Scopoletin potentiates the anti-cancer effects of cisplatin against cholangiocarcinoma cell lines
Abstract
Chemotherapy with cisplatin in cholangiocarcinoma produces adverse effects and leads to resistance development by tumors. We aimed to evaluate anti-cancer effects by coadministration of cisplatin and scopoletin in cholangiocarcinoma cells. MTT assay, median effect principle, cell cycle arrest and apoptosis assay were conducted to determine anti-cancer effects. Results revealed that treatment with cisplatin and scopoletin resulted in dose-dependent reduction of cell viability for cholangiocarcinoma cells. Combination of these agents inhibited proliferation of cells significantly more than single agent either. Combination indices reflect additive cytotoxic effect, leading to >2 times dose reduction for each agent. Both the cell cycle arrest (G0/G1) and apoptosis induction underling the enhanced cytotoxicity for the combination. Besides, single agent conferred cell cycle arresting and apoptotic effects in cholangiocarcinoma cells. By contrast, non-cancer cells were less affected with combination. Our observations suggest that cisplatin and scopoletin combination may bring positive significance in cholangiocarcinoma treatment.
Introduction
Cholangiocarcinoma (CCA) is a fatal adenocarcinoma with poor prognosis, and a health problem worldwide (Gores, 2003). Though surgical removal is the only curative approach (Kuhlmann, 2012), the recurrence rate is approximately 60% even after resection (Aljiffry et al., 2009). Chemotherapy with cisplatin, 5-fluorouracil and gemcitabine either alone or in combination has been practiced to improve survival of patients with cholangiocarcinoma (Thongprasert, 2005). Treatment with cisplatin has some drawbacks, including toxicity, adverse effects and resistance of tumors (Siddik, 2003). These limitations warrant the desperate search for relatively non-toxic natural products as chemopreventive agents (Pan and Ho, 2008).
Several natural products and their derivatives have been found to exhibit immense pharmacological and biological properties including molecular interventions in carcinogenesis (Saha and Khuda-Bukhsh, 2013). The ability of natural products to bind a variety of protein domains and folding motifs makes them effective modulators of cellular processes such as immune responses, signal transduction, mitosis and apoptosis (Peczuh and Hamilton, 2000). It was reported that coumarin inhibited the growth of HeLa cells (Chuang et al., 2007) and cholangiocarcinoma cells (Prakobwong et al., 2011). Phenolic compound scopoletin (7-hydroxy-6-methoxycoumarin) is ubiquitous in the plant kingdom (Peterson et al., 2003). Studies demonstrated that scopoletin exhibited significant pharmacological activities including antitumor, antiangiogenic (Zhou et al., 2012) and anti-inflammatory activities (Kang et al., 1999; Moon, 2007). Currently, a number of natural products are being evaluated in vitro and in vivo to demonstrate their additive or synergistic interaction with clinically approved anti-cancer drugs. However, there is no report on combined effects of scopoletin and cisplatin. Therefore, we aimed to evaluate potential of scopoletin for enhancing anti-cancer effects of cisplatin in cholangiocarcinoma cells.
Materials and Methods
Reagents and media
Cisplatin and scopoletin were purchased from the Sigma-Aldrich (USA). Culture media RPMI-1640, DMEM and supplements including antibiotics and fetal bovine serum (FBS) were purchased from Gibco Invitrogen Corporation (USA). FITC Annexin V apoptosis detection kit was purchased from BioLegend Inc. (San Diego, CA, USA). All other chemicals were purchased from Sigma-Aldrich (USA). Cisplatin was dissolved in normal saline (0.18 mg/mL) and kept in 4°C. Scopoletin was dissolved in dimethyl sulfoxide (DMSO) and stored at -20ºC until use. The final concentration of DMSO was less than 0.5% (v/v), which was also present in the corresponding controls.
Cell lines and cell culture
In this study, two cholangiocarcinoma cell lines, KKU-100 (poorly differentiated) and KKU-M214 (moderately differentiated), and a human bile duct epithelial cell line (H69 cells) were used. Cancer cell lines were cultured in RPMI-1640 medium supplemented with 10% fetal bovine serum, penicillin (100 U/mL), and streptomycin (100 µg/mL) (Gibco-BRL). H-69 cells were cultured in enriched Dulbecco's Minimum Essential Medium (DMEM) (Gibco, Invitrogen, USA) containing 10% (v/v) fetal bovine serum (FBS) (Gibco, Invitrogen), 100 U/mL penicillin (Gibco, Invitrogen), 100 ug/mL streptomycin (Gibco, Invitrogen), 25 ug/mL adenine (Sigma, USA), 5 ug/mL insulin (Gibco, Invitrogen), 1 ug/mL epinephrine (Sigma), 13.6 ng/mL T3T triiodo-L-thyronine (T3) (Sigma), 8.3 ug/mL holotransferrin (Gibco, Invitrogen), 0.62 ug/mL hydrocortisone (Sigma) and 10 mg/mL epidermal growth factor (EGF; CytoLab Ltd., Israel). The cells were incubated at 37°C in a humidified atmosphere with 5% CO2.
