Cerebroprotective effect of Glycyrrhiza glabra Linn. root extract on hypoxic rats
Abstract
The present study was carried out to evaluate the cerebroprotective effect of the aqueous extract of the roots of Glycyrrhiza glabra Linn. (250 and 500 mg/kg) in hypoxic rats. Hypoxia was induced by providing sodium nitrite drinking water to rats for 14 days. Extract at the tested doses promoted the locomotor activity and spatial behavior significantly, which was impaired in hypoxic rats. The extract administration restored the decreased levels of brain enzymes such as glutamate and dopamine and decreased acetylcholinesterase (AChE) activity significantly. Levels of antioxidant enzymes such as superoxide dismutase, glutathione peroxidase, glutathione reductase and catalase were reduced due to hypoxia and were restored to near normalcy by administration of ethanol extract of G. glabra. Increased lipid peroxidation in hypoxic rats was also restored significantly by extract treatment. Thus, this study suggests that ethanol extract of G. glabra possess a cerebroprotective effect in hypoxic rats, which may be mediated by its antioxidant effects.
Introduction
Oxidative stress is implicated as one of the primary factors that contribute to the development of neurodegenerative diseases like, Alzheimer's, Parkinsonism and neurological conditions like epileptic seizures, stroke, brain damage, neurotrauma, hypoxia etc (Srinivasan, 2002). The highest degree of oxidative damage usually occurs in organs like brain, heart and skeletal muscles, since these organs are composed primarily of post mitotic cells. the central nervous system shows increased susceptibility to oxidative stress because of its high oxygen consumption rate (20% of the total oxygen inhaled by the body) that accounts for the increase in generation of oxygen free radicals and reactive oxygen substances like superoxide radical (O2), single oxygen (O2), H2O2 and hydroxyl radical (OH) (Srinivasan, 2002).
All the cells and tissues of our body are also equipped with anti oxidative enzymes like super oxide dismutase (SOD), glutathione peroxidase (GPX), glutathione reductase (GRD) and substances like reduced glutathione (GSH). They dispose the free radicals as and when they are generated there by protecting the cells and tissues from the oxidative attack. Normally the balance is maintained between the oxidative attack of the free radicals and the antioxidative defense system prevailing in the cells and tissues of our body (Srinivasan, 2002).
Glycyrrhiza glabra Linn. of the family Leguminosae, is a genus of perennial herbs and under shrubs distributed in the subtropical and warm temperate regions of the world, chiefly in the Mediterranean countries and China. The dried, peeled or unpeeled underground stems and roots constitute the drug known in the trade as liquorice (Anonymous, 1985).
The roots and rhizomes possess demulcent, anti-inflammatory, anti stress, anti depressive and expectorant property and useful in the treatment of peptic ulcer. Carbenoxolone, one of the oleandane derivatives from prepared from G. glabra possess considerable mineralocorticoid activity (Khare, 2007). The roots and rhizomes of G. glabra has been studied with respect to spatial learning and passive avoidance (Ravichandra et al., 2007); anxiolytic activity (Ambawade et al., 2001); memory enhancement (Dinesh et al., 2004); preliminary free radical scavenging (Toshio et al., 2003); cerebral ischemia (Zhan and Yang, 2006) and antioxidant capacity towards LDL oxidation (Vaya et al., 1998).
Accordingly, the present study was designed to explore the potential of G. glabra roots to protect brain from hypoxic damage induced by sodium nitrite.
Materials and Methods
Plant material
Roots of G. glabra were purchased from an herbal drug store in Chennai, Tamil Nadu, India during August 2007. The material was identified by Prof. P. Jayaraman, Director, Plant Anatomy Research Centre, Chennai. A voucher specimen was deposited in our laboratory for future reference.
Preparation of extract
The roots were shade dried and pulverized in a grinder to a coarse powder. Weighed quantity (1 kg) was passed through sieve number 40 and subjected to soxhlet extraction using distilled water. The extract was dried and weighed. A brownish black waxy residue with 21.1% w/w yield was obtained. This aqueous extract of G. glabra was used for animal administration.
Experimental animals
Inbred colony strains of Wister rats of either sex weighing 150-250 g procured from the animal house of C. L. Baid Metha College of Pharmacy were used for the study. The animals were maintained in polypropylene cages of standard dimensions at a temperature of 28 ± 1°C and standard 12:12 hours day/night rhythm. The animals were fed with standard rodent pellet diet (Hindustan Lever Ltd.) and water ad libitum. Prior to the experiment, the animals were acclimatized to the laboratory conditions. The experimental protocol was approved by Institutional Animal Ethical Committee (IAEC) constituted under CPCSEA. (IAEC Ref No: IAEC/XIII/06/CLBMCP/2007-08 dated 24-07-2007).
