Chemical synthesis, docking studies and biological effects of functionalized 1,3-diaryl-2-propen-1-ones on human colon cancer cells
Abstract
A series of 1, 3-diaryl-2-propen-1-ones was synthesised in order to obtain a new type of anti-cancer drug, designed with hybrid features to inhibit colon cancer activated receptor. Based on computational modelling and docking studies, potential inhibitors were synthesised and their biological activity evaluated. The structures of newly synthesized compounds were confirmed by 1HNMR, 13CNMR and Mass spectrometry. All analogues were evaluated for in vitro cytotoxicity against human colon (caco-2) cancer cell lines. Compounds 1b, 1f-1h, and 2i showed significant cytotoxicity. Chalcones 1b, 1f and 1g were identified as the most potent and selective anti-cancer agents with IC50 values <1 µg/mL and 1.5 µg/mL, against caco-2 cell line, respectively. In conclusion, this finding confirms the suitability of indolyl chalcone analogues as candidates for further investigation towards the management of colon cancer related diseases.
Introduction
Various research groups have focused on chemoprevention and working on development of new lead molecules. Usually early detection and focus on improvement of currently used drugs can be very helpful for curbing the disease. From the available literature it's clear that the quinoline ring system and their fused derivatives are significant structural units and present as substructure in various alkaloids, therapeutics and synthetic analogues, which exhibit good biological activities (Larsen et al., 1996; Roma et al., 2000). Various quinolines derivatives are reported as anti-malarial, anti-inflammatory, antiasthmatic, antibacterial, anti-hypertensive and platelet derived growth factor receptor tyrosine kinase (PDGF-RTK) inhibiting agents (Dube et al., 1998). A large variety of quinolines are reported to exhibit substantial anti-cancer activities (Alkasomi et al., 2010) Quinoline derivative act as anti-cancer agents through a variety of mechanisms for example; cell cycle arrest in the G2 phase (Kim et al., 2005) inhibition of topoisomerase (Ching et al., 2008) and tubulin polymerization inhibition (Alkasomi., 2009) Another mechanism of action is the inhibition of tyrosine kinases (Mulvihill et al., 2008). These results encourage us to design the molecules containing quinoline ring with different functional group to assess their biological activity.
Medicinal chemists are tirelessly exploring for a better and more suitable cancer therapeutics. Chalcones (1, 3-diaryl-2-propen-1-ones), constituting an enone system between two aromatic rings are an important class of natural products which are considered as precursors for various flavonoids and exhibit interesting pharmacological activities (Stu et al., 1971). Chalcones, originating from natural and synthetic routes possess several biological activities, such as cytotoxic (Modzelewska et al., 2006) antimalarial (Dominguez et al., 2005), anti-leishmanial (Boeck et al., 2006) anti-inflammatory (Yang et al., 2007), anti-HIV (Cheenpracha et al., 2006), antifungal (Svetaz et al., 2004) and as tyrosine kinase inhibitors (Neryr et al., 2004). Because of very high pharmacological interest, these molecules have attracted medicinal chemists to design and synthesize further large number of chalcones with different functional groups. In the recent years, the development of anti-cancer agents was achieved by structural modification of chalcones to increase their bioavailability and to study the effect of various substituents on aryl or heteroaryl rings (Meng et al., 2007).
The heteroaryl rings are widely distributed in nature and possess a variety of significant biological activities. The indole ring is an important moiety in many pharmacologically active compounds in which some studies related reported for anti-cancer effectiveness (Grugni et al., 2006). Some of the individual anti-cancer compounds in which the indole ring is responsible for the activity are panobinostat (Prince et al., 2009), cediranib (Nikolinakos et al., 2008), indole-3-carbinol (Aggarwal et al., 2005) (Figure 1). Basically, indolyl chalcones are not much explored for their anti-cancer potential (Aggarwal et al., 2005). In the present study, we have synthesized two different series Figure 2 of novel indolyl chalcones 1a-j (Scheme 1) and 2a-k (Scheme 2) and evaluated their anti-cancer activity in vitro against four human cancer cell lines. Compounds 1b, 1f-1h, and 2i showed significant cytotoxicity. Chalcones 1b, 1f and 1g were identified as the most potent and selective anti-cancer agents with IC50 values 7.4 µg/mL and 7.8 µg/mL, against human colon (Caco-2) cell line, respectively. Based on computational modelling and docking studies, potential inhibitors were synthesised and their biological activity evaluated.
Scheme 1: Reagents and conditions: A) allyl bromide, acetone, 60°C; B) NaOH, methanol, 1-15 hours, RT
Scheme 2: Reagents and conditions: c) SOCl2, methanol, 1-2 hours, RT
Figure 1: Anti-cancer drugs having indole ring
Figure 2: Skeleton of chalcone (alpha, beta-unsaturated ketone) responsible for its biological activity
Materials and Methods
Chemistry and instrument
Melting point was determined on a Toshniwal melting point apparatus and is uncorrected. IR spectra were recorded on a PerkinElmer 1719 FT-IR spectrophotometer. NMR spectra were obtained in acetone-d6, DMSO-d6 and pyridine-d5 on a Bruker Avance, 300 MHz instrument using TMS as internal standard. The chemical shift values are reported in ppm and coupling constants in Hz. ESI-MS spectra were recorded on a Perkin Elmer Turbo Mass/Shimadzu LC-MS. TLC analyses were carried out on precoated silicage l60 F254 plates (Merck) using solvent system, hexane: ethyl acetate (7:3). The compounds were visualized by either exposure of TLC plates to I2 vapours or by spraying with vanillin- sulphuric acid reagent, followed by heating at 110°C for 15 min. Si-gel, 60-120 mesh (spectrochem) was used in the column chromatography for the purification of metabolites. HPLC analyses were carried out on waters spherisorb ODS2 (250 x 4.6 mm i.d., 10 µm) column using binary gradient elution with acetonitrile and water mobile phase (70:30) at a flow rate of 0.6 mL /min, column temperature of 25º and UV detection at λ230 nm. The compounds were identified by their spectral IR, ID (1H, 13C, DEPT) and 2D (COSY, HSQC, HMBC) ESIMS) NMR and ESI/MS analysis.
Synthesis of indolyl chalcones, series 1
Indolyl chalcones 1a-j were prepared by the reaction of indol-3-carboxaldehyde 5 with appropriate acetophenone 4 in presence of NaOH at RT (Scheme 1) (Jeong et al., 2004).
The contents of reaction mixture were poured into ice-cold water and neutralized with dilute hydrochloric acid. The solid so obtained was filtered, column chromatographed and recrystallized from ethanol to afford pure compounds.
Trans-3-(1H-indol-3-yl)-1-(4’-flouro-3’-methylphenyl)-2-propen-1-one (1a)
Orange powder; 20% yield; mp 59-60°C; IR νmax(KBr): 3422, 1548, 1154, 737 (NH), 1653 (chalcone C=O), 1520, 1491, 1440(aromatics) cm-1;1H NMR (300 MHz, acetone-d6): δ 2.35 (3H, s, CH3), 7.19 (1H, d, J=9.3 Hz, H-5’’), 7.27 (2H, m, H-5’, H-6’), 7.54 (1H, dd, J=8.1, 1.2 Hz, H-4’), 7.70 (1H, d, J=15.6 Hz, H-2), 7.98 (1H, d, J=2.7 Hz, H-2’’), 8.04 (1H, dd, J=8.7, 2.7 Hz, H-6’’), 8.08 (1H, brs, H-2’), 8.11 (1H, d, J=15.6 Hz, H-3), 8.10 (1H, d, J=8.1, 1.2 Hz, H-7’), 10.90 (1H, brs, NH); 13C NMR(75 MHz, acetone-d6): δ 14.35 (CH3), 113.01 (C-4’), 114.36 (C-1’), 115.49a (C-5’’), 115.79a (C-2), 121.16 (C-7’), 121.93 (C-6’), 123.61 (C-5’), 126.34 (C-3’), 128.99 (C-6’’), 132.66b (C-2’’), 132.72b (C-2’), 136.17 (C-1’’), 138.65 (C-8’), 139.36 (C-3), 162.74c (C-3’’), 166.03c (C-4’’), 188.37 (C-1) (a,b,c=interchangable); ESI-MS, MeOH (Positive): m/z 280 [M+H]+, 302 [M+Na]+, (Negative): 278[M-H]-, C18H14FNO.