Cell viability assay
Cell growth inhibition was determined by MTT (3-(4, 5-dimethylthiazol-2-yl)-2, 5-diphenyltetrazolium bromide) assay as described elsewhere (Senawong et al., 2013). Briefly, cells were seeded at a density of 8 x 103 cells/well into 96 well plates in triplicate and incubated for 24 hours for adherence. After 24 hours, these cells were treated with different concentrations of cisplatin and scopoletin for 24, 48 and 72 hours. For combination study, the subtoxic dose for each agent against each cell line alone and in combination was used. Vehicle control group was exposed to DMSO (final concentration of 0.5%) or isotonic saline. After desired period, the medium was removed and cells were incubated with MTT (0.5 mg/mL in PBS) for 2 hours. Formazan formed after MTT reduction was dissolved in DMSO, and the absorbance at 550 nm was measured with a microtiter plate reader (Bio-Rad Laboratories, USA). The absorbance at 655 nm was used as a reference wavelength. The number of viable cells is proportional to the production of formazan. Cell viability was determined as a percentage by the following equation:
%Cell viability = [Sample O.D./Control O.D.] x 100
Analysis of drug interaction
To study drug interaction, cells were seeded into a 96-well plate and allowed to adhere for 24 hours. After that, cells were treated with cisplatin and scopoletin alone or in combination for 72 hours. Then cell viability was determined by MTT assay and the half maximal inhibitory concentration (IC50 value) in combination was calculated. Nature of interaction between cisplatin and scopoletin was evaluated according to the median-drug effect principle described by Chou and Talalay (1984) and Chou (2006). The combination index (CI) and dose reduction index (DRI) reflect the nature of drug interaction and the magnitude of dose to be reduced as a favorable response to interaction without hampering killing efficiency, respectively. For 50% growth inhibition, the combination index (CI) values for agents with mutually non-exclusive mode of actions were calculated based on the equation stated below:
CI = {(D)1/(Dx)1 +(D)2/(Dx)1+((D)1(D)2) / ((Dx)1.(Dx)2)}
where (D)1 and (D)2 are doses of drug 1 (cisplatin) and drug 2 (scopoletin) in combination that inhibit 50% of cell proliferation. (Dx)1 and (Dx)2 are the doses of drug 1 (cisplatin) and drug 2 (scopoletin) alone that also inhibit 50% of cell proliferation (Chou and Talalay, 1984; Chou, 2006). α = 1 for mutually non-exclusive modes of drug action. CI< 0.9 indicates synergism; CI = 0.9-1.1 indicates an additive relationship, and CI >1.1 indicates antagonism. The dose reduction index (DRI), which determines the magnitude of dose reduction allowed for each drug in a combination as compared to the concentration of single agent required to achieve the same effect, was also calculated (Chou and Talalay, 1984; Chou, 2006). The equation for dose reduction is as follows:
(DRI)1 = (Dx)1/(D)1
(DRI)2 = (Dx)2/(D)2
Flow cytometric analysis of cell cycle profile
For cell cycle analysis, KKU-100 and KKU-M214 cells were seeded at the density of 2.5 x 105 cells/mL in a 4.5-cm culture dish. After 24 hours, cells were treated with cisplatin and scopoletin alone and in combination and incubated for 24 hours. After exposure to 24 hours, the percentages of cells distributed in different phases of cell cycle were determined by flow cytometry using propidium iodide (PI) staining. Briefly, cells were harvested, washed with PBS and centrifuged at 3,000 rpm for 3 min. Pellets were fixed in 70% cold ethanol at 4°C for 1 hour. After fixation, cells were washed with PBS twice and incubated with 0.5 mg/mL RNase (RNase A type I-A; Sigma) for 1 hour to avoid double stranded RNA staining. Lastly, nuclear DNA staining was carried out using propidium iodide (50 µg/mL) in PBS solution under subdued light for 40 min at room temperature. The DNA histograms reflecting cell cycle distribution were determined using BD FACanto II Calibur flow cytometer (Becton-Dickinson, UK).