Preliminary phytochemical study
Preliminary phytochemical screening was performed as per procedure of Kokate et al. (2004).
Acute toxicity studies
Albino mice weighing 22-25 g selected by random sampling technique were used in the study. Acute oral toxicity was performed as per OECD-423 guidelines (Ecobichon, 1997). The animals were fasted overnight, provided only water after which aqueous extract was administered to the groups orally at the dose level of 5 mg/kg body weight by gastric intubation and the groups were observed for 14 days. If mortality was observed in 2 or 3 animals among 6 animals then the dose administered was assigned as a toxic dose. If mortality was observed in one animal, then the same dose was repeated again to confirm the toxic dose. If mortality was not observed, the procedure was repeated for further higher doses such as 50, 300 and 2,000 mg/kg body weight. The animals were observed for toxic symptoms such as behavioral changes, locomotion, convulsions and mortality for 72 hours.
Induction of hypoxia
Hypoxia was induced by administration of sodium nitrite water (30 mg/kg body weight of sodium nitrite dissolved in normal water) by gavage (5 mL/kg dosing volume) for 14 days except the control group, which was provided with normal water (Hirneth and Classen, 1984)
Experimental design
The animals were divided into 4 groups of 6 rats each as follows: Group I served as control and received normal saline. Group II served as hypoxic rats and received sodium nitrite water for 14 days. Group III animals received extract (250 mg/kg; p.o) suspended in 1% gum acacia and sodium nitrite water for 14 days. Group IV animals received extract (500 mg/kg; p.o) suspended in 1% gum acacia and sodium nitrite water for 14 days.
Locomotor activity
The locomotor activity was monitored by using actophotometer. The cognitive effect was measured by placing the animals in the actophotometer and the readings were recorded at 0, 30, 60, 90, 120 and 150 min for 10 min. The locomotor activity was expressed in terms of total photo beam interruption counts/min/animal (Turner, 1965).
Water maze task
The Morris water maze was performed using a circular water tank of standard dimensions (Morris, 1984). After several trails, the test was conducted on the 14 day of sodium nitrite water drink. The time required to escape on to the platform was recorded.
Estimation of dopamine and metabolic enzymes
On the day of experiment rats were sacrificed, whole brain was dissected out and separated the sub cortical region (including the striatum). Weighed quantity of tissue and was homogenized in 0.1 mL hydrochloric acid-butanol, (0.9 mL of 37% hydrochloric acid in one liter n-butanol for spectroscopy) for 1 min in a cool environment. The sample was then centrifuged for 10 min at 2,000 rpm. 0.08 mL of supernatant phase was removed and added to an Eppendorf reagent tube containing 0.2 mL of heptane (for spectroscopy) and 0.025 mL 0.1 M hydrochloric acid. After 10 min of vigorous shaking, the tube was centrifuged under same conditions to separate two phases. Upper organic phase was discarded and the aqueous phase (0.02 mL) was used for dopamine estimation (Schlumpf et al., 1974).
Estimation of dopamine To this phase 0.005 mL of 0.4 M hydrochloric acid and 0.01 mL EDTA/sodium acetate buffer (pH 6.9) were added, followed by 0.01 mL iodine solution (0.1 M in ethanol) for oxidation. The reaction was stopped after 2 min by addition of 0.01 mL Na2SO3 in 5 M NaOH (0.5 g Na2SO3 in 2 mL H2O + 18 mL 5 M NaOH). Acetic acid (0.01 mL, 10 M) was added 1.5 min later. The solution was then heated to 100°C for 6 min. when the sample again reached room temperature, excitation and emission spectra were read in the microcuvette. The readings were taken at 330-375 nm (Schlumpf et al., 1974).
Estimation of acetylcholinesterase (AChE)- 20 mg of brain tissue per mL of phosphate buffer (0.1 M; pH 8) was homogenized in a potter-elvehjem homogenizer. A 0.4 mL aliquot of brain homogenate was added to a cuvette containing 2.6 mL of 0.1 M phosphate buffer. 100 µL of dithiobisnitro benzoic acid reagent was added to the cuvette and the absorbance was measured at 412 nm. 20 µL of acetylcholine chloride was added. A change in the absorbance was recorded and the change in absorbance/min was calculated. It was expressed in µmol/min/g tissue (Ellman et al., 1961).
