In vitro studies on the antimicrobial, antioxidant and antidiabetic potential of Cephalaria gigantea
Abstract
This study evaluates the antimicrobial, antioxidant and antidiabetic potential of leaf and root extracts of Cephalaria gigantea. The results showed that the extracts inhibited both bacteria and fungi tested at MIC values which ranged from 3.1 to 12.5 mg/mL. The root water and leaf hydro-ethanol extracts had the highest content of phenolics exhibiting IC50 values of 310.9 mg/g and 275.2 mg/g respectively. The leaf water extract possessed the highest amount of flavonoids with IC50 value of 145.4 mg/g followed by root hydro-ethanol extract with IC50 value of 53.4 mg/g. The leaf and root water extracts had the best DPPH radical scavenging activity with IC50 values of 0.6 µg/mL and 2.8 µg/mL compared to gallic acid at 14.0 µg/mL. The leaf hydro-ethanol and root water extracts scavenged ABTS radical best. Also the leaf and root hydro-ethanol extract exhibited the best reducing power activity when compared to gallic acid. The root water and leaf ethanol extracts displayed the most potent inhibition of α-amylase and α-glucosidase respectively. It can be concluded that C. gigantea extracts possessed antioxidant and antidiabetic potentials but has poor antimicrobial activities.
Introduction
The use of herbs as alternative medicine had continue to increase over the last two decades (Rosenbloom et al, 2011) and World Health Organization (WHO) asserted that about 80% of world’s population relies on traditional medicine for their primary healthcare needs (Ajose, 2007). South Africans, like most developing countries of the world, rely on traditional medicine due to the fact that they are easily accessible and affordable by all (Isaac et al., 2011). Medicinal plants are also used in treatment of many ailments because they are believed to have little or no adverse effects, unlike the synthetic drugs that may produce several side effects (Said et al., 2002). These plants whose different pharmacological potentials vary (antioxidant, anti-inflammatory, antimicrobial, anthelmintic, etc) are used by traditional healers in the treatment and management of several diseases.
Cephalaria gigantea (Dipsacaceae) is native to southern Europe, western and central Asia as well as northern and southern Africa (Kayce and Kirmizigul, 2010). This perennial plant has stout, upright stems which are tall but airy and “see-through’’ arising from a rosette of divided leaves, and when in flower, the pretty pale butter-yellow scabious shape flowers can reach 180 cm (6 feet). Species from Cephalaria have been used as folk medicines for their anti-infectant, hypothermic and relaxant activities (Gerkens et al., 2007). C. gigantea is used in traditional medicine as sedative and anti-inflammatory remedies (Tabatadze et al., 2007). They contain a great variety of compounds with pharmaceutical activity such as alkaloids, iridoids, flavonoids and triterpenoid saponins (Caliskan et al., 1994). Several triterpene saponins and flavonoids have been isolated from this plant which includes giganteosides D and E (Mshvildadze et al., 2001; Movsumov et al., 2006). Some of these compounds showed interesting antileishmanial, antifungal and antiprotozoal activities
Despite the wide usage of this plant in traditional medicine, there is dearth of information on the scientific evaluation of its biological activities. This study therefore, aimed at evaluating the in vitro antimicrobial, antioxidant and antidiabetic potential of C. gigantea.
Materials and Methods
Plant collection and identification
The plant was collected from multiple populations in November 2013 within the premises of the University of the Free State, Qwaqwa campus and was identified by Ms H Mtshali of the department of plant sciences, University of the Free State. Herbarium specimen Ashmed 05/QHb was deposited at the Qwaqwa herbarium. The shoot and root were separated and rinsed to remove debris before drying at 40°C in an Echotherm oven and subsequently ground into fine powder and store at 4°C.
Preparation of extracts
10 g of both powdered leaves and roots of C. gigantea were extracted in acetone, ethanol, methanol, hydro-ethanol, and distilled water respectively and placed on Labcon Platform shaker (Laboratory Consumables, PTY, Durban, South Africa) for 24 hours. All extracts were filtered using Whatman No. 1 filter paper. The filtrates from acetone, ethanol, and methanol were concentrated under reduced pressure at 40°C using rotary evaporator (Cole Parmer SB 1100, Shangai, China), while water and hydro-alcohol extracts were freeze dried using Virtis BenchTop (SP Scientific Series, USA) freeze dryer. The acetone, ethanol, methanol, hydro-ethanol and water extracts yielded 0.5, 0.7, 2.4, 4.2, 3.5 g and 0.4, 2.2, 3.8, 5.1, and 1.7 g for leaf and root respectively.
Chemicals and reagents
Porcine pancreatic α-amylase, rat intestinal α-glucosidase, 1,1-diphenyl–2 picrylhydrazyl, gallic acid, acarbose and paranitrophenyl-glucopyranoside were products of Sigma-Adrich Co., St Louis, USA while starch soluble (extra pure) was obtained from J. T. Baker Inc., Phillipsburg, USA. Other chemicals and reagents were of analytical grade and the water used was glass-distilled.