Flow cytometric analysis of apoptosis
Apoptosis induction of KKU-100 and KKU-M124 cells was analyzed by flow cytometer using the Annexin V-FITC apoptosis detection kit according to the manufacturer's instructions. Briefly, cells were seeded at the density of 2.5 x 105 cells/mL in a 4.5-cm culture dish and cultured for 24 hours. Cells were treated with cisplatin and scopoletin alone and in combination for 24 hours. After 24 hours of exposure, cells were harvested by trypsinization, washed with cold PBS and centrifuged at 3,000 rpm for 3 min. The pellets were resuspended in the annexin binding buffer. Cell density was determined and diluted in the Annexin binding buffer to 105 cells per assay. Cells were incubated with annexin V-FITC and propidium iodide (PI) at room temperature for 15 min. Following the incubation, cells were analyzed by flow cytometry using a BD FACanto II calibur flow cytometer (Becton-Dickinson, UK). The flow cytometry results were compared with conventional cell count and morphology under a fluorescence microscope.
Statistical analysis
All experiments were repeated independently at least three times. Data were expressed as means ± standard deviation (SD) from three independent experiments. Test for significant differences between sample-treated and solvent-treated cells was carried out using one-way ANOVA with Duncan's post hoc test. The criterion for statistical significance was set at p<0.05.
Result and Discussion
To evaluate the therapeutic efficacy of scopoletin and cisplatin in cholangiocarcinoma cell lines, cell viability of KKU-100 and KKU-M214 cells was assessed. Results from dose-response curve revealed that cisplatin inhibited proliferation of KKU-100 and KKU-M214 in a dose- and period-dependent fashion, with IC50 values of 7.1 ± 0.7 µM and 12.8 ± 0.5 µM at 72 hours, respectively. Like-wise, scopoletin treatment resulted in significant reduction of cell viability in KKU-100 and KKU-M214 in a concentration- and time-dependent manner when treated at 0-500 µM concentrations (Figure 1). The estimated IC50 values reflecting sensitivity against cell lines were 486.2 ± 1.5 µM and 493.5 ± 4.7 µM for KKU-100 and KKU-M214 cells for 72 hours exposure, respectively.
Figure 1: Effect of cisplatin and scopoletin alone or in combination on the cell viability of CCA cells. KKU-100 and KKU-M214 cells were treated with various concentrations of (A, B) cisplatin, (C, D) scopoletin alone or (E, F) in combination for 24, 48 and 72 hours and analyzed by MTT assay. For combination study: (E) KKU-100 cells were treated with cisplatin alone (3 µM), scopoletin alone (250 µM), and combination of cisplatin (3 µM) and scopoletin (250 µM); (F) KKU-M214 cells were treated with cisplatin alone (6 µM), scopoletin alone (250 µM), and combination of cisplatin (6 µM) and scopoletin (250 µM). Data were expressed as mean ± SD of three individual experiments. The letter "a" indicates significant difference between drug and solvent control treatments while "b" indicates significant difference between combination and single drug treatments (p<0.05)
In agreement with our findings, several researchers documented the cytotoxic effects of cisplatin and scopoletin on various cancer cells. Cisplatin has been reported to produce cytotoxic effects against NSCLC cells (Chougule et al., 2011). A similar type of finding was reported by Li et al. (2012) who demonstrated that cisplatin-induced cytotoxicity in SC-M1 gastric cancer cells in a dose-dependent manner. Scopoletin and its several derivatives developed by a systematic combinatorial chemical approach have been reported to show anti-tumor potential against mammary (MCF-7 and MDA-MB231) and colon (HT-29) carcinoma cell lines (Liu et al., 2012). Treatment with scopoletin was found to reduce the viability of fibroblast-like synoviocytes (Li et al., 2009) and suppress proliferation of PC3 cells (a human prostate adenocarcinoma cell line) (Liu et al., 2001) and HL-60 cells (a promyeloleukemic cell line) (Kim et al., 2005) in a dose- and time-dependent manner. It is important to report that some synthetic derivatives of scopoletin exerted profound cytotoxic effects than the prototype scopoletin in both tumor cells and human umbilical vein endothelial cells (Cai et al., 2013). Besides, some other coumarin compounds have also been reported to have anti-cancer effects. Resveratrol, a plant compound called polyphenol, has been demonstrated to inhibit the proliferation of SK-ChA-1 cells (Roncoroni et al., 2008) and KKU-100 and KKU-M156 cells (Hahnvajanawong et al., 2011).