Trans-3-(1H-indol-3-yl)-1-(4’-benzyloxyphenyl)-2-propen-1-one (1b)
Yellow solid; 60%, yield; mp 74-75°C; IR νmax (KBr): 3448, 1562, 1120, 735 (NH), 1654(chalcone C=O), 1523, 1495, 1437 (aromatics) cm-1;1H NMR (300 MHz, acetone-d6): δ 5.29 (2H, s, H2-7’’), 7.24 (2H, m, H-5’, H-6’), 7.27 (1H, m, H-4’’), 7.35 (3H, m, H-10’’, H-11’’, H-12’’), 7.49 (2H, m, H-4’, H-5’’), 7.53(2H, m H-9’’, H-13’’), 7.68 (1H, d, J=15.6 Hz, H-2), 7.70 (1H, s, H-2’’), 7.72 (1H, d, J=7.8, 2.1 Hz, H-7’), 8.01 (1H, d, J=2.7 Hz, H-2’), 8.08 (1H, m, H-6’’), 8.10 (1H, d, J=15.6 Hz, H-3), 10.92 (1H, brs, NH); 13C NMR (75 MHz, acetone-d6): δ 70.16 (C-7’’), 112.70 (C-4’), 114.05 (C-1’), 114.28 (C-2’’), 116.85 (C-2), 119.35 (C-4’’), 120.82 (C-6’’), 121.18 (C-7’), 121.65 (C-6’), 123.29 (C-5’), 126.04 (C-3’), 128.00 (C-9’’, (C-13’’), 128.22 (C-5’’), 128.85 (C-10’’, C-12’’), 130.04 (C-11’’), 132.38 (C-2’), 137.74 (C-8’), 138.33 (C-1’’), 139.62 (C-3), 141.10 (C-8’’), 159.36 (C-3’’), 189.09 (C-1); ESI-MS, MeOH (Positive): m/z 354 [M+H]+, (Negative): 352[M-H]-, C24H19NO2.
Trans-3-(1H-indol-3-yl)-1-(anthracenyl)-2-propen-1-one (1c)
Yellow powder; 80% yield; mp 108-109°C; IR νmax(KBr): 3395, 1561, 1164, 746 (NH), 1649 (chalcone C=O), 1515, 1483, 1430 (aromatics) cm-1;1H NMR (300 MHz, acetone-d6): δ 7.16 (2H, d, J=7.8 Hz, H-4’’, H-12’’), 7.23 (2H, m, H-5’, H-6’), 7.26 (4H, m, H-5’’, H-6’’, H-10’’, H-11’’), 7.44 (1H, d, J=2.1 Hz, H-8’’), 7.51 (1H, dd, J=8.1, 2.1 Hz, H-4’), 7.59 (2H, d, J=7.8 Hz, H-3’’, H-13’’), 7.87 (1H, d, J=15.6 Hz, H-2), 7.89 (1H, brs, H-2’), 8.21 (1H, dd, J=8.1, 2.1 Hz, H-7’), 8.55 (1H, d, J=15.6 Hz, H-3), 13.05 (1H, brs, NH); 13C NMR (75 MHz, acetone-d6): δ 111.65 (C-5’’, C-11’’), 112.53 (C-4’’, C-12’’), 113.06 (C-4’), 114.23 (C-1’), 116.45 (C-3’’, C-13’’), 121.11a (C-7’), 121.89a (C-2, C-6’), 123.15b (C-6’’, C-10’’), 123.48b (C-5’), 124.15 (C-8’’), 126.40 (C-3’), 128.15 (C-1’’), 133.50 (C-2’), 138.88 (C-8’), 139.30 (C-3), 155.35c (C-7’’, C-9’’), 155.91c (C-2’’, C-14’’), 179.72 (C-1) (a,b,c=interchangable); ESI-MS, MeOH (Positive): m/z 348 [M+H]+, 346[M-H]-, C25H17NO.
Trans-3-(1H-indol-3-yl)-1-( benzofuran)-2-propen-1-one (1d)
Dark brown solid; 40%, yield; mp 59-60°C; IR νmax(KBr): 3395, 1151, 1156, 736 (NH), 1654 (chalcone C=O), 1509, 1483, 1427 (aromatics) cm-1;1H NMR (300 MHz, acetone-d6): δ 7.11 (2H, m, H-5’, H-6’), 7.35 (1H, d, J=15.6 Hz, H-2), 7.49 (3H, m, H-4’, H-5’’, H-6’’), 7.53 (1H, d, J=15.6 Hz, H-3), 7.61 (1H, d, J=2.7 Hz, H-2’), 7.92 (1H, dd, J=8.4, 2.1 Hz, H-7’), 8.01 (1H, dd, J=6.9, 2.7 Hz, H-4’’), 8.12 (1H, dd, J=7.2, 2.4 Hz, H-8’’), 8.62 (1H, s, H-2’’), 13.05 (1H, brs, NH); 13C NMR (75 MHz, acetone-d6): δ 112.92 (C-4’), 113.31 (C-1’), 120.69a (C-2), 121.86a (C-7’), 123.42 (C-6’), 124.71 (C-5’), 125.71b (C-3’, C-2’’), 125.91b (C-7’’), 126.66 (C-4’’), 127.96 (C-5’’), 128.72 (C-3’’), 129.05 (C-6’’), 131.76 (C-8’’) 133.17 (C-2’), 136.65c (C-8’), 138.47c (C-1’’), 142.74 (C-3), 198.91 (C-1), (a,b,c=interchangable); ESI-MS, MeOH (Positive): m/z 288[M+H]+, Negative: 286[M-H]-, C19H13NO2.
Trans-3-(1H-indol-3-yl)-1-(4’-chlorophenyl)-2-propen-1-one (1e)
Yellow fluffy crystals, 60% yield, obtained and analysed by spectroscopic data as described by an earlier method (Black., et al 1992).
Trans-3-(1H-indol-3-yl)-1-(2’-chlorophenyl)-2-propen-1-one (1f)
Yellow shiny crystals, 85% yield, obtained and analysed by spectroscopic data as described by an earlier method (Black., et al 1992).
Trans-3-(1H-indol-3-yl)-1-(2’-hydroxyphenyl)-2-propen-1-one (1g)
Light brown crystals, 55% yield, obtained and analysed by spectroscopic data as described by an earlier method (Black., et al 1992).