Brain glutamate estimation Weighed quantity of the brain portion was homogenized with 2 parts by weight of perchloric acid and centrifuged for 10 min at 3,000 rpm. 3 mL of supernatant fluid was adjusted to pH 9.0 with 1 mL phosphate solution. It was allowed to stand for 10 min in an ice bath and then filtered through fluted filter paper. Absorbance was measured at 340 nm. Similarly a blank reading at 340 nm was measured. The level of glutamate was expressed as µmol/g tissue (Bernt and Bergmeyer, 1965).
Estimation of antioxidant enzymes 100 mg of the brain tissue was weighed and homogenate was prepared in 10 mL tris hydrochloric acid buffer (0.5 M; pH 7.4) at 4°C. The homogenate was centrifuged and the supernatant was used for the assay of cytoprotective enzymes namely catalase (Aebi, 1983); glutathione peroxidase (Lawrence and Burk, 1976); superoxide dismutase (Marklund and Marklund, 1974); glutathione reductase (Dobler and Anderson, 1981) and lipid peroxidation (Luck, 1965).
Results
The preliminary phytochemical screening carried out on aqueous extract of G. glabra revealed the presence of phytoconstituents such as alkaloids, carbohydrates, saponins, triterpenoids, flavanoids and flavones.
The extract did not produce any toxic symptoms of mortality up to the dose level of 2,000 mg/kg body weight in rats, and hence the drugs were considered safe for further pharmacological screening. According to the OECD-423 guidelines for acute oral toxicity, the LD50 dose of 2000 mg/kg and above is categorized as unclassified.
There was an increase in escape latency in negative control group in the water maze task when compared with the control group (p<0.01). The groups treated with 250 mg/kg and 500 mg/kg aqueous extract showed the significant decrease in the escape latency (p<0.05 and p<0.01) respectively. The hypoxia induced group (negative control) indicated decrease in locomotor activity (p<0.01) in comparison with the control group (Table I).
Table I: Effect of G. glabra on water maze task
Group | Escape latency (sec) |
---|---|
I | 29.0 ± 4.1 |
II | 57.5 ± 8.2b |
III | 37.7 ± 2.5a |
IV | 30.6 ± 3.3b |
Values are mean ± SEM; n = 6; ap<0.05, bp<0.01 |
The extract treated groups exhibited significant (p<0.05 and p<0.01) increase in locomotor activity respectively for 250 mg/kg and 500 mg/kg of aqueous extract when compared with the negative control group (Table II).
Table II: Effect of G. glabra on locomotor activity
Group | Time | |||||
---|---|---|---|---|---|---|
0 min | 30 min | 60 min | 90 min | 120 min | 150 min | |
I | 375 ± 30.1 | 405 ± 36.4 | 401 ± 33.9 | 416 ± 20.3 | 425 ± 15.2 | 417 ± 9.5 |
II | 201 ± 15.5b | 224 ± 17.5b | 231 ± 16.3b | 239 ± 20.2b | 245 ± 18.5b | 257 ± 20.2b |
III | 260 ± 14.7a | 274 ± 10.6a | 291 ± 11.9ns | 320 ± 16.4a | 325 ± 16.5a | 350 ± 18.0a |
IV | 340 ± 27.4b | 390 ± 11.1b | 395 ± 28.1b | 410 ± 21.0b | 427 ± 17.9b | 429 ± 10.5b |
Values are mean ± SEM; n = 6; ap<0.05, bp<0.01, ns = not significant |
Induction of hypoxia significantly (p<0.01) increased the AChE activity and brain glutamate level when compared with control group. In the aqueous extract-treated groups, (250 and 500 mg/kg) there was a significant (p<0.01) reduction in enzyme levels when compared with the hypoxic group. The dopamine levels in negative control group was significantly decreased (p<0.01) when compared with the control group. The levels of dopamine in 250 and 500 mg/kg of aqueous extract treatment, significantly increased the reduced dopamine level (p<0.05 and p<0.01) respectively (Table III).