The bar graphs (Figures 1E and 1F) depict the effects of concurrent co-treatments of cisplatin and scopoletin on antiproliferative activity of KKU-100 and KKU-M214 cells. As demonstrated, the combined exposure of cisplatin (3 µM) and scopoletin (250 µM) resulted in cell viability reduction of 39.1 ± 2.0% in KKU-100 while cisplatin (3 µM) alone reduced cell viability to 71.9 ± 3.3% and scopoletin (250 µM) reduced cell viability to 72.4 ± 4.3%. In KKU-M214 cells, the cell viability decreased to 42.6 ± 2.1% for the combination of cisplatin (6 µM) and scopoletin (250 µM), which was significantly lower than cisplatin (73.2 ± 1.7%) and scopoletin (73.3 ± 4.8%) alone. The inhibition of cell growth by this combination was additive in that its effect was equal to the sum of the effects of the two compounds separately. For 50% growth inhibition, the combination index (CI) values (Table I), determined using median effect principle, reflect the additive interaction between cisplatin and scopoletin against both cancer cell lines, which leads to positive dose reduction.
Table I: Combination index (CI) and dose reduction index (DRI) at IC50 level of cisplatin and scopoletin against CCA cells for 72 hours exposure
Cell lines | Drug combination | Combination Index (CI) | Dose reduction index (DRI) | |
---|---|---|---|---|
Cisplatin | Scopoletin | |||
KKU-100 | Cisplatin + Scopoletin | 1.0 | 2.5 | 2.2 |
KKU-M214 | Cisplatin + Scopoletin | 1.0 | 2.5 | 2.3 |
Our results are congruous with findings of several researchers where they documented additive to synergistic interaction between cisplatin and some natural compounds. Synergistic anti-cancer effects between noscapine (an alkaloid) and cisplatin have been reported in lung tumor NSCLC cells and these synergistic effects are through induction of apoptosis via intrinsic and extrinsic pathways, activation of growth inhibitory, and inhibition of survival proteins (Chougule et al., 2011). The cytotoxicity of cisplatin in H460 cells was modulated in a synergistic manner by sabarubicin (Bigioni et al., 2008). Several other studies have provided evidence that enhanced tumor growth inhibition can be achieved by combining cisplatin with other natural compounds. Esculetin (6,7-dihydroxycoumarin) potentiates anti-cancer activity of cisplatin in DMBA-induced mammary tumor in rat (Tikoo et al., 2011). The cytotoxic interactions between curcumin and cisplatin were synergistic in nature (Notarbartoloa et al., 2005).
To further justify the therapeutic application of drug combination, the impacts of this combination on proliferation of a non-cancer cell line (H69 cells) was examined. For combination study, the highest subtoxic doses used against KKU-100 and KKU-M214 cells for each agent were selected to evaluate. Figure 2 shows the antiproliferative activity of cisplatin and scopoletin alone and in combination in H69 cells. Dose response curve demonstrates that cisplatin and scopoletin were relatively ineffective against H69 cells, with IC50 values of 15.1 ± 0.6 µM and >500 µM, respectively, for 72 hours exposure. The combination of cisplatin (6.0 µM) with scopoletin (250 µM) reduced the viability to 63.7 ± 1.9% for 72 hours exposure while cisplatin (6.0 µM) reduced the viability 80.0 ± 1.7% and scopoletin (250 µM) to 85.6 ± 2.1% (Figure 2). The cell viability of a non-cancer cell line for the combination was still higher when compared with cancer cell lines, KKU-100 (39.1 ± 2.0%) and KKU-M214 (42.6 ± 2.1%) cells after concurrent treatment with cisplatin and scopoletin. It has been reported that scopoletin and its derivatives have lower cytotoxic effects on PBMC than tumor cells (Cai et al., 2013). A prenylated coumarin, diversin, has been observed to exert considerable cytotoxic effects in bladder carcinoma 5637 cells, but not on normal human fibroblast cells (Haghighitalab et al., 2014). In PBMC, only large concentrations of the coumarin compounds elicited a cytostatic action (Lopez-Gonzalez et al., 2004).
Figure 2: Effect of cisplatin and scopoletin alone or in combination on the cell viability of a non cancer cell line (H69 cells). Cells were treated with (A) cisplatin alone, (B) scopoletin alone and (C) combination of cisplatin and scopoletin for 24, 48 and 72 hours, and analyzed by MTT assay. For combination study, cells were treated with cisplatin alone (6 µM), scopoletin alone (250 µM), and combination of cisplatin (6 µM) and scopoletin (250 µM). Data were expressed as mean ± SD of three individual experiments
To investigate the mechanism underlying the cytotoxic potentiation of cisplatin with scopoletin, we aimed to analyze the cell cycle profile of propidium iodide (PI) stained cells using flow cytometry. The percentage of cells distributing in different phases of the cell cycle were determined based on the cellular DNA content at different cell cycle phases. As presented in Table II, in the case of KKU-100 cells, data from flow cytometry revealed that cisplatin (10 µM) produced growth arrest at G1 check point leading to higher percentage(57.9 ± 3.3%) of cells at G0/G1, preventing G1/S entry. Scopoletin (500 µM) induced significant G0/G1 arrest with 71.0 ± 1.3%, and concomitant reduction of S phase cells (by 20.2%) when compared with control (36.6 ± 1.3%). But when the cells were exposed to combination treatment, they underwent both G0/G1 arrest and apoptosis as evidenced from more sub-G1 number. In the case of KKU-M214 cells, the cell cycle profile showed no growth arrest at any particular phase when compared with treatment and control. Single agent and combination treatment resulted in increased sub-G1 fraction where the combination produced the highest percentage (25.2 ± 1.8%) followed by cisplatin (18.4 ± 1.9%) and then scopoletin (8.9 ± 1.1%).