Test microorganisms
Four Gram-positive bacteria: Staphylococcus aureus (ATCC 6538), S. aureus (OK2a), S. aureus (OK2b), Streptococcus faecalis; eight Gram-negative bacteria: Escherichia coli (ATCC 8739), Shigella sonnei (ATCC 29930), Shigella flexneri (KZN), Klebsiella pneumoniae (ATCC 13047), Klebsiella pneumoniae, Pseudomonas aeruginosa, Salmonella typhi, Salmonella typhimurium and four fungal strains: Candida rugosa, C. neoformans, C. albicans, and Trichophyton mucoides were used to investigate the antimicrobial activity of C. gigantea. These organisms were obtained from the Department of Biochemistry and Microbiology, University of Fort Hare, South Africa, and were maintained on nutrient broth (Oxoid LTD, Basingstoke, Hampshire, England) for 24 hours before being used.
Antimicrobial activity
The Minimum Inhibitory Concentration (MIC) values for the extracts and fractions against microorganisms (both bacteria and fungi) were determined by using twoÂfold serial microdilution method as described by (Eloff, 1998). Organisms were maintained on the nutrient agar at 4°C in the refrigerator and were revived for bioassay by sub-culturing in fresh nutrient broth (Oxoid Ltd, Basingstoke, Hampshire, England) 24 hours before use. Briefly, organisms were cultured overnight (24 hours) in an autoclaved Nutrient Broth (Oxoid LTD, Basindstoke, Hampire, England) and was adjusted to a final density of 10 µL/mL. This was used to inoculate 96Âwell microtiter plates containing serial two fold dilutions of the extracts (12.5Â0.1 mg/mL) under aseptic condition. The plates were incubated under aerobic conditions at 37°C and examined after 24 hours. Controls were prepared from extract free microtiter plates. 40 µL of 0.2 mg/mL p-iodonitrotetrazolium (97% purity, Sigma, South Africa) solution was added to each well as an indicator of microbial growth and incubated for 30 min at 37°C. Tetrazolium salt was reduced to colored (red) product which is an indication of organism growth. Therefore, red colored wells indicated presence of organisms, while clear wells indicated inhibition of organisms. Each treatment was performed in duplicate.
Determination of total phenol content
The total phenol content was determined according to the method of Singleton et al. (1999). Briefly, appropriate dilution of the extracts was oxidized with 2.5 mL 10% Folin–Ciocalteau’s reagent (v/v) and neutralized by 2.0 mL of 7.5% Na2CO3. The reaction mixture was incubated for 40 min at 45°C, and the absorbance was measured at 765 nm in the spectrophotometer (Biochrom WPA Biowave II, Cambridge, England). Gallic acid was used as standard phenol, and the total phenol content was subsequently calculated as gallic acid equivalent.
Determination of total flavonoid content
The total flavonoid content of the extracts were determined using a slightly modified method reported by Meda et al, (2005). Briefly, 0.5 mL of appropriately diluted sample was mixed with 0.5 mL methanol, 50 mL of 10% AlCl3, 50 mL of 1M potassium acetate and 1.4 mL H2O, and allowed to incubate at room temperature for 30 min. Thereafter, the absorbance of the reaction mixture was subsequently measured at 415 nm. Quercetin was used as standard flavonoid, and the total flavonoid content was calculated as quercetin equivalent.
DPPH free radical scavenging ability
The free radical scavenging ability of the extracts against DPPH (1,1-diphenyl–2 picrylhydrazyl) free radical was evaluated as described by Gyamfi et al. (1999). Briefly, an appropriate dilution of the extracts (1 mL) was mixed with 1 mL of 0.4 mmol/L methanolic solution containing DPPH radicals. The mixture was left in the dark for 30 min and the absorbance was measured at 516 nm. The DPPH free radical scavenging ability was subsequently calculated with respect to the reference (which contains all the reagents without the test sample).
Determination of reducing property
The reducing property of the extracts was determined by assessing the ability of the extract to reduce FeCl3 solution as described by Oyaizu (1986). A 2.5 mL aliquot of the extracts was mixed with 2.5 mL of 200 mmol/L sodium phosphate buffer (pH 6.6) and 2.5 mL of 1% potassium ferricyanide. The mixture was incubated at 50°C for 20 min and then 2.5 mL of 10% trichloroacetic acid was added. This mixture was centrifuged at 650 rpm for 10 min. 5 mL of the supernatant was mixed with an equal volume of water and 1 mL of 0.1% ferric chloride. The absorbance was measured at 700 nm. The ferric reducing antioxidant property was subsequently calculated using ascorbic acid as standard.
ABTS radical scavenging ability
The assay was performed according to the method described by Singleton et al. (1999). The ABTS+ was generated by reacting 7 mM ABTS aqueous solution with K2S2O8 (2.45 mM, final concentration) in the dark for 16 hours and adjusting the absorbance 734 nm to pH 0.700 with ethanol. 0.2 mL of appropriate dilution of the extract was added to 2.0 mL ABTS+ solution and the absorbance were measured at 734 nm after 15 min. The trolox equivalent antioxidant capacity (TEAC) was subsequently calculated.