Trans-3-(1H-indol-3-yl)-1-(3’-allyloxyphenyl)-2-propen-1-one (1h)
Light brown powder; 65% yield; mp 90-92°C;IR νmax(KBr): 3389 1564, 1211, 739 (NH), 1653 (chalcone C=O), 1523, 1458, 1420 (aromatics) cm-1;1H NMR (300 MHz, DMSO-d6): δ 4.64 (2H, d, J=5.1 Hz, H2-7’’), 5.26 (1H, dd, J=10.5, 0.9 Hz, Ha-9’’), 5.40 (1H, dd, J=17.4, 1.5 Hz, Hb-9’’), 6.05 (1H, m, H-8’’), 7.24 (3H, m, H-5’, H-6’, H-4’’), 7.49 (2H, d, J=8.1 Hz, H-4’, H-5’’), 7.54 (1H, s, H-2’’), 7.60 (1H, d, J=15.6 Hz, H-2), 8.03 (1H, d, J=6.6 Hz, H-7’), 8.06 (1H, d, J=15.6 Hz, H-3), 8.09 (1H, d, J=2.1 Hz, H-2’), 7.70 (1H, d, J=8.1 Hz, H-6’’), 9.90 (1H, brs, NH); 13C NMR (75 MHz, DMSO-d6): δ 69.20 (C-7’’), 113.42 (C-4’), 113.60 (C-1’), 114.44 (C-2’’), 116.31 (C-2), 118.42 (C-9’’), 119.82 (C-4’’), 121.09 (C-7’), 121.59 (C-6’’), 122.07 (C-6’), 123.59 (C-5’), 126.05 (C-3’), 130.72 (C-5’’), 134.10 (C-2’), 134.39 (C-8’’), 138.44 (C-8’), 140.08 (C-3), 140.90 (C-1’’), 159.25 (C-3’’), 189.60 (C-1); ESI-MS, MeOH (Positive): m/z 304[M+H]+, Negative: 302[M-H]-, C20H17NO2.
Trans-3-(1H-indol-3-yl)-1-(4’-allyloxyphenyl)-2-propen-1-one (1i)
Light brown powder; 30%; mp 110-111°C; IR νmax(KBr): 3372 1561, 1205, 736 (NH), 1651 (chalcone C=O), 1511, 1470, 1406 (aromatics) cm-1;1H NMR (300 MHz, DMSO-d6): δ 4.65 (2H, d, J=5.04 Hz, H2-7’’), 5.28 (1H, d, J=10.52 Hz, Hb-9’’), 5.42 (1H, d, J=17.21 Hz, Ha-9’’), 6.05 (1H, m, H-8’’), 7.08 (2H, d, J=8.64 Hz, H-3’’, H-5’’), 7.24 (1H, m, H-5’, H-6’), 7.53 (1H, m, H-4’), 7.67 (1H, d, J=15.40 Hz, H-2), 8.06 (1H d, J=15.56 Hz, H-3), 8.09 (2H, m, H-2’, H-7’), 8.13 (1H, d, J=8.72 Hz, H-2’’), 8.13 (1H, d, J=8.72 Hz, H-6’’), 9.91 (1H, brs, NH); 13C NMR (75 MHz, DMSO-d6): δ 68.86 (C-7’’), 113.00 (C-4’), 113.19 (C-1’), 115.02 (C-3’’, C-5’’), 115.66 (C-2), 118.36 (C-9’’), 120.74 (C-7’), 121.53 (C-6’), 123.07 (C-5’), 125.66 (C-3’), 130.85 (C-6’’), 131.80 (C-1’’), 130.85 (C-2’’), 133.37 (C-2’), 133.65 (C-8’’), 138.06 (C-8’), 138.74 (C-3), 162.05 (C-4’’), 187.76 (C-1); ESI-MS, MeOH (Positive): m/z 304[M+H]+, Negative: 302[M-H]-, C20H17NO2.
Trans-3-(1H-indol-3-yl)-1-(4’-allyloxy-2’-hydroxyphenyl)-2-propen-1-one (1j)
Yellow powder; 30% yield; mp 110-111°C; IR νmax(KBr): 3569 1559, 1229, 735 (NH), 3380 (OH), 1621 (chalcone C=O), 1497, 1439, 1369 (aromatics) cm-1; 1H NMR (300 MHz, DMSO-d6): δ 4.65 (2H, d, J=4.92 Hz, H2-7’’), 5.29 (1H, d, J=10.68 Hz, Hb-9’’), 5.42 (1H, d, J=17.32 Hz, Ha-9’’), 6.04 (1H, m, H-8’’), ), 6.50 (1H, s, H-3’’), 6.58 (1H, dd, J=8.92, 2.08 Hz, H-5’’), 7.25 (2H, m, H-5’, H-6’), 7.52 (dd, J=7.92, 2.52 Hz, H-4’), 7.54 (1H, dd, J=7.92, 2.52 Hz, H-4’), 7.68 (1H, d, J=15.3 Hz, H-2), 8.11 (1H, dd, J=7.92, 2.52 Hz, H-7’), 8.14 (1H, s, H-2’), 8.17 (1H, d, J=15.3 Hz, H-3), 8.19 (1H, m, H-6’’), 9.45 (1H, brs, NH); 13C NMR (75 MHz,DMSO-d6): δ 69.00 (C-7’’), 113.10 (C-4’), 113.36 (C-1’), 102.20 (C-3’’), 107.93 (C-5’’), 114.11 (C-2), 114.51 (C-1’’), 118.46 (C-9’’), 120.85 (C-7’), 121.81 (C-6’), 123.30 (C-5’), 125.63 (C-3’), 132.46 (C-6’’), 165.89 (C-2’’), 134.43 (C-2’), 133.47 (C-8’’), 138.14 (C-8’), 139.90 (C-3), 164.43 (C-4’’), 192.08 (C-1); ESI-MS, MeOH (Positive): m/z 320[M+H]+, Negative: 318[M-H]-, C20H17NO3.
Synthesis of indolyl chalcones 2(a-k)
Further, the reaction of 3-acetylindole 6 with appropriate aldehyde 7 in presence of SOCl2 resulted in the formation of indolyl chalcones 2a-k (Scheme 2) (Prince et al., 2009). Acetophenones (4h-4j) for the preparation of the compounds 1h-1j were prepared by etherification of o-hydroxy (3h), p- hydroxy (3i) and o,p-hydroxy (3j) acetophenones respectively with allyl bromide in the presence of KBr in acetone using refluxing condition.
The contents of reaction mixture were poured into ice-cold water. The solid so obtained was filtered, dried and recrystallized from ethanol to afford pure 2(a-k).
Trans-1-indolyl-3-(anthracenyl)-2-propen-1-one (2a)
Yellow powder; 70% yield; mp 190-191°C, IR νmax(KBr): 3398, 1570, 1234, 728 (NH), 1639 (chalcone C=O), 1518, 1442, 1419, 1381 (aromatics) cm-1; 1H NMR (300 MHz, pyridine-d5): δ 6.90 (4H, m, H-5’, H-5’’, H-6’’, H-11’), 6.83(2H, m, H-4’, H-12’), 6.95 (2H, m, H-3’, H-13’), 7.16 (1H, d, J=15.6 Hz, H-2), 7.53 (1H, d, J=7.8 Hz, H-4’’), 7.83 (2H, d, J=8.1 Hz, H-6’, H-10’), 7.96 (1H, brs, H-8’), 8.07 (1H, brs, H-2’’), 8.45 (1H, d, J=15.56 Hz, H-3), 8.63 (1H, d, J=7.8 Hz, H-7’’), 12.81 (1H, brs, NH);13C NMR (75 MHz, pyridine-d5): δ 113.08 (C-4’’), 119.2 (C-1’’), 123.02 (C-6’’, C-7’’), 123.32 (C-5’’), 124.23 (C-4’, C-12’), 126.04 (C-5’, C-11’), 126.10 (C-6’, C-10’), 126.81 (C-3’, C-13’), 127.49 (C-3’’), 128.34 (C-8’), 130.19 (C-1’), 131.67 (C-2’, C-14’), 132.01 (C-7’, C-9’), 134.39 (C-2), 137.57 (C-3), 135.04 (C-2’’), 138.47 (C-8’’), 184.37 (C-1); ESI-MS, MeOH (Positive): m/z 348 [M+H]+, 370 [M+Na]+, C25H17NO.