Table II: Effect of cisplatin alone, scopoletin alone and combination of cisplatin and scopoletin on cell cycle profile of CCA cells
Cell lines | Treatments | Cell cycle distribution (%) ± SD | |||
Sub-G1 | G0/G1 | S | G2/M | ||
KKU 100 | Solvent control | 3.0 ± 1.0 | 36.6 ± 1.3 | 26.1 ± 1.1 | 34.3 ± 1.2 |
Cisplatin (10 µM) | 12.5 ± 1.5a | 57.9 ± 3.3a | 12.9 ± 1.3a | 16.8 ± 1.7a | |
Scopoletin (500 µM) | 4.9 ± 0.6 | 71.0 ± 1.3a | 5.9 ± 0.7a | 18.3 ± 1.4a | |
Cisplatin (10 µM) + Scopoletin (500 µM) |
16.3 ± 0.9a | 50.8 ± 1.9a,b | 16.1 ± 1.3a,b | 16.9 ± 1.4a | |
KKU M214 | Solvent control | 5.1 ± 0.3 | 67.1 ± 1.4 | 12.6 ±1.3 | 15.2 ± 1.5 |
Cisplatin (10 µM) | 18.4 ± 1.9a | 48.9 ± 1.1a | 15.4 ± 2.1 | 17.4 ± 1.6 | |
Scopoletin (500 µM) | 8.9 ± 1.1 | 69.4 ± 2.2 | 7.9 ± 0.9a | 13.8 ± 2.1 | |
Cisplatin (10 µM) + Scopoletin (500 µM) |
25.2 ± 1.8a,b | 50.1 ±1.9a,b | 12.3 ± 1.0 | 12.4 ± 2.1 |
Our findings on cell cycle arrest are corroborated by some earlier studies. Cisplatin mediated cell cycle arrest at the G1/S checkpoint with an increase of cells in the G0/G1 phase and reduction of cells in the S phase (Sato et al., 2006). Cisplatin has been demonstrated to retard cell cycle progression of lung cancer cells by accumulation in the G1 phase and the concomitant reduction of cells in the S phase of the cell cycle (Wang et al., 2004). Scopoletin prevented proliferation of human prostate tumor PC3 cells by arresting cell cycle at G0/G1 and S phases (Liu et al., 2001). Diversin was found to produce considerable cytotoxic effects in bladder carcinoma 5637 cells by blocking G2 phase of the cell cycle (Haghighitalab et al., 2014). It has been reported that coumarin and 7-hydroxycoumarin inhibited cell growth by inducing cell cycle arrest in the G1 phase in lung carcinoma cell lines (Lopez-Gonzalez et al., 2004). Resveratrol was observed to significantly inhibit CCA cell growth in a dose- and time-dependent manner by inducing cell cycle arrest at the G0/G1 phase in KKU-100 cells and the S and G2 phases in KKU-M156 cells (Hahnvajanawong et al., 2011). Representative DNA histograms of cell cycle profile are depicted in Figure 3.
Figure 3: Representative DNA histograms on cell cycle profile of CCA cells. KKU-100 and KKU-M214 cells treated with DMSO (0.5%; v/v) as a solvent control, cisplatin alone (10 µM), scopoletin alone (500 µM) and combination of cisplatin (10 µM) and scopoletin (500 µM) for 24 hours were subjected to cell cycle analysis by flow cytometry using propidium iodide (PI) staining. Histograms showed a number of cells per channel (vertical axis) vs DNA content (horizontal axis)
After evaluation of the cytotoxic effects, we further aimed to explore the mechanisms of cytotoxicity enhancement of cisplatin by scopoletin by examining the induction of apoptosis in KKU-100 and KKU-M214 cells after exposure to scopoletin and cisplatin alone or in combination for 24 hours. The percentage of cell undergoing apoptosis upon treatment was determined based on detection of phosphatidylserine externalization by flow cytometry using annexin V-FITC staining. Consistent with sub-G1 group in cell cycle analysis, as can be seen in Figure 4, the combination of scopoletin with cisplatin induced more cells to undergo apoptosis than cisplatin or scopoletin either alone, which suggest additive interaction between the drugs in both cholangiocarcinoma cells. Cisplatin (10 µM) exposure to KKU-100 and KKU-M214 cells increased apoptosis to 14.3 ± 1.4% and 12.3 ± 0.6% and scopoletin (500 µM) produced 12.6 ± 0.7% and 10.8 ± 0.8%, respectively while combination of both agents led to 27.8 ± 1.1% and 23.3 ± 0.9% which are significantly higher than that of single drug controls.