α-Amylase inhibitory assay
This assay was carried out using a modified procedure of McCue and Shetty (2004). A total of 250 μL of extract was placed in a test tube and 250 μL of 0.02 M sodium phosphate buffer (pH 6.9) containing α-amylase solution was added. This solution was pre-incubated at 25°C for 10 min, after which 250 μL of 1% starch solution in 0.02 M sodium phosphate buffer (pH 6.9) was added at timed intervals and then incubated at 25°C for 10 min. The reaction was terminated by adding 500 μL of dinitrosalicylic acid (DNS) reagent. The tubes were then incubated in boiling water for 5 min and cooled to room temperature. The reaction mixture was diluted with 5 mL distilled water and the absorbance was measured at 540 nm using a spectrophotometer (Spectrumlab S23A, Globe Medical England). The control and blank were prepared using the same procedure replacing the extract with DMSO and distilled water respectively. The α-amylase inhibitory activity was calculated as percentage inhibition, thus;
% Inhibition = [(ΔAcontrol-ΔAextract)/AΔcontrol] x 100
Mode of α-amylase inhibition
The mode of inhibition of α-amylase by the C. gigantea extracts was conducted using the extract with the lowest IC50 according to the modified method described by Ali et al. (2006). Briefly, 250 μL of the (5 mg/mL) extract was pre-incubated with 250 μL of α-amylase solution for 10 min at 25°C in one set of tubes. In another set of tubes α-amylase was pre-incubated with 250 μL of phosphate buffer (pH 6.9). 250 μL of starch solution at increasing concentrations (0.3–5.0 mg/mL) was added to both sets of reaction mixtures to start the reaction. The mixture was then incubated for 10 min at 25°C, and then boiled for 5 min after addition of 500 μL of DNS to stop the reaction. The amount of reducing sugars released was determined spectrophotometrically using a maltose standard curve and converted to reaction velocities. A double reciprocal (Lineweaver-Burk) plot (1/v versus 1/[S]) where v is reaction velocity and [S] is substrate concentration was plotted to determine the mode of inhibition.
α-Glucosidase inhibitory assay
The effect of the plant extracts on α-glucosidase activity was determined according to the method described by Kim et al. (2005). The substrate solution, p-nitropheynyl glucopyranoside (pNPG) was prepared in 20 mM phosphate buffer, pH 6.9. 100 μL of α-glucosidase (E.C. 3.2.1.20) was pre-incubated with 50 μL of the different concentrations of the extracts for 10 min. Then 50 μL of 3.0 mM pNPG dissolved in 20 mM phosphate buffer (pH 6.9) was added to start the reaction. The reaction mixture was incubated at 37°C for 20 min and stopped by adding 2 mL of 0.1 M Na2CO3. The α-glucosidase activity was determined by measuring the yellow colored paranitrophenol released from pNPG at 405 nm. The control and blank were prepared using the same procedure by replacing the extract with DMSO and distilled water respectively. Percentage inhibition was calculated thus;
% Inhibition = [(ΔAcontrol-ΔAextract)/AΔcontrol] x 100
Concentrations of extracts resulting in 50% inhibition of enzyme activity (IC50) were determined graphically
Mode of α-glucosidase inhibition
The mode of inhibition of α-glucosidase by the extracts was determined using the extract with the lowest IC50 according to the modified method described by Ali et al. (2006). Briefly, 50 μL of the (5 mg/mL) extract was pre-incubated with 100 μL of α-glucosidase solution for 10 min at 25°C in one set of tubes. In another set of tubes, α-glucosidase was pre-incubated with 50 μL of phosphate buffer (pH 6.9). 50 μL of PNPG at increasing concentrations (0.6–2.0 mg/mL) was added to both sets of reaction mixtures to start the reaction. The mixture was then incubated for 10 min at 25°C and 500 μL of Na2CO3 was added to stop the reaction. The amount of reducing sugars released was determined spectrophotometrically using a paranitrophenol standard curve and converted to reaction velocities. A double reciprocal (Lineweaver-Burk) plot (1/v versus 1/[S]) where v is reaction velocity and [S] is substrate concentration was plotted to determine the mode of inhibition.
Statistical analysis
Statistical analysis was performed using GraphPad Prism 5 statistical package (GraphPad Software, USA). The data were analysed by one way analysis of variance (ANOVA) followed by Bonferroni test. All the results were expressed as mean ± SEM for triplicate determinations.
Results
Tables I and II showed the result of antimicrobial activity of different extracts of C. gigantea on different microorganisms. The ethanol leaf extract showed the best inhibition against bacteria with minimum inhibitory concentration (MIC) between 1.6 to 3.1 mg/mL. This inhibition was observed against Escherichia coli (ATCC 8739), Klebsiella pneumoniae (ATCC 13047), Salmonella typhi and Streptococcus faecalis. Acetone, methanol, ethanol, and hydro-ethanol leaf extracts showed better inhibition against fungi at 3.1 to 6.2 mg/mL. Both the standard antifungal (fluconazole) and antibacterial (ciprofloxacin) agents showed better inhibition against all microbes compared to the extracts.