Trans-1-indolyl-3-(2',4’-dimethoxyphenyl)-2-propen-1-one (2b)
Creamish white crystals, 10% yield, obtained and analysed by spectroscopic data as described by an earlier method (Yesuthangan., et al 2011).
Trans-1-indolyl-3-(3',4’,5’-trimethoxyphenyl)-2-propen-1-one (2c)
Light orange crystals;70% yield; mp 191-192°C; IR νmax(KBr): 3448 1581, 1197, 755 (NH), 1642 (chalcone C=O), 1515, 1459, 1426 (aromatics) cm-1;1H NMR (300 MHz, DMSO-d6): δ 3.86 (3H, s, OCH3), 3.71 (6H, s, 2 x OCH3), 7.18 (2H, brs, H-2’, H-6’), 7.24 (2H, m, H-5’’, H-6’’), 7.51 (1H, dd, J=6.3, 2.1 Hz, H-4’’), 7.62 (1H, d, J=15.6 Hz, H-2), 7.78 (1H, d, J=15.6 Hz, H-3), 8.38 (1H, dd, J=6.3, 2.1 Hz, H-7’’), 8.75 (1H, d, J=3 Hz, H-2’’), 12.12 (1H, brs, NH);13C NMR (75 MHz, DMSO-d6): δ 56.97 (2 x OCH3), 60.99 (OCH3), 106.92 (C-2’, C-6’), 113.04 (C-4’’), 118.68 (C-1’’), 122.70 (C-6’’, C-7’’), 123.97 (C-5’’), 124.77 (C-3), 126.81 (C-3’’), 131.69 (C-1’), 135.52 (C-2’’), 137.79 (C-8’’), 140.07 (C-4’), 140.84 (C-2), 154.01 (C-3’, C-5’), 184.54 (C-1); ESI-MS, MeOH (Positive): m/z 338 [M+H]+, C20H19NO4.
Trans-1-indolyl-3-(2'3',4’-trimethoxyphenyl)-2-propen-1-one(2d)
Creamish powder;70% yield; mp 163-164°C; IR νmax(KBr): 3431 1586, 1201, 754 (NH), 1640 (chalcone C=O), 1525, 1493, 1414 (aromatics) cm-1;1H NMR (300 MHz, DMSO-d6): δ 3.67 (3H, s, OCH3), 3.76 (6H, s, 2 x OCH3), 6.71 (1H, d, J=9.0 Hz, H-5’), 7.12 (2H, m, H-5’’, H-6’’), 7.40 (1H, d, J=6.6 Hz, H-4’’), 7.60 (1H, d, J=15.6 Hz, H-2), 7.66 (1H, d, J=9.0 Hz, H-6’), 7.74 (1H, d, J=15.56 Hz, H-3), 8.25 (1H, d, J=6.6 Hz, H-7’’), 8.57 (1H, brs, H-2’’), 11.79 (1H, brs, NH);13C NMR (75 MHz, DMSO-d6): δ 56.87, 61.32, 62.3 (3 x OCH3), 109.28 (C-5’), 113.02 (C-4’’), 118.64 (C-1’’), 122.63 (C-6’’, C-7’’), 122.45 (C-1’), 123.41 (C-6’), 123.91 (C-5’’), 124.01 (C-2), 126.80 (C-3’’), 134.66 (C-3), 135.18 (C-2’’), 137.71 (C-8’’), 142.72 (C-3’), 153.55 (C-2’), 155.85 (C-4’), 184.70 (C-1); ESI-MS, MeOH (Positive): m/z 338 [M+H]+, Negative: 336[M-H]+, C20H19NO4.
Trans-1-indolyl-3-(3'-ethoxy-4-hydroxyphenyl)-2-propen-1-one (2e)
Light brown crystals;70% yield; mp 154-155°C; IR νmax(KBr): 3535 1557, 1204, 746 (NH), 3394 (OH), 1641 (chalcone C=O), 1512, 1479, 1403 (aromatics) cm-1;1H NMR (300 MHz, DMSO-d6): δ 1.17 (3H, t, J=6.9 Hz, CH3), 3.99 (2H, q, J=6.9 Hz, OCH2-), 7.35 (1H, d, J=8.1 Hz, H-5’), 7.39 (1H, d, J=8.1 Hz, H-6’), 7.47 (2H, m, H-5’’, H-6’’), 7.55 (1H, d, J=1.5 Hz, H-2’), 7.67 (1H, d, J=7.5 Hz, H-4’’), 7.85 (1H, d, J=3.0 Hz, H-2’’), 8.06 (1H, d, J=15.3 Hz, H-2), 8.35 (1H, d, J=15.3 Hz, H-3), 9.21 (1H, d, J=7.8 Hz, H-7’’), 13.23 (1H, brs, NH);13C NMR (75 MHz, DMSO-d6): δ 16.07 (CH3), 65.89 (OCH2-), 113.90 (C-4’’), 114.33 (C-2’, C-14’), 118.24 (C-5’, C-11’), 120.84 (C-1’’), 123.47 (C-2), 123.76 (C-6’’), 124.59 (C-7’’), 124.77 (C-5’’), 125.02 (C-6’, C-10’), 128.78 (C-3’’), 129.10 (C-1’), 135.34 (C-2’’), 139.48 (C-8’’), 142.96 (C-3), 149.57 (C-3’, C-13’), 152.04 (C-4’, C-12), 186.47 (C-1); ESI-MS, MeOH (Positive): m/z 308 [M+H]+, Negative: 306[M-H]+, C19H17NO3.
Trans-1-indolyl-3-(3',5’-dimethoxy-4’-hydroxyphenyl)-2-propen-1-one (2f)
Creamy crystals;70% yield; mp 210-211°C; IR νmax(KBr): 3445, 1580, 1191, 740 (NH), 3445 (OH), 1640 (chalcone C=O), 1522, 1491, 1404 (aromatics) cm-1;1H NMR (300 MHz, DMSO-d6): δ 1H NMR (300 MHz, DMSO-d6): δ 3.51 (6H, brs, 2x OCH3), 6.49 (2H, br s, H-2’), 6.61 (2H, m, H-5’’, H-6’’), 6.90 (1H, dd, J=6.9, 1.5 Hz, H-4’’), 6.99 (1H, d, J=15.3Hz, H-2), 7.04 (1H, d, J=15.3 Hz, H-3), 7.70 (1H, dd, J=8.1, 1.8 Hz, H-7’’), 8.06 (1H, d, J=2.7 Hz, H-2’’), 11.43 (1H, brs, NH); 13C NMR (75 MHz, DMSO-d6): δ 57.01 (2 x OCH3), 107.03 (C-2’, C-6’), 113.08 (C-4’’), 118.56 (C-1’’), 122.47 (C-2), 122.61 (C-7’’), 122.81 (C-6’’), 124.08 (C-5’’), 126.42 (C-1’), 126.64 (C-3’’), 135.29 (C-2’’),137.67 (C-8’’), 138.62 (C-4’), 141.83 (C-3), 148.90 (C-3’, C-5’), 185.13 (C-1); ESI-MS (Positive): m/z 324 [M+H]+, Negative: 322 [M-H]-, C19H17NO4.