Figure 4: Effect of cisplatin, scopoletin alone or in combination on apoptosis induction of CCA cells.(A, B) Representative dot plots display the apoptotic death of KKU-100 and KKU-M214 cells treated with the indicated agents. Cells were treated with DMSO (0.5%; v/v) as a solvent control, cisplatin alone (10 µM), scopoletin alone (500 µM) and combination of cisplatin (10 µM) and scopoletin (500 µM) for 24 hours, and analyzed for apoptosis induction using Annexin V-FITC/Propidium Iodide (PI) staining with flow cytometry. (C, D) Bar graphs show the summarized data from three independent experiments performed in duplicate. ap<0.05 for comparison between single drug and control treatments. bp<0.05 for comparison between the combinative treatment compared with single agent treatment
Our results comply with the several research findings where scopoletin, its derivatives and some natural coumarin are reported to induce apoptosis. It has been reported that scopoletin increased apoptosis in human prostate tumor PC3 cells (Liu et al., 2001) and in human leukemia cell line HL-60 (Kim et al., 2005). Scopoletin has been suggested being a possible candidate for chemoprevention as scopoletin treatment for carcinogen-induced skin papilloma in mice produced apoptosis by increasing apoptosis regulator p53 (Bhatta-charyya et al., 2010). Treatment with synthetic coumarin has unequivocally been demonstrated to induce up-regulation of p53 and apoptotic proteins Bax in DMBA-administered mice that favored apoptosis and antagonized tumor formation (Bhattacharyya et al., 2009). Antineoplastic action of scopoletin has been observed to be associated with increased apoptosis of HeLa cells in vitro (Bhattacharyya et al., 2008). Scopoletin remarkably induced apoptosis in a dose-dependent manner in fibroblast-like synoviocytes, which was accompanied by up-regulated Bax mRNA expression and down-regulated mRNA level of Bcl-2(Li et al., 2009). It has been documented that resveratrol inhibits growth of the KKU-100 and KKU-M156 cells by inducing mitochondrial-dependent apoptosis (Hahnvajanawong et al., 2011). Apoptosis was observed in adenocarcinomas with coumarin compounds. Coumarins in combination with other antineoplastic drugs might increase the effectiveness of NSCLC treatments (Lopez-Gonzalez et al., 2004). Noscapine and cisplatin combination caused higher percent of apoptotic cell death in H460 and A549 NSCLC cells in comparison to single agent treatment, reflecting synergistic interaction between noscapine and cisplatin (Chougule et al., 2011). Curcumin potentiated the antitumor and apoptotic effects of cisplatin on human hepatic cancer HA22T/VGH cells in a sequence dependent way (Notarbartoloa et al., 2005).
Conclusion
Taken together, it can be concluded that scopoletin can be used to improve the efficacy of cisplatin in cancer treatments.
Acknowledgements
This study was supported by the National Research Council of Thailand (through Khon Kaen University, Fiscal year 2014). We acknowledge Faculty of Science, Khon Kaen University, Thailand, for providing a graduate scholarship to Md. Ali Asgar. We would like to thank Prof. Nison Sattayasai, Coordinator of the Biological Science program, Khon Kaen University.
References
Aljiffry M, Walsh MJ, Molinari M. Advances in diagnosis, treatment and palliation of cholangiocarcinoma: 1990-2009. World J Gastroenterol. 2009; 15: 4240-62.
Bhattacharyya SS, Mandal SK, Biswas R, Paul S, Pathak S, Boujedaini N, Belon P, Khuda-Bukhsh AR. In vitro studies demonstrate anticancer activity of an alkaloid of the plant Gelsemiumsem pervirens. Exp Biol Med (Maywood). 2008; 233: 1591-601.
