΔG (Kcal/mol) |
---|---|
1 | -3.17 |
2 | -3.17 |
3 | -3.16 |
4 | -1.34 |
5 | -1.18 |
Table II: AutoDock analysis of top two poses
Rank | Ligand binding pocket | H-bonds |
---|---|---|
1 | ASN100, CYS101, LEU102, PHE 104, SER108, TYR334, LEU335, LEU353 | ASN100: - Daucosterol: H10 LEU102:NH-Daucosterol: O4 SER108:O- Daucosterol: H99 |
2 | ILE99, ASN100, CYS101, LEU102, PHE 104, SER108, TYR334, LEU353 SER354 | ASN100: O - Daucosterol: H96 ASN100:O- Daucosterol: H99 |
The ligand-receptor interaction (covalent or non covalent) and the number of hydrogen bonds formed has been displayed in Figure 3. The best interaction has a ΔG of -3.17 Kcal/mol and forms three hydrogen bond with ASN100, LEU102, and SER108 of the enzyme's binding pocket. The binding pocket in this case is of following amino acids ASN100, CYS101, LEU102, PHE 104, SER108, TYR334,LEU335 and LEU353. The H10 , H99 and O4 position of the ligand is interacting with ASN100, LEU102, and SER108 respectively with distance of 2.12A, 1.97A and 2.15A. Figure 4a shows the mapping of top pose against the hit (ASN100, LEU102, and SER108). The second best pose Figure 4b also has a ΔG of -3.17 Kcal/mol, but forms only two hydrogen bond in the enzyme's binding pocket. The binding pocket in this case is of following amino acids ILE99, ASN100, CYS101, LEU102, PHE 104, SER108, TYR334, LEU353 and SER354.The H96and H99 position of the ligand is interacting with ASN100 with distance of 1.72A and 2.26A.
The MDS were performed on the best complex of daucosterol with HDC protein. Initially the system was energy minimized for a period of 1 ns and a position restraints simulation was performed using steepest decent integrator on a stepwise manner. The RMSD and RMSF of the HDC-daucosterol complex was checked (Figure 5), for the protein alone it was about 0.242 nm after 10 ns of MD simulation concluding the complex to be stable throughout the run. The RMSF of the protein represented by black in Figure 5b and complex in red color is showing the function throughout, indicating the effect of drug on the structure of histidine decarboxylase protein. The protein folding process shows good reliability as the protein structure is quite stable during the period of simulation.
Using an in silico structure-based approach, we have generated the data for daucosterol as a specific inhibitor for histidine decarboxylase. Out of the top 5 poses made by daucosterol, the first pose was selected for MDS. The Molecular docking as well as MDS is showing tremendous potential for daucosterol in blocking the active site of histidine decarboxylase protein, thus making it a suitable compound for further dug development.
Figure 3: Two dimensional representation of the binding pocket of top two poses generated by doucosterol with the active site of HDC
Figure 4: Three dimensional representation by scaled ball and stick of top two poses of doucoserol with the physically interacting amino acids of HDC enzyme, (A) pose 1 (B) pose 2
Figure 5: (a) HDC-daucosterol complex RMSDs at 300 K Black line represents HDC and red represents daucoserol, (b) RMSF of each residue with (black ) and without drug (green), showing the change in the flexibility of the catalytic loop
Acknowledgement
We would like to sincerely thank Yantai Blood Center for providing the facilities for this research.
References
Banerji A, Long AA, Camargo CA Jr. Diphenhydramine versus nonsedating antihistamines for acute allergic reactions: A literature review. Allergy Asthma Proc. 2007; 28: 418–26.
Berendsen HJC, Grigera JR, Straatsma TP. The missing term in effective pair potentials. J Phys Chem. 1997; 91: 6269–71.
Berendsen HJC, van der Spoel D, van Drunen R. GROMACS: A message-passing parallel molecular dynamics implementation. Comp Phys Comm. 1995; 91: 43–56.