Table I: Antibacterial and antifungal activity of Cephalaria leaf extracts showing minimal inhibitory concentrations
Organisms | Gram | Extracts (mg/mL) | Ciprofloxacin | ||||
---|---|---|---|---|---|---|---|
Bacteria | Acetone | Methanol | Ethanol | Water | H-E | ||
Escherichia coli ATCC 8739 | - | 3.1 | 3.1 | 1.6 | 3.1 | 6.2 | <0.08 |
Klebsiella pneumoniae ATCC 13047 | - | 6.2 | 3.1 | 3.1 | 6.2 | 12.5 | <0.08 |
Klebsiella pnuemoniae | - | 6.2 | 3.1 | 1.6 | 6.2 | 12.5 | <0.08 |
Pseudomonas aeruginosa | - | 3.1 | 6.2 | 3.1 | 6.2 | 3.1 | <0.08 |
Salmonella typhi | - | 3.1 | 6.2 | 1.6 | 6.2 | 12.5 | <0.08 |
Salmonella typhimurium | - | 3.1 | 12.5 | 3.1 | 6.2 | 12.5 | <0.08 |
Shingella flexneri KZN | - | 6.2 | 3.1 | 3.1 | 6.2 | 12.5 | <0.08 |
Shingella sonnei ATCC 29930 | - | 3.1 | 3.1 | 3.1 | 6.2 | 12.5 | <0.08 |
Staphylococcus aereus OK2a | + | 3.1 | 3.1 | 3.1 | 6.2 | 12.5 | <0.08 |
Staphylococcus aereus OK2b | + | 12.5 | 3.1 | 3.1 | 6.2 | 12.5 | <0.08 |
Staphylococcus aureus ATCC 6538 | + | 3.1 | 3.1 | 3.1 | 6.2 | 12.5 | <0.08 |
Streptococcus faecalis | + | 6.2 | 6.2 | 1.6 | 6.2 | 3.1 | <0.08 |
Fungi | Fluconazole | ||||||
Candida rugosa | 3.1 | 3.1 | 3.1 | 3.1 | 6.2 | 1.6 | |
Candida neoformans | 6.2 | 3.1 | 6.2 | 3.1 | 6.2 | <0.08 | |
Candida albicans | 3.1 | 3.1 | 6.2 | 3.1 | 6.2 | 1.6 | |
Trichophyton mucoides | 3.1 | 6.2 | 3.1 | 6.2 | 6.2 | <0.08 |
Table II: Antibacterial and antifungal activity of Cephalaria root extracts showing minimal inhibitory concentrations
Organisms | Gram | Extracts (mg/mL) | Ciprofloxacin | ||||
---|---|---|---|---|---|---|---|
Bacteria | Acetone | Methanol | Ethanol | Water | H-E | ||
Escherichia coli ATCC 8739 | - | 12.5 | 12.5 | 12.5 | 12.5 | 12.5 | <0.08 |
Klebsiella pneumoniae ATCC 13047 | - | 12.5 | 6.2 | 12.5 | 12.5 | 12.5 | <0.08 |
Klebsiella pnuemoniae | - | 12.5 | 6.2 | 12.5 | 12.5 | 12.5 | <0.08 |
Pseudomonas aeruginosa | - | 12.5 | 6.2 | 12.5 | 12.5 | 12.5 | <0.08 |
Salmonella typhi | - | 12.5 | 6.2 | 12.5 | 12.5 | 12.5 | <0.08 |
Salmonella typhimurium | - | 12.5 | 6.2 | 12.5 | 12.5 | 12.5 | <0.08 |
Shingella flexneri KZN | - | 12.5 | 6.2 | 12.5 | 12.5 | 12.5 | <0.08 |
Shingella sonnei ATCC 29930 | - | 12.5 | 6.2 | 12.5 | 12.5 | 12.5 | <0.08 |
Staphylococcus aereus OK2a | + | 12.5 | 6.2 | 12.5 | 12.5 | 12.5 | <0.08 |
Staphylococcus aereus OK2b | + | 12.5 | 6.2 | 12.5 | 12.5 | 12.5 | <0.08 |
Staphylococcus aureus ATCC 6538 | + | 12.5 | 12.5 | 12.5 | 12.5 | 12.5 | <0.08 |
Streptococcus faecalis | + | 12.5 | 6.2 | 12.5 | 12.5 | 12.5 | <0.08 |
Fungi | Fluconazole | ||||||
Candida rugosa | 12.5 | 12.5 | 3.1 | 12.5 | 12.5 | 1.6 | |
Candida neoformans | 12.5 | 12.5 | 3.1 | 12.5 | 12.5 | <0.08 | |
Candida albicans | 12.5 | 12.5 | 3.1 | 12.5 | 12.5 | 1.6 | |
Trichophyton mucoides | 12.5 | 12.5 | 3.1 | 12.5 | 12.5 | <0.08 |
The root extracts showed poor inhibition against most of the bacteria strains, but the ethanol extract was active against most of the fungi strains at the MIC of 3.1 mg/mL.