Bhattacharyya SS, Paul S, Dutta S, Boujedaini N, Khuda-Bukhsh AR. Antioncogenic potential of a plant coumarin (7-hydroxy-6- methoxy coumarin) against 7,12- dimethylbenzen[a]anthracene-induced skin papilloma in mice: The possible role of several key signal proteins. Chin J Integr Med. 2010; 8: 645-54.
Bhattacharyya SS, Paul S,Mandal SK, Banerjee A, Boujedaini N, Khuda-Bukhsh AR. A synthetic coumarin (4-methyl-7-hydroxy coumarin) has anticancer potentials against DMBA-induced skin cancer in mice. Eur J Pharmacol. 2009; 614: 128-36.
Bigioni M, Benzo A, Irrissuto C, Lopez G, Curatella B, Maggi CA, Manzini S, Crea A, Caroli S, Cubadda F, Binaschi M. Antitumour effect of combination treatment with sabarubicin (MEN 10755) and cisplatin (DDP) in human lung tumourxenograft. Cancer Chemother Pharmacol. 2008; 62: 621-29.
Cai X, Yang J, Zhou J, Lu W, Hu C, Gu Z, Huo J, Wang X, Cao P. Synthesis and biological evaluation of scopoletin derivatives. Bioorg Med Chem. 2013; 21: 84-92.
Chou TC, Talalay P. Quantitative analysis of dose-effect relationships: The combined effects of multiple drugs or enzyme inhibitors. Adv Enzyme Regul. 1984; 22: 27-55.
Chou TC. Theoretical basis, experimental design, and computerized simulation of synergism and antagonism in drug combination studies. Pharmacol Rev. 2006; 58: 621-81.
Chougule M, Patel AR, Sachdeva P, Jackson T, Singh M. Anticancer activity of noscapine, an opioid alkaloid in combination with cisplatin in human non-small cell lung cancer. Lung Cancer. 2011; 71: 271-82.
Chuang J-Y, Huang Y-F, Lu H-F, Ho H-C, Yang J-S, Li T-M, Chang N-W, Chung J-G. Coumarin induces cell cycle arrest and apoptosis in human cervical cancer HeLa cells through a mitochondria- and caspase-3 dependent mechanism and NF-κB down-regulation. In vivo. 2007; 21: 1003-10.
Gores GJ. Cholangiocarcinoma: Current concepts and insights. Hepatology 2003; 37: 961-69.
Haghighitalab A, Matin MM, Bahrami AR, Iranshahi M, Saeinasab M, Haghighi F. In vitro investigation of anti-cancer, cell-cycle-inhibitory, and apoptosis-inducing effects of diversin, a natural prenylated coumarin, on bladder carcinoma cells. Z Naturforsch C. 2014; 69: 99-109.
Hahnvajanawonga C, Ketnimita S, Boonyanugomola W, Pattanapanyasatd K, Chamgramole Y, Sripae B, Namwatf N, Pinmaig K, Tassaneeyakulh W, Reutrakuli V. Inhibition of cell cycle progression and apoptotic activity of resveratrol in human intrahepatic cholangiocarcinoma cell lines. Asian Biomed. 2011; 5: 775-85.
Kang TH, Pae HO, Jeong SJ, Yoo JC, Choi BM, Jun CD, Chung HT, Miyanoto T, Higuchi R, Kim YC. Scopoletin: An inducible nitric oxide synthesis inhibitory active constituent from Artemisia feddei. Planta Med. 1999; 65: 400-03.
Kim E-K, Kwon K-B, Shin B-C, Seo E-A, Lee Y-R, Kim J-S, Park J-W, Park B-H, Ryu D-G. Scopoletin induces apoptosis in human promyeloleukemic cells, accompanied by activation of nuclear factor ƙB and caspase-3. Life Sci. 2005; 77: 824-36
Kuhlmann JB. Treatment of advanced cholangiocarcinoma: Current status and future perspectives. EMJ Gastroenterol. 2012; 1: 63-7.
Li C-J, Chu C-Y, Huang L-H, Wang M-H, Sheu L-F, Yeh J-I, Hsu H-Y. Synergistic anticancer activity of triptolide combined with cisplatin enhances apoptosis in gastric cancer in vitro and in vivo. Cancer Lett. 2012; 319: 203-13.
Li Y, Dai Y, Liu M, Pan R, Luo Y, Xia Y, Xia X. Scopoletin induces apoptosis of fibroblast-like synoviocytes from adjuvant arthritis rats by a mitochondrial-dependent pathway. Drug Develop Res. 2009; 70: 378-85.
Liu X-L, Zhang L, Fu X-L, Chen K, Qian B-C. Effect of scopoletin on PC3 cell proliferation and apoptosis. Acta Pharmacol Sin. 2001; 22: 929-33.