Trans-1-indolyl-3-(3',5’-dimethoxy-4’-benzyloxyphenyl)-2-propen-1-one (2g)
Creamish crystals;70% yield; mp 209-210°C; IR νmax(KBr): 3440 1576, 1203, 739 (NH), 1655 (chalcone C=O), 1742(ester CO), 1512, 1466, 1426 (aromatics) cm-1;1H NMR (300 MHz, DMSO-d6): δ 7.24 (1H, dd, J=7.5, 1.5 Hz, H-10’), 7.31 (2H, d, J=7.5 Hz, H-3’, H-6’), 7.54 (2H, dd, J=8.4, 2.7 Hz, H-9’, H-11’), 7.58 (2H, m, H-5’’, H-6’’), 7.68 (1H, d, J=15.3 Hz, H-2), 8.70 (1H, br s, H-2’’), 7.72 (1H, dd, J=7.2, 1.2 Hz, H-4’’), 7.88 (1H, d, J=15.3 Hz, H-3), 8.12 (2H, d, J=7.5 Hz, H-8’, H-12’), 8.38 (1H, dd, J=6.6, 2.1 Hz, H-7’’), 12.20 (1H, brs, NH);13C NMR (75 MHz, DMSO-d6): δ 106.79 (C-2’, C-6’), 113.12 (C-4’’), 118.67 (C-1’’), 122.77 (C-6’’, C-7’’), 123.23 (C-5’’), 125.90 (C-2), 126.79 (C-3’’), 129.32 (C-1’), 129.90 (C-9’, C-11’), 130.85 (C-8’, C-12’), 134.96 (C-10’), 135.77 (C-2’’), 137.83 (C-8’’), 140.43 (C-3), 153.02 (C-3’, C-4’, C-5’), 164.48 (CO), 184.42 (C-1); ESI-MS, MeOH (Positive): m/z 428[M-H]+, 450 [M+Na]+, Negative: 428[M-H]-, C26H21NO5.
Trans-1-indolyl-3-(4'-hydroxyphenyl)-2-propen-1-one (2h)
Dark brown powder,85% yield, obtained and analysed by spectroscopic data as described by an earlier method (Kumar., et al 2010).
Trans-1-indolyl-3-(2'-methylphenyl)-2-propen-1-one (2i)
Obtained as brown solid; 80% yield; mp 140-142ºC; IR νmax(KBr): 3422, 1562, 1156, 748 (NH), 1639 (chalcone C=O), 1520, 1442, 1492 (aromatics) cm-1;1H NMR (300 MHz, DMSO-d6):δ 2.34(3H, s, CH3), 7.13-7.18 (5H, m, H-3', H-4', H-5', H-5'', H-6''), 7.40 (1H, dd, J=8.1 Hz, 2.1 Hz, H-4''), 7.63 (1H, d, J=15.6 Hz, H-2), 7.82 (1H, d, J=15.6 Hz, H-3), 7.87 (1H, dd, J=7.5, 2.4 Hz, H-6'), 8.25 (1H, dd, J=6.6, 2.1Hz, H-7''), 8.63 (1H, d, J=3.0 Hz, 1H, H-2''), 12.05 (1H, brs, NH); 13CNMR (75 MHz, DMSO-d6): δ 20.23 (CH3), 113.07 (C-4''), 118.54 (C-1''), 122.61 (C-7''), 122.74 (C-6''), 123.99 (C-5''), 126.36 (C-2), 126.80 (C-3''), 127.11 (C-5'), 127.36 (C-6' ), 130.41 (C-4'), 131.55 (C-3'), 134.68 (C-1'), 135.63 (C-2''), 137.54 (C-3), 137.76 (C-8''), 138.23 (C-2'), 184.55 (C-1); ESI-MS, MeOH (Positive): m/z 262 [M+H]+, 284 [M+Na]+, Negative: 260 [M-H]-, C18H15NO.
Trans-1-indolyl-3-(thiophenyl)-2-propen-1-one (2j)
Creamish white powder;70% yield; mp 181-182°C; IR νmax(KBr): 3448 1578, 1199, 754 (NH), 1632 (chalcone C=O), 1523, 1493, 1438, 1315 (aromatics) cm-1;1H NMR (300 MHz, DMSO-d6): δ 6.03 (1H, d, J=4.2 Hz, H-2’), 6.10 (2H, m, H-5’’, H-6’’), 6.34 (1H, m, H-4’’), 6.36 (1H, d, J=15.3 Hz, H-2), 6.45 (1H, brs, H-4’), 6.55 (1H, d, J=4.2 Hz, H-3’), 6.66 (1H, d, J=15.3 Hz, H-3), 7.50 (1H, d, J=7.5 Hz, H-7’’), 7.52 (1H, brs, H-2’’), 10.95 (1H, brs, NH);13C NMR (75 MHz, DMSO-d6): δ 113.06 (C-4’’), 118.34 (C-1’’), 122.62 (C-7’’), 122.72 (C-6’’), 124.00 (C-5’’), 124.07 (C-2), 126.71 (C-3’’), 129.32 (C-2’), 129.76 (C-3’), 132.06 (C-4’), 133.32 (C-3), 135.40 (C-2’’), 137.73 (C-8’’), 141.06 (C-1’), 184.03 (C-1); ESI-MS, MeOH (Positive): m/z 254 [M+H]+, 276 [M+Na]+, Negative: 252 [M-H]-, C15H11NOS.
Trans-1-indolyl-3-(benzodioxanyl)-2-propen-1-one (2k)
Light orange,90% yield; mp 154-155°C; IR νmax(KBr): 3449 1580, 1251, 752 (NH), 1638 (chalcone C=O), 1509, 1439, 1291 (aromatics) cm-1;1H NMR (300 MHz, DMSO-d6): δ 4.25 (2H, s, H-5’, H-6’), 6.88 (1H, d, J=8.4 Hz, H-3’), 7.21 (2H, m, H-5’’, H-6’’), 7.28 (1H, dd, J=8.4, 1.5 Hz, H-2’), 7.42 (1H, d, J=1.5 Hz, H-8’), 7.48 (1H, dd, J=6.6, 2.7 Hz, H-4’’), 7.52 (1H, d, J=15.3 Hz, H-2), 7.66 (1H, d, J=15.6 Hz, H-3), 8.33 (1H, dd, J=6.3, 2.4 Hz, H-7’’), 8.68 (1H, brs, H-2’’), 12.04 (1H, brs, NH);13C NMR (75 MHz, DMSO-d6): δ 64.86* (C-4’), 65.21* (C-5’), 122.66 (C-6’’, C-7’’), 123.26 (C-8’), 123.70 (C-2), 123.94 (C-5’’), 126.76 (C-3’’), 129.56 (C-1’), 135.34 (C-2’’), 137.71 (C-8’’), 140.28 (C-3), 144.45 (C-6’), 145.97 (C-3’), 184.69 (C-1) (*=interchangable);ESI-MS, MeOH (Positive): m/z 306 [M+H]+, Negative: 304 [M-H]-, C19H15NO3.
Molecular modelling parameters and energy minimization
To find the possible interactions of indolyl chalcones analogues compounds 1b, 1f and 1g with colon cancer target cyclin-dependent kinase2 (CDK2), we docked compounds at CDK2 binding site. Sybyl X 2.0 interfaced with Surflex–Dock module was used for molecular docking. Program automatically docks ligand into binding pocket of a target protein using protomol based algorithm and empirically produced scoring function. The X-ray crystallographic structures of CDK2 complex with ligand (PDB ID: 2R3J) (Yoon et al., 2013) was taken from the protein data bank and water molecules were removed, H atoms were added and side chains were fixed. Protein structure minimization was performed by applying Tripos force field and partial atomic charges were calculated by Gasteiger-Huckel method. In reasonable binding pocket, all the compounds were docked into the binding pocket and 20 possible active docking conformations with different scores were obtained for each compound. During the docking process, all of the other parameters were assigned their default values (Yadav et al., 2014).