Tables III depicted the phenolics and flavonoid composition of different extracts of C. gigantea leaves and root. The hydro-ethanol extract of the leaf possessed the highest amount of phenolics (275.2 mg/g) while the water extract had the highest quantity of flavonoids (145.4 mg/g). The phenolic content of the hydro-ethanol extract was not significantly different from the water extract but the flavonoid content of the water extract was significantly different (p<0.05) from all other extracts. Conversely, the water extract of the root possessed the greatest quantity of phenolics (310.9 mg/g) while the hydro-ethanol extract had the largest amount of flavonoids (53.4 mg/g), compared to all other extracts.
Table III: Total phenolics and flavonoids composition of Cephalaria gigantea leaf and root extracts
Extract | IC50 (mg/g) | |||
---|---|---|---|---|
Leaf | Root | |||
Phenolics | Falvonoids | Phenolics | Falvonoids | |
Acetone | 104.6 ± 2.1a | 27.8 ± 0.2a | 306.8 ± 6.0a | 16.1 ± 0.0a |
Methanol | 198.1 ± 4.0b | 77.8 ± 2.1b | 269.6 ± 4.2b | 21.5 ± 0.5b |
Ethanol | 88.0 ± 0.9c | 34.4 ± 0.5a | 293.6 ± 4.0a | 14.2 ± 0.1a |
Water | 227.2 ± 4.2d | 145.4 ± 4.0c | 310.8 ± 6.5a | 24.9 ± 0.3b |
Hydro-ethanol | 275.2 ± 3.5d | 103.4 ± 3.0d | 203.8 ± 5.0c | 53.4 ± 1.0c |
Table IV showed the result of antioxidant activities of different extracts of C. gigantea leaf. Water extract has the lowest IC50 (0.6 µg/mL) in the DPPH assay, which is significantly different (p<0.05) compared to the standard (gallic acid) and other extracts. Hydro-ethanol extract possess the lowest IC50 for both ABTS radical scavenging and reducing activity. Its value for ABTS assay (1.0 µg/mL) is significantly different (p<0.05) to other extracts but comparable to gallic acid while the value for the reducing property (1.7 µg/mL) is significantly different (p<0.05) to other extracts and gallic acid. Water extract from the root of C. gigantea also exhibited the lowest IC50 (2.8 µg/mL) for the DPPH assay which is significantly different (p < 0.05) to other exracts and standard (Table III). Acetone extract displayed significantly reduced IC50 (2.8 µg/mL) compared to other samples in the ABTS assay while hydro-ethanol extract had the lowest IC50 (2.1 µg/mL) in terms of reducing property, and are comparable to the values for ethanol and methanol extracts.
Table IV: Antioxidant activity of different extracts from the leaf and root of Cephalaria gigantea
Extract | IC50 (µg/mL) | |||||
---|---|---|---|---|---|---|
Leaf | Root | |||||
DPPH | ABTS | Reducing power | DPPH | ABTS | Reducing power | |
Acetone | 27.0 ± 0.3a | 7.8 ± 0.0a | 16.7 ± 2.2a | 117.0 ± 3.8a | 2.8 ± 0.0a | 5.0 ± 0.3a |
Methanol | 6.3 ± 0.0b | 4.4 ± 0.0b | 3.7 ± 1.3b | 123.0 ± 2.2a | 8.6 ± 0.0b | 3.1 ± 0.0b |
Ethanol | 5.6 ± 0.0b | 5.0 ± 0.0b | 7.0 ± 0.5c | 539.0 ± 9.1b | 11.0 ± 0.0b | 2.2 ± 0.0b |
Water | 0.6 ± 0.0c | 2.8 ± 0.0c | 3.0 ± 0.1b | 2.8 ± 0.9c | 4.4 ± 0.0c | 11.6 ± 0.3c |
Hydro-ethanol | 3.6 ± 0.0d | 1.0 ± 0.0d | 1.7 ± 0.1d | 34.0 ± 0.8d | 6.4 ± 0.0c | 2.0 ± 0.0b |
Gallic acid | 14.0 ± 0.4e | 1.5 ± 0.0d | 12.2 ± 2.0a | 14.0 ± 0.4e | 1.5 ± 0.0d | 12.2 ± 2.0c |
The result of inhibition of α-amylase and α-glucosidase by the leaf and root extracts of C. gigantea are presented in Table V. Methanol and ethanol leaf extracts of the plant exhibited the lowest IC50 for α-amylase (0.3 mg/mL) and α-glucosidase (0.2 mg/mL) respectively. These values are significantly different (p<0.05) when compared to other extracts. The ethanol root extract of the plant also exhibited the lowest IC50 (0.5 mg/mL) for the inhibition of α-amylase, which is comparable to the standard, acarbose. However, hydro-ethanol extract of the root displayed the lowest IC50 (0.3 mg/mL) for the inhibition of α-glucosidase and is significantly different (p<0.05) to other extracts and standard. Figures 1 and 2 showed the mode of inhibition of α-amylase and α-glucosidase by the root water and leaf ethanol extracts respectively. The root water extract inhibited α-amylase in a non-competitive manner while root water extracts inhibited α-glucosidase competitively.