Brunton LL, Parker KL, Blumenthal D, Buxton I. Manual of pharmacology and therapeutics. 11th ed. New York, McGraw Hill, 2008, pp 1-25.
Chikan NA, Bhavaniprasad V, Anbarasu K, Shabir N, Patel TN. From natural products to drugs for epimutation computer-aided drug design. Appl Biochem Biotechnol. 2013; 170: 164-75.
DeLano WL. Pymol. Schrodinger LLC, Portland, OR. 2009.
Ezidiegwu CN, Lauenstein KJ, Rosales LG, Kelly KC, Henry JB. Febrile nonhemolytic transfusion reactions: Management by premedication and cost implications in adult patients. Arch Pathol Lab Med. 2004; 128: 991-95.
Geiger TL, Howard SC. Acetaminophen and diphenhydramine premedication for allergic and febrile nonhemolytic transfusion reactions: Good prophylaxis or bad practice? Transfus Med Rev. 2007; 21: 1–12.
Heddle NM. Pathophysiology of febrile nonhemolytic transfusion reactions. Curr Opin Hematol. 1999; 6: 420–26.
Hess B, Bekker H, Berendsen HJC, Fraaije J. LINCS: A linear constraint solver for molecular simulations. J Comp Chem. 1996; 18: 1463–72.
Humphrey W, Dalke A, Schulten K. VMD: Visual molecular dynamics. J Mol Graphics. 1996; 14: 33–38.
Jiang H, Liu K, Meng S, Chu Z. Chemical constituents of the dry sorophore of Cordyceps militarys. Acta Pharma Sin. 2000; 35: 663–68.
Kirchmair J, Distinto S, Schuster D. Enhancing drug discovery through in silico screening: Strategies to increase true positives retrieval rates. Curr Med Chem. 2008; 15: 2040-53.
Komori H, Nitta Y, Ueno H, Higuchi Y. Structural study reveals that Ser-354 determines substrate specificity on human histidine decarboxylase. J Biol Chem. 2012; 287: 29175-83.
Li JX, Li J, Xu LZ, Yang SL, Zou ZM. Studies on chemical constituents of Cordyceps sinensis (Berk) Sacc. Chin Pharm J. 2003; 38: 499–501.
MartÃ-Carvajal AJ, Solà I, González LE, Leon de Gonzalez G, Rodriguez-Malagon N. Pharmacological interventions for the prevention of allergic and febrile non-haemolytic transfusion reactions. Cochrane Database Syst Rev. 2010; 6: CD007539.
Morris GM, Goodsell DS, Halliday RS, Ruth Huey, William EH, Richard KB, Arthur JO. Automated Docking using a lamarckian genetic algorithm and empirical binding free energy function. J Computational Chem. 1998; 19: 1639-62.
Patterson BJ, Freedman J, Blanchette V, Sher G, Pinkerton P, Hannach B, Meharchand J, Lau W, Boyce N, Pinchefsky E, Tasev T, Pinchefsky J, Poon S, Shulman L, MacK P, Thomas K, Blanchette N, Greenspan D, Panzarella T. Effect of premedication guidelines and leukoreduction on the rate of febrile nonhaemolytic platelet transfusion reactions. Transfus Med. 2000; 10: 199–206.
Tobian AA, King KE, Ness PM. Transfusion premedications: A growing practice not based on evidence. Transfusion. 2007; 47: 1089–96.
van Gunsteren WF, Billeter SR, Eising AA, Hünenberger PH, Krüger P, Mark AE, Scott WRP, Tironi IG. Bimolecular simulation: GROMOS96 manual and user guide. BIOMOS, Zurich, Groningen. 1996, pp 1-1042.
Xu L. Chinese materia medica: Combinations and applications. 2002.
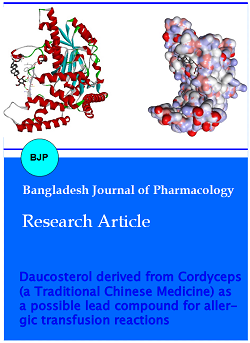