Screening through pharmacokinetic properties
During the process of drug discovery, most of drugs fail to cross the clinical trials because of poor pharmacokinetic properties (absorption, Distribution, metabolism, excretion, and toxicity) (Yadav et al., 2013). Some properties correlate well e.g., primary determinant of fractional absorption referred to as polar surface area (PSA) (cut-off <140 Angstrom2) and low molecular weight for absorption). The compound distribution in the body depends on factors such as blood-brain barrier, permeability, the volume of distribution and plasma protein binding. The descriptors' values of 90% orally active compounds follows Lipinski's rule. The bioavailability of compounds was evaluated by topological polar surface area value. This descriptor correlates well with passive molecular transport through membranes. The number of rotatable bonds is a topological parameter as a measure of molecular flexibility (cut-off ≤10) and oral bioavailability (Bhagat et al., 2013).
MTT antiproliferative activity assay
In vitro anti-cancer activity of phytomolecules is done by using MTT assay. Cytotoxicity testing in vitro was done by the method of Woerdenbag et al. 1-2 x 104 cells/well were incubated in the 5% CO2 incubator for 24 hours to enable them to adhere properly to the 96-well polystyrene microplate (Grenier, Germany). Test compounds dissolved in 100% DMSO (Merck, Germany) in at least ï¬ve doses was added and left for 6 hours after which the compounds plus media was replaced with fresh media and the cells were incubated for another 48 hours in the CO2 incubator at 37°C. The concentration of DMSO used in our experiments never exceeded 1.25%, which was found to be non-toxic to cells. Then, 10 µL MTT [3-(4,5-dimethylthiazol-2-yl)-2,5-diphenyltetrazolium bromide; Sigma M 2128] was added, and plates were incubated at 37°C for 4 hours. One hundred microlitres of dimethyl sulfoxide (DMSO, Merck, Germany) was added to all wells and mixed thoroughly to dissolve the dark blue crystals. After a few min at room temperature to ensure that all crystals were dissolved, the plates were read on a spectrofluorometer FLUO star Omega (BMG Labtech) at 570 nm. Plates were normally read within 1 hour of adding the DMSO. The experiment was done in triplicate and the inhibitory concentration (IC) values were calculated as follows:
% inhibition = (1-OD at 570 nm of sample well/OD at 570 nm of control well) x 100
IC50 is the concentration mg/mL required for 50% inhibition of cell growth as compared to that of untreated control.
Result and Discussion
Chalcones are a major class of natural products and are considered as the precursors of flavonoids and isoflavonoids. Chemically, chalcones are 1,3-diaryl-2-propen-1-ones in which two aromatic rings are joined by a three carbon bridge having a carbonyl moiety and alpha,beta unsaturation (Aggarwal et al., 2005). In this study both series of indolyl chalcones found in good yields, which are in line with aforementioned references. All these indolyl chalcones were assayed for their in vitro cytotoxicity against human colon (Caco-2) cancer cell lines. The IC50 values were used to determine the growth inhibition of these cancer cell lines. From the IC50 values summarized in Table I, the compounds 1b, 1f and 1g have shown significant cytotoxicity. Furan moieties are common sub-structures in numerous natural products. Chalcone 1b bearing benzofuran ring is most active in this series and selectively cytotoxic against colon cancer cell lines (Caco-2) with an IC50 value of 7.4 µg/mL whereas compound 1f bearing benzyloxy group in the aromatic ring is as active as 1g, IC50 7.8 µg/mL against Caco-2 cancer cell line. Compound 1g with o-hydroxy group is moderately cytotoxic against all the caco2 cell lines without any selectivity. Introduction of m-allylic group in the aryl ring i.e. compound 1h is beneficial for the activity as compared to compound 1g.
Table I:In vitro cytotoxicity data of indolyl chalcones (1a-j)
Compounds | R | Caco-2 IC50 (µg/mL) |
---|---|---|
1a | 96 | |
1b | 7.4 | |
1c | 100 | |
1d | 52 | |
1e | NO | |
1f | 7.8 | |
1g | 7.8 | |
1h | 8.7 | |
1i | NO | |
1j | NO | |
Doxorubicin | 3.5 |
In other series, Compound 2i has displayed significant cytotoxicity against Caco-2 with an IC50 value of 7.4 µg/mL. Indolyl chalcone 2c with a 3,4,5-trimethoxy substituent and 2i with 3-ethoxy-4-hydroxy substituent were moderately active and selective against caco-2 with an IC50 value of 7.4 µg/mL (Table II).
Table II: In vitro cytotoxicity data of indolyl chalcones (2a-k)
Compounds | R | Caco-2 IC50 (µg/mL) |
---|---|---|
2a | NO | |
2b | NO | |
2c | 74 | |
2d | NO | |
2e | 88 | |
2f | NO | |
2g | 32 | |
2h | NO | |
2i | 7.4 | |
2j | NO | |
2k | NO | |
Doxorubicin | 3.5 |
In the study, we explored the orientations and binding affinities (in terms of total score). The docking reliability was validated by using the known crystallized X-ray structure of target protein LRH-1 complex with 3-bromo-5-phenyl-N-(pyridin-3-ylmethyl) pyrazolo [1,5- a]pyrimidin-7-amine. The co-crystallized structure was re-docked into the binding site and the docked conformation with the highest total score of 6.54 was selected as the most probable binding conformation. The low root mean-square deviation (RMSD) of 0.56 Angstrom between the docked and the crystal conformations indicates the high reliability of Surflex-dock software in reproducing the experimentally observed binding mode for doxorubicin. Redocked molecules were almost in the same position with co-crystallized at the active site of paclitaxel. Crystallography data CDK2 showed that the amino acid Asp-86 is the "gatekeeper" residue, an important determinant of inhibiting in the CDK2 binding pocket.
The docking results as shown for compounds 1b, 1f and 1g was docked at CDK2 binding pocket as shown docking score in the form of total score was i.e. 7.1740, 5.9001 and 6.3035 respectively. While, the docking scores of doxorubicin were 4.6772 only. The docked view of compounds 1b and 1g shows the formation of a hydrogen bond of length 2.0, 1.8 and 1.8 Angstrom to the polar hydrophobic residue Asp-86, Asp-145 and Asn-132. In docking pose, the conserved binding site pocket of amino acid residues within a selection radius of 3 Angstrom from bound ligand were hydrophobic residue Val-18 (valine), Phe-80, Phe-82 (phenylalanine), Asp-86, Asp-145 (aspartic acid), HIS-390 (histidine), Leu-83, Leu-134, (leucine), Ala-31, Ala-144 (alanine), Ile-10 (isoleucine), nucleophilic (polar, hydrophobic), i.e. Thr-14 (threo-nine) and polar amide, e.g. Gln-85, Gln-131 (glutamine) as a result as shown in Table III, bind compound showed a high interaction compare to with doxorubicin show more stability and activity in this compound. Overall, docking studies clearly indicates that compound 1b and 1g binds well (Figure 3) with CDK2 binding site and hence may exhibit similar inhibition effects on colon cancer receptor CDK2. These results were further substantiated by wet lab experiments.