Figure 1: Mode of inhibition of α-amylase by water extract of Cephalaria gigantea root
Figure 2:Mode of inhibition of α-glucosidase by ethanol extract of Cephalaria gigantea leaf
Table V: α-Amylase and α-glucosidase inhibitory activity of Cephalaria gigantea leaf and root extracts
Extract | IC50 (mg/mL) | |||
---|---|---|---|---|
Leaf | Root | |||
α-Amylase | α-Glucosidase | α-Amylase | α-Glucosidase | |
Acetone | 0.5 ± 0.0a | 0.4 ± 0.0a | 3.4 ± 0.1a | 0.9 ± 0.0a |
Methanol | 0.3 ± 0.0c | 0.2 ± 0.0b | 3.0 ± 0.0b | 0.7 ± 0.0b |
Ethanol | 0.8 ± 0.0b | 0.2 ± 0.0b | 0.5 ± 0.0c | 0.7 ± 0.0b |
Water | 0.4 ± 0.0c | 0.7 ± 0.0c | 1.1 ± 0.0d | 1.6 ± 0.0c |
Hydro-ethanol | 0.6 ± 0.0a | 0.4 ± 0.0a | 1.2 ± 0.0d | 0.3 ± 0.0d |
Acarbose | 0.5 ± 0.0a | 0.0 ± 0.0d | 0.5 ± 0.0c | 0.0 ± 0.0e |
Discussion
The antimicrobial study on different extracts of C. gigantea showed that the root extracts of the plant does not possess antibacterial activity while only the ethanol extract exhibit antifungal activities. This is an indication that the root of this plant may not be suitable for use as an antibacterial agent. Acetone, methanol and ethanol leaf extracts of C. gigantea displayed moderate antimicrobial potential compared to the other extracts. This may be due to qualitative and quantitative differences in the phytochemical composition of the extracts (Geyyid et al., 2005). The observed reduced inhibition of microorganisms by the water and hydro-ethanol extracts of C. gigantea leaf may not be unconnected with the fact the active antimicrobial agents in the plant may be non-polar (Mabona and Van Vuuren, 2013).
We found out that root extracts of C. gigantea is richer in phenolics than the leaf extracts while the leaf is richer in flavonoids than the root. These results also indicated that the water and hydro-ethanol extracts of both leaf and root of C. gigantea contained the highest amounts of both phenolics and flavonoids respectively. Phenolics are widespread naturally-occurring constituents of plants (Weichselbaum and Buttriss, 2010) and flavonoids also belong to one of the groups of phenolics. Studies have shown that the antiradical activity of flavonoids and phenolics is principally based on the structural relationship between different parts of their chemical structure (Rice-Evans et al., 1996). Natural polyphenols have the ability to remove free radicals, chelate metal catalysts, activate antioxidant enzymes and inhibit oxidases (Amic et al., 2003; Alia et al., 2003).
The antioxidant activities of plant phytochemicals occur by preventing the production of free radicals or by neutralizing/scavenging free radicals produced in the body or reducing/chelating the transition metal composition of biomolecules (Oboh et al., 2007). The water extracts of both the leaf and root displayed the best scavenging activity of DPPH radicals. This depicted by its lowest IC50 compared to the other extracts. The phenolic compounds present in the extracts could be responsible for the observed DPPH radical scavenging activity, since phenols can readily donate hydrogen atom to the radical (Tung et al., 2009). DPPH is frequently used in the determination of free radical scavenging ability; however, it has the limitation of color interference and sample solubility (Dorman et al., 2004). Therefore, the free radical scavenging ability of the leaf and root extracts was further studied using a moderately stable nitrogen-centered radical species: ABTS radical. The leaf water extract also scavenged the ABTS radical best while acetone extract displayed the best activity for the root. Therefore, the result of the inhibitory activities of the leaf extracts on ABTS radical followed a similar trend as that obtained from inhibition of DPPH radical. These activities may not be unconnected with the phytochemical (phenolics and flavonoids) composition of the extracts.
Reducing power is a potent antioxidant defense mechanism; the two mechanisms that are available to effect this property are electron transfer and hydrogen atom transfer (Dastmalchi et al., 2007). The hydro-ethanol extracts of both leaf and root had higher reducing ability compared to other extracts. This result is in agreement with the total phenolic and flavonoid content of the extracts. The reducing power of the extracts was assessed based on their ability to reduce Fe3+ to Fe2+. This is because the ferric-to-ferrous iron reduction occurs rapidly with all reductants with half reaction reduction potentials above that of Fe3+/Fe2+, the values in the reducing power assay will express the corresponding concentration of electron donating antioxidants (Halvorsen et al., 2002).