Liu W, Hua J, Zhou J, Zhang H, Zhu H, Cheng Y, Gust R. Synthesis and in vitro antitumor activity of novel scopoletin derivatives. Bioorg Med Chem Lett. 2012; 22: 5008-12.
Lopez-Gonzalez JS, Prado-Garcia H, Aguilar-Cazares D, Molina-Guarneros JA, Morales-Fuentes J, Mandoki JJ. Apoptosis and cell cycle disturbances induced by coumarin and 7-hydroxycoumarin on human lung carcinoma cell lines. Lung Cancer. 2004; 43: 275-83.
Moon PD, Lee BH, Jeong HJ, An HJ, Park SJ, Kim HR, Ko SG, Um JY, Hong SH, Kim HM. Use of scopoletin to inhibit the production of inflammmatory cytokines through inhibition of the NF-kappa B signal cascade in the human mast cell line HMC-1. Eur J Pharmcol. 2007; 555: 218-25.
Notarbartoloa M, Pomaa P, Perria D, Dusoncheta L, Cervellob M, D’Alessandro N. Antitumor effects of curcumin, alone or in combination with cisplatin or doxorubicin, on human hepatic cancer cells. Analysis of their possible relationship to changes in NF-kB activation levels and in IAP gene expression. Cancer Lett. 2005; 224: 53- 65.
Pan MH, Ho CT. Chemopreventive effects of natural dietary compounds on cancer development. Chem Soc Rev. 2008; 37: 2558-74.
Peczuh MW, Hamilton AD. Peptide and protein recognition by designed molecules. Chem Rev. 2000; 100: 2479-94.
Peterson JK, Harrison HF, Jackson DM. Biological activities and contents of scopolin and scopoletin in sweet potato clones. Hort Sci. 2003; 38: 1129-33.
Prakobwong S, Gupta S C, Kim JH, Sung B, Pinlaor P, Hiraku Y, Wongkham S, Sripa B, Pinlaor S, Aggarwal BB. Curcumin suppresses proliferation and induces apoptosis in human biliary cancer cells through modulation of multiple cell signaling pathways. Carcinogenesis 2011; 32: 1372-80.
Roncoroni L, Elli L, Dolfini E, Erba E, Dogliotti E, Terrani C,Doneda L, Grimoldi MG, Bardella MT. Resveratrol inhibits cell growth in a human cholangiocarcinoma cell line. Liver Int. 2008; 28: 1426-36.
Saha SK, Khuda-Bukhsh AR. Molecular approaches towards development of purified natural products and their structurally known derivatives as efficient anticancer drugs: Current trends. Eur J Pharmcol. 2013; 714: 239-48.
Sato T, Suzuki M, Sato Y, Echigo S, Rikiishi H. Sequence-dependent interaction between cisplatin and histone deacetylase inhibitors in human oral squamous cell carcinoma cells. Int J Oncol. 2006; 28: 1233-41.
SenawongT, Misuna S, Khaopha S, Nuchadomrong S, Sawatsitang P, Phaosiri C, Surapaitoon A, Sripa B. Histone deacetylase (HDAC) inhibitory and antiproliferative activities of phenolic-rich extract derived from The rhizome of Hydnophytumformicarum Jack.: Sinapinic acid acts as HDAC inhibitor. BMC Complement Altern Med. 2013; 13: 232.
Siddik ZH. Cisplatin: Mode of cytotoxic action and molecular basis of resistance. Oncogene. 2003; 22: 7265-79.
Thongprasert S. The role of chemotherapy in cholangiocarcinoma. Ann Oncol. 2005; 16 (Suppl 2): ii93–96.
Tikoo K, Shinde, AB, Gupta C, Jena G. Modulation of p53 â„Aktâ„ phosphatase and tensin homolog expression by esculetin potentiates the anticancer activity of cisplatin and prevents its nephrotoxicity. Cancer Sci. 2011; 103: 1-8.
Wang G, Reed E, Li QQ. Molecular basis of cellular response to cisplatin chemotherapy in non-small cell lung cancer (Review). Oncol Rep. 2004; 12: 955-65.
Zhou J, Lei W, Lijuan W, Yu Z, Huibin Z, Yubin W, Peng C, Ao N, Jing W, Yue D. Synthesis and antitumor activity of scopoletin derivatives. Lett Drug Des Discov. 2012; 9: 397-401.
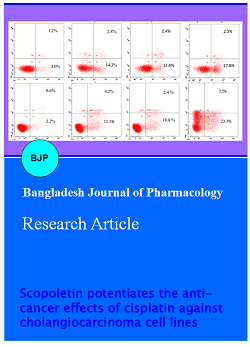