Table III: Comparison of binding affinity of standard drug (control) anti-cancer drugs and active chalcone derivative against colon cancer receptor (PDB ID:2R3J)
Compound name |
Total score | Amino acid involved in active pocket in 3Angstrom |
Involved group of amino acid | Length of H-bond Angstrom‚ | Number of Hydrogen Bond |
---|---|---|---|---|---|
1b | 7.2 | Ile-10, Gly-11, Glu-12, Gly-13, Val-18, Ala-31, Phe-80, Phe-82, Leu-83, His-84, Gln-85, Asp-86, Lys-89, Lys-129, Gln-131, Asn-132, Leu-134, Ala-144 | Asp-86 | 2.0 | 1 |
1f | 5.9 | Ile-10, Val-18, Ala-31, Lys-33, Val-64, Phe-80, Glu-81, Phe-82, Leu-83, His-84, Leu-134, Ala-144, Asp-145 | - | - | - |
1g | 6.3 | Ile-10, Glu-12, Gly-13, Thr-14, Val-18, Ala-31, Lys-33, Val-64, Phe-80, Glu-81, Phe-82, Leu-83, Lys-129, Asn-132, Leu-134, Ala-144, Asp-145 | Asp-145 Asn-132 |
1.8 1.8 |
2 |
Doxorubicin | 4.7 | Ile-10, Val-18, Ala-31, Lys-33, Val-64, Phe-80, Phe-82, Leu-83, His-84, Gln-85, Asp-86, Lys-88, Lys-89, Gln-131, Leu-134, Ala-144 | Lys-89 Asp-86 Leu-83 |
2.0 1.9 2.1 |
3 |
Figure 3: In silico molecular docking studies elucidating the possible mechanisms of compound 1b and 1g induced modulation of colon cancer protein (CDK2) receptor (PDB: 2R3J). The docking studies were carried out using SYBYL-X 2.0, Tripos International. Compound 1b and 1g docked on CDK2 form a H-bond of length 2.0 and 1.8 Angstrom to the binding pocket residue Asp-86, Asp-145 and Asn-132 and total score 7.1740 (A) and 6.3035 (B) was observed
In our study, the ADME (absorption, distribution, metabolism and excretion) parameters were calculated for the active chalcone derivatives namely, compounds 1b, 1f and 1g. The values of these parameters also showed close correspondence with those of control compound doxorubicin and were within the standard range of values exhibited by 95% of all known drugs. Typically, low solubility is associated with bad absorption, so the general aim is to avoid poorly soluble compounds. The aqueous solubility (logS) of a compound significantly affects its absorption and distribution characteristics. The calculated logS values of the studied compounds were within the acceptable interval. Other calculations related to solubility, serum protein binding, the blood–brain barrier (log BB and apparent MDCK cell permeability), gut–blood barrier (Caco-2 cell permeability), predicted central nervous system activity, number of likely metabolic reactions, log IC50 for hERG K+ channel blockage, skin permeability (Kp), and human oral absorption in the gastrointestinal tract showed that these values for the active chalocone derivatives fell within the standard ranges generally observed for drugs (Table IV).
Table IV: Compliance of active indolyl chalcones to computational parameters of pharmacokinetics (ADME)
Compounds | log S for aq. solubility | log Khsa, serum protein binding | log BB for brain/ blood | Number of metabolic reactions | Predicted CNS activity | log HERG for K+ Channel Blockage | Apparent Caco-2 permeability nm/sec | Apparent MDCK Permeability nm/sec | log Kp for skin permeability | % Human oral absorption in GI (+-20%) | Qual model for human oral absorption |
---|---|---|---|---|---|---|---|---|---|---|---|
1b | -5.293 | 0.825 | -0.506 | 2 | -1 | -6.390 | 2157.263 | 1135.692 | -0.365 | 100 | High |
1f | -4.432 | -4.432 | -0.410 | 1 | -1 | -6.179 | 1783.35 | 924.50 | -1.001 | 100 | High |
1g | -3.688 | 0.303 | -0.607 | 1 | -1 | -5.424 | 1136 | 568 | -1.521 | 100 | High |
Doxorubicin | -2.162 | -0.628 | -2.633 | 9 | -2 | -5.916 | 3.982 | 1.394 | -7.423 | 0 | Low |
Stand. Range* | (-6.5 / 0.5) | (-1.5 / 1.5) | (-3.0 / 1.2) | (1.0 / 8.0) | <2 in-active+2 active | concern below -5 | <25 poor, >500 great | <25 poor, >500 great | –8 to –1, Kp in cm/hr | <25% is poor | >80% is high |
Toxicity screening results showed that compounds 1b, 1f and 1g possess risk of mutagenicity toxicity, however indicate significant docking and experimental based anti-cancer activity (Table V). Thus, there is a need for more qualitative safety evaluation of chalcone. This is particularly important because of the fact that chalcone derivatives are used very frequently in clinical and non-clinical settings. The compliance of active chalcone derivatives namely, compounds 1b, 1f and 1g with computational toxicity risks parameters indicate that these compounds are active and safe except mutagenicity toxicity risk at high doses or long term use similar to standard anti-cancer drugs namely doxorubicin. Therefore lead optimization of these active chalcone derivatives is a subject of further research work. Results of ADMET revealed that the overall drug scores of predicted active compounds are comparable to that of standard drugs and also established through in vitro experimental data (Table I) tested in colon (Caco-2) cancer cell line.
Table V:Compliance of active indolyl chalcones to computational toxicity risks parameters (i.e., mutagenicity, tumorogenicity, irritation and reproduction)
Compounds | Toxicity risk parameters | |||
---|---|---|---|---|
Compounds | MUT | TUMO | IRRI | REP |
1b | High risk | No risk | No risk | No risk |
1f | High risk | No risk | No risk | No risk |
1g | High risk | No risk | No risk | No risk |
Doxorubicin | No risk | No risk | No risk | No risk |
Conclusion
The indolyl chalcone analogues synthesized by various methods are potential candidates for further investigation towards the management of colon cancer.
References
Aggarwal BB, Ichikawa H. Molecular targets and anti-cancer potential of indole-3-carbinol and its derivatives. Cell Cycle. 2005; 4: 1201-15.
Agarwal A, Srivastava K, Puri SK, Chauhan PMS. Synthesis of substituted indole derivatives as a new class of antimalarial agents. Bioorg Med Chem Lett. 2005; 15: 3133.
Alqasoumi S I, Al-Taweel, AM, Alafeefy A M, Noaman E, Ghorab MM. Novel quinolines and pyrimido[4,5-b]quinolines bearing biologically active sulfonamide moiety as a new class of antitumor agents. Eur J Med Chem. 2010; 45: 738.
Ali Mohammad, Fauzia Bano Faruqi, Jamal Mustafa. Cancer: Possible prevention and chemotherapy by fatty materials: A review. Arc Apl Sci Res. 2009; 1: 178-99.
Alqasoumi SI, Al-Taweel AM, Alafeefy AM, Hamed MM, Noaman E, Ghorab MM. Synthesis and biological evaluation of amino-7,7-dimethyl-4-substituted-5-oxo-1-(3,4,5-trimethoxy)-1,4,5,6,7,8-hexahydro-quinoline-3-carbonitrile derivatives as potential cytotoxic agents. Bioorg Med Chem Lett. 2009; 19: 6939.
Bhagat S, Sharma R, Sawant DM, Sharma L, Chakraborti AKJ. LiOH·H2O as a novel dual activation catalyst for highly efficient and easy synthesis of 1,3-diaryl-2-propenones by Claisen–Schmidt condensation under mild conditions. J Mol Catal A: Chem. 2006; 244: 20.
Boeck P, Falca CAB, Leal PC, Yunes PA, Filho VC, Torres-Santos EC, Synthesis of chalcone analogues with increased anti-leishmanial activity. Bioorg Med Chem. 2006; 14: 1538–45.
Black DS, Renu BD, Kumar N. Synthesis of indol-3-yl-substituted 1-pyrroline 1-oxides. Aust J Chem. 1992; 45: 611.
Manna F, Chimenti F, Bolasco A, Bizzarri B, Filippelli W, Filippelli A, Gagliardi. L. Anti-inflammatory, analgesic and antipyretic 4,6-disubstituted 3-cyano-2-aminopyridines. Eur J Med Chem. 1999; 34: 245.