Inhibition of enzymes involved in the hydrolysis of carbohydrates such as α-amylase and α -glucosidase has been exploited as therapeutic approaches for controlling postprandial hyperglycemia (Shim et al., 2003). Pancreatic α-amylase is involved in the breakdown of starch into disaccharides and oligosaccharides while intestinal α-glucosidase catalyzes the breakdown of disaccharides to liberate glucose, which is later absorbed into the blood circulation. Inhibition of these enzymes would slow down the breakdown of starch in the gastrointestinal tract, thus reducing postprandial hyperglycemia (Kwon et al., 2007). Out of all the extracts tested, methanol leaf extract inhibited α-amylase best and so had the lowest IC50 while ethanol root extract also possessed the highest IC50. Since part of the drawbacks of synthetic antidiabetic agents like acarbose is due to the excessive inhibition of α-amylase, suitable alternatives should be mild inhibitor of the enzyme (Kazeem et al., 2013). Therefore, water or hydro-ethanol root extracts of the plant may be suitable agents as they possessed IC50 that doubles that of acarbose. Methanol leaf extracts of C. gigantea had the strongest inhibition of α-glucosidase, which culminated in it having the lowest IC50 out of all the extracts.
The non-competitive inhibition of α-amylase by the root water extract of the plant implies that the active components in the extract binds to a site other than the active site of the enzyme and combines with either free enzyme or the enzyme-substrate complex, possibly interfering with the action of both (Berg et al., 2002). However, the inhibitory components of the extract have equal affinity for the enzyme and enzyme-substrate complex. On the other hand, the competitive inhibition of α-glucosidase by the leaf ethanol extract suggests that the active inhibitory component(s) of the extract are structurally similar to the normal substrate of the enzyme (Shai et al., 2010). Therefore, it binds reversibly to the active site of the enzyme and occupies it in a mutually exclusive manner with the substrate
Conclusion
Although, the extracts exhibit weak activity against most microorganisms tested in this study, yet, it can be concluded that C. gigantea extracts possessed potent antioxidant and anti-diabetic potentials.
References
Ajose FO. Some Nigerian plants of dermatologic importance. Int J Dermatol. 2007; 46: 48-55.
Ali H, Houghton PJ, Soumyanath A. Alpha-amylase inhibitory activity of some Malaysian plants used to treat diabetes with particular reference to Phyllanthus amarus. J Ethnopharmacol. 2006; 107: 449-55.
Alia M, Horcajo C, Bravo L, Goya L. Effect of grape antioxidant dietary fiber on the total antioxidant capacity and the activity of liver antioxidant enzymes in rats. Nutr Res. 2003; 23: 1251-67.
Amic D, Davidovic-Amic D, Beslo D, Trinajstic N. Structure–radical scavenging activity relationship of flavonoids. Croatia Chemical Acta. 2003; 76: 55–61.
Berg JM, Tymoczko JL, Stryer L. Glycolysis and gluconeogenesis. In: Biochemistry. New York, W. H. Freeman and Company, 2001, pp 425-64.
Caliskan OA, Anil H, Stephenson GR. Cephalaria saponin A, a new bidesmosidic triterpene saponin from Cephalaria transsylvanica. J Nat Prod. 1994; 57: 1001-03.
Dastmalchi K, Dorman HJD, Kosar M, Hiltunen R. Chemical composition and in vitro antioxidant evaluation of a water soluble Moldavian balm (Dracocephalum moldavica L.) extract. Lebensmittel Wissenschaft Technol. 2007; 40: 239–48.
Dorman HJD, Hiltunen R. Fe(II) reductive and free radical scavenging properties of summer savory (Satureja hortensis L.) extract and subfractions. Food Chem. 2004; 88: 193-99.
Eloff JN. A sensitive and quick microplate method to determine the minimal inhibitory concentration of plant extracts for bacteria. Planta Medica. 1998; 64: 711-13.
Gerkens PC, Dobson R, Tabatadze N, Mshviladzade V, Elias R, Peulen OJ, Jolois OM, De Pauw-Gillet MA. Apoptosis and cytolysis induced by giganteosides and hederacolchisides in HL-60 Cells. Anticancer Res. 2007; 27: 2529-34.
Geyid A, Abebe D, Debella A, Makonnen Z, Aberra F, Teka F, Kebede T, Urga K, Yersaw K, Biza T, Mariam BH, Guta M. Screening of some medicinal plants of Ethiopia for their antimicrobial properties and chemical profiles. J Ethnopharmacol. 2005; 97: 421-27.
Gyamfi MA, Yonamine M, Aniya Y. Free-radical scavenging action of medicinal herbs from Ghana: Thonningia sanguinea on experimentally-induced liver injuries. Gen Pharmacol. 1999; 32: 661–67.
Halvorsen BL, Holte K, Myhrstad MCW, Barikmo I, Hvattum E, Remberg SF. Systematic screening of total antioxidants in dietary plants. J Nutr. 2002; 132: 461–71.
Isaac AB, George IN, Oladimeji TA, James DH. A bioactive flavonoid from Pavetta crassipes K. Schum. Org Med Chem Lett. 2011; 1: 14
Kayce P, Kırmızıgül S. Chemical constituents of two endemic Cephalaria species. Records Nat Prod. 2010; 4: 141-48.
Kazeem MI, Abimbola SG, Ashafa AOT. Inhibitory potential of Gossypium arboreum Linn leaf extracts on diabetes key enzymes (α-amylase and α-glucosidase). Bangladesh J Pharmacol. 2013; 8: 149-55.
Kim YM, Jeong YK, Wang MH, Lee WY, Rhee HI. Inhibitory effects of pine bark extract on alpha-glucosidase activity and postprandial hyperglycemia. Nutrition 2005; 21: 756-61.
Kwon YI, Apostolidis E, Kim YC, Shetty K. Health benefits of traditional corn, beans and pumpkin: In vitro studies for hyperglycemia and hypertension management. J Med Foods. 2007; 10: 266–75.
Mabona U, van Vuuren SF. Southern African medicinal plants used to treat skin diseases. South African J Bot. 2013; 87: 175-93.
Mccue PP, Shetty K. Inhibitory effects of rosmarinic acid extracts on porcine pancreatic mylase in vitro. Asia Pacific J Clin Nutr. 2004; 13: 101–06.
Meda A, Lamien CE, Romito M, Millogo J, Nacoulma OG. Determination of the total phenolic, flavonoid and proline contents in Burkina Fasan honey as well as their radical scavenging activity. Food Chem. 2005; 91: 571–77.
Movsumov IS, Garaev EA, Isaev MI. Flavonoids from Cephalaria gigantea flowers. Chem Nat Comp. 2006; 42: 677-80.
Mshvildadze V, Elias R, Faure R, Debrauwer L, Dekanosidze G, Kemertelidze E, Balansard G. Triterpenoid saponins from berries of Hedera colchica. Chem Pharmaceut Bull (Tokyo). 2001; 49: 752-54.
Oboh G, Puntel RL, Rocha JBT. Hot pepper (Capsicum annuum, Tepin and Capsicum chinese, Habanero) prevents Fe2+-induced lipid peroxidation in brain in vitro. Food Chem. 2007; 102: 178-85.
Oyaizu M. Studies on products of browning reaction: Antioxidative activity of products of browning reaction prepared from glucosamine. Japan J Nutr. 1986; 44: 307–15.
Rice-Evans C, Miller NJ, Paganga G. Structure–antioxidant activity relationships of flavonoids and phenolic acids. Free Radical Biol Med. 1996; 20: 933-56.
Rosenbloom R, Chaudhary J, Castro-Eschenbach D. Traditional botanical medicine: An introduction. Am J Ther. 2011; 18: 158-61.
Said O, Khalil K, Fulder S, Azaizeh H. Ethnobotanical survey of medicinal herbs of the Middle Eastern region. J Ethnopharmacol. 2002; 83: 251-65.
Shai LJ, Masoko P, Mokgptho MP, Magano SR, Mogale AM, Boaduo N, Elof JN. Yeast alpha glucosidase inhibitory and antioxidant activity of six medicinal plants collected in Phalaborwa, South Africa. South African J Bot. 2010; 76: 465-70.
Shim YA, Doo HB, Ahn SB, Kim YC. Inhibitory effect of aqueous extract from the gall of Rhus chinensis on alpha-glucosidase activity and postprandial blood glucose. J Ethnopharmacol. 2003; 85: 283–87.
Singleton VL, Orthofer R, Lamuela-Raventos RM. Analysis of total phenols and other oxidation substrates and antioxidants by means of Folin–Ciocalteu reagent. In: Methods in enzymology. Vol. 299. Oxidants and antioxidants, part A San Diego, Academic Press, 1999, pp 152–78.
Tabatadze N, Elias R, Faure R, Gerkens P, De Pauw-Gillet MA, Kemertelidze E, Chea A, Ollivier E. Cytotoxic triterpenoid saponins from the roots of Cephalaria gigantea. Chem Pharmaceut Bull. 2007; 55: 102-05.
Tung YT, Wu JH, Huang CY, Ku YH, Chang ST. Antioxidant activities and phytochemical characteristics of extracts from Acacia confusa bark. Bioresources Technol. 2009; 100: 509-14.
Weichselbaum E, Buttriss JL. Polyphenols in the diet. Nutr Bull. 2010; 35: 157-64.
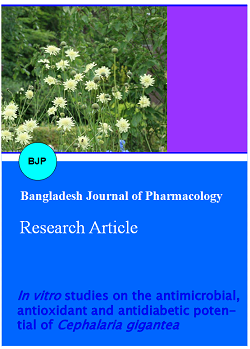