Cheng Y, An LK, Wu N, Wang XD, Bu XZ, Huang ZS, Gu LQ. Synthesis, cytotoxic activities and structure–activity relationships of topoisomerase I inhibitors: Indolizino-quinoline-5,12-dione derivatives. Bioorg Med Chem. 2008; 16: 4617.
Grimes CA, Jope RS. The multifaceted roles of glycogen synthase kinase 3β in cellular signaling. Prog Neurobiol. 2001; 65: 391-426.
Cheenpracha S, Karalai C, Ponglimanont C, Subhadhirasakul S, Tewtrakul S. Anti-HIV- 1 protease activity of compounds from Boesenbergia pandurata. Bioorg Med Chem Lett. 2006; 14: 1710-14.
Dube D, Blouin M, Brideau C, Chan CC, Desmarais S, Ethier D, Falgueyret JP, Friesen RW, Girard M, Girard Y, Guay J, Riendeau D, Tagari P, Young RN. Quinolines as potent 5-lipoxygenase inhibitors: Synthesis and biological profile of L-746,530. Bioorg Med Chem Lett. 1998; 8: 1255-61.
Dominguez JN, Leon C, Rodrigues J, Gamboa de Dominguez N, Gut J, Rosenthal PJ. Synthesis and evaluation of new antimalarial phenylurenyl chalcone derivatives. J Med Chem. 2005; 48: 3654-58.
Grugni M, Cassin M, Colella G, De Munari S, Pardi G, Pavesi P. Indole derivatives with antitumor activity. WO/2006/066923.
Jeong TS, Kim KS, An SJ, Lee S, Lee WS. Novel 3,5-diaryl pyrazolines as humanacyl-CoA: Cholesterol acyltransferase inhibitors. Bioorg Med Chem Lett. 2004; 14: 2715.
Kim YH, Shin K J, Lee TG, Kim E, Lee MS, Ryu SH, Suh PG. G2 arrest and apoptosis by 2-amino-N-quinoline-8-yl-benzenesulfonamide (QBS), a novel cytotoxic compound. Biochem Pharmacol. 2005; 69: 1333-41.
Kumar D, Kumar NM, Akamatsu K, Kusaka E, Harada H, Ito T. Synthesis and biological evaluation of indolyl chalcones as antitumor agents. Bioorg Med Chem Lett. 2010; 20: 3916-19.
Larsen RD, Corley EG, King AO, Carrol JD, Davis P, Verhoeven TR, Reider PJ, Labelle M, Gauthier JY, Xiang YB, Zamboni RJ. Practical route to a new class of LTD4 receptor antagonists. J Org Chem. 1996; 61: 3398-405.
Mulvihill MJ, Ji QS, Coate HR, Cooke A, Dong H, Feng L, Foreman K, Franklin M R, Honda A, Mak G, Mulvihill KM, Nigro AI, O’Connor M, Pirrit C, Steinig AG, Siu K, Stolz KM, Sun Y, Tavares PA, Yao Y, Gibson NW. Novel 2-phenylquinolin-7-yl-derived imidazo[1,5-a]pyrazines as potent insulin-like growth factor-I receptor (IGF-IR) inhibitors. Bioorg Med Chem. 2008; 16: 1359-75.
Modzelewska A, Pettit C, Achanta G, Davidson NE, Huang P, Khan SR. Anti-cancer activities of novel chalcone and bis-chalcone derivatives. Bioorg Med Chem. 2006; 14: 3491-95.
Meng QC, Ni L, Worsencroft KJ, Ye J, Weingarten MD, Simpson JM, Skudlarek JW, Marino EM, Suen K Kunsch C, Souder A, Howard RB, Sundell CL, Wasserman MA, Sikorski JA. Carboxylated, heteroaryl-substituted chalcones as inhibitors of vascular cell adhesion molecule-1 expression for use in chronic inflammatory diseases. J Med Chem. 2007; 50: 1304-15.
Nerya O, Musa R, Khatib S, Tamir S, Vaya J. Chalcones as potent tyrosinase inhibitors: The effect of hydroxyl positions and numbers. Phytochemistry 2004; 65: 1384–95.
Nikolinakos P, Heymach JV. The tyrosine kinase inhibitor cediranib for nonsmall cell lung cancer and other thoracic malignancies. J Thorac Oncol. 2008; 3: 131-34
Prince HM, Bishton M, Panobinostat (LBH589): A novel pan-deacetylase inhibitor with activity in T cell lymphoma, hematology meeting reports. Vol. 3. Peter MacCallum Cancer Centre and University of Melbourne, Parkville, Australia, 2009, pp 33-38.
Roma G, Braccio MD, Grossi G, Mattioli F, Ghia M. 1,8-Naphthyridines IV. 9-substituted N,N-dialkyl-5-(alkylamino or cycloalkylamino) [1,2,4]triazolo[4,3-a][1,8]naphthyridine-6-carboxamides, new compounds with anti-aggressive and potent anti-inflammatory activities. Eur J Med Chem. 2000; 35: 1021–35.
Stu AW, Marby TJ. Flavanoid front exudates from two Jamaican ferns, Pityrogramma tartarea and P. calomelanos. Phytochemistry 1971; 10: 2812-17.
Srinivasan B, Johnson TE, Lad R, Xing C. Structure-activity relationship studies of chalcone leading to 3-hydroxy-4,3',4',5'-tetramethoxychalcone and its analogues as potent nuclear factor kappaB inhibitors and their anti-cancer activities. J Med Chem. 2009; 52: 7228.
Svetaz L, Tapia A, Lopez SN, Furlan RLE, Petenatti E, Pioli R. Antifungal chalcones and new caffeic acid esters from Zuccagnia punctata acting against soybean infecting fungi. J Agric Food Chem. 2004; 52: 3297–300.
Yang HM, Shin HR, Cho SH, Bang SC, Song GY, Ju JH. Structural requirement of chalcones for the inhibitory activity of interleukin-5. Bioorg Med Chem. 2007; 15: 104–11.
Yesuthangam Y, Pandian S, Venkatesan K, Gandhidasan R, Murugesan R. Photogeneration of reactive oxygen species and photoinduced plasmid DNA cleavage by novel synthetic chalcones. J Photochem Photobiol B: Biol. 2011; 102: 200–08.
Yoon H, Kim TW, Shin SY, Park MJ, Yong Y, Kim DW, Islam T, Lee YH, Jung KY, Lim Y. Design, synthesis and inhibitory activities of naringenin derivatives on human colon cancer cells. Bioorg Med Chem Lett. 2013; 23: 232-38.
Yadav DK, Kalani K, Singh AK, Khan F, Srivastava SK, Pant AB. Design, synthesis and in vitro evaluation of 18β-glycyrrhetinic acid derivatives for anti-cancer activity against human breast cancer cell line MCF-7. Curr Med Chem. 2014; 21: 1160-70.
Yadav DK, Kalani K, Khan F, Srivastava SK. QSAR and docking based semi-synthesis and in vitro cytotoxic evaluation of glycyrrhetinic acid derivatives against human lung cancer cell line A549. Med Chem. 2013; 9: 1073-84.
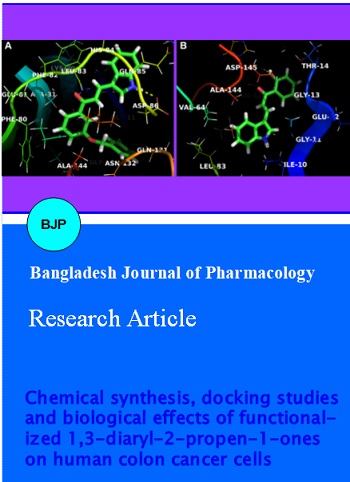