Table III: Effect of G. glabra on brain enzymes
Group | AChE (µmol/min/mg protein sec) | Glutamate (µmol/ g tissue) | Dopamine (pg/mg tissue) |
---|---|---|---|
I | 16.3 ± 0.1 | 73.8 ± 1.3 | 642.3 ± 14.6 |
II | 19.3 ± 0.1a** | 85.3 ± 1.5a** | 521.5 ± 10.6a |
III | 18.0 ± 0.1b* | 78.7 ± 1.3b** | 580.6 ± 11.9b* |
IV | 16.0 ± 0.1b | 74.5 ± 1.4b** | 592.5 ± 12.2b** |
Values are mean ± SEM; n = 6; *p<0.05, **p<0.01; aGroup I vs. Group II; bGroup II vs. Groups III and IV |
The levels of cytoprotective (antioxidant) enzymes such as super oxide dismutase, glutathione peroxidase, glutathione reductase and catalase were reduced (p<0.01) due to induction of hypoxia in Group II, with an increase in lipid peroxidation (p<0.01). Administration of aqueous extract increased the levels of the enzymes significantly (p<0.05 and p<0.01) for 250 and 500 mg/kg respectively. Lipid peroxidation was significantly decreased (p<0.01) by the administration of aqueous extract 250 and 500 mg/kg (Table IV).
Table VI: Effect of G. glabra on antioxidant enzymes
Parameter | Group I | Group II | Group III | Group IV |
---|---|---|---|---|
Superoxide dismutase (U/mg) | 8.1 ± 0.3 | 5.6 ± 0.1a** | 6.9 ± 0.4b* | 8.04 ± 0.3b** |
Catalase (µmol/mg) | 2.3 ± 0.03 | 1.6 ± 0.1a** | 1.9 ± 0.05b* | 2.2 ± 0.1b** |
Glutathione peroxidase (U/mg) | 34.1 ± 1.2 | 26.2 ± 1.0a** | 31.0 ± 0.7b* | 34.8 ± 1.4b** |
Glutathione reductase (U/mg) | 31.7 ± 0.4 | 24.7 ± 0.4a** | 27.9 ± 0.3b** | 32.1 ± 0.6b** |
Lipid peroxidation (nmol TABRS/mg) | 2.6 ± 0.04 | 4.4 ± 0.05a** | 3.8 ± 0.1b** | 2.2 ± 0.1b** |
Values are mean ± SEM; n = 6; *p<0.05, **p<0.01; aGroup I vs. Group II; bGroup II vs. Groups III and IV |
Discussion
The present study has revealed the neuroprotective effect of aqueous extract of G. glabra on sodium nitrite induced hypoxia deficits in rats. G. glabra is a medicinal plant with antioxidant properties. Previous studies show that oral administration for seven days protect against cerebral ischemia damage, cerebral energy metabolism, brain Na+K+ATPase activity, malondialdehyde content, total adenine nucleotides in dependent manner. Na+K+ATPase responsible for establishing the electrochemical gradient of Na+ and K+ ions across the cell membrane in central nervous system (Tao et al., 2001).
During hypoxia, changes will occur in the diffusion parameters of the extracellular space and extracellular concentration of energy–related metabolites and glutamate in rat cortex. Hypoxia in rat cortex has shown decrease in extracellular space volume and increase in tortuosity with in few minutes following cardiac arrest. Glucose levels were critical in regulating redox state during hypoxia; the cellular redox state was determined by increasing the reactive oxygen species. Supply of glucose increases cellular redox state and lowers the reactive oxygen species and cell death (Holingan and Jain, 2006).
Exposure to hypoxia alters the dendritic carbonization of hippocampal neurons and impairs the spatial learning and memory. Hypoxia will affect dendric morphology of the CA1 neurons. Exposure to more days result in significant reduction in branching points, intersections and dendric length in most of the segments, significantly elevated levels of calcium and protein synthesis may lead to delayed neuronal death in CA1 region after hypoxia. The CA3 cells have higher level of metabolic activity than the CA1 cells and this may make them more vulnerable to hypoxia (Titus et al., 2007)
Glutamate is a major excitatory neurotransmitter in brain. Down regulation of glutamate transporter expression and uptake, activity was observed during hypoxia. GABA levels were highly correlated with endogenous glutamate levels during hypoxia, it increases GABA levels when glutamate level raises above the normal level when glutamate level decreases GABA level decreases automatically inhibitors of glutamate decarboxylase and GABA transaminase suggested that increased synthesis and decreased catabolism may both contribute to increase in GABA levels during hypoxia (Ales et al., 2006). ATP depletion effects on the release and redistribution of glutamate and aspartate in rat hippocampal slices, glutamate is released during ATP depletion by reversal of cotransporters.
The treatment with aqueous extract ameliorated cognitive deficits in sodium nitrite drinked rats. In water-maze test consumption of aqueous extract decreased the escape latency almost to normal levels in dose-dependent manner; it is possible that neuroprotection plays a role in favorable effect of G. glabra on sodium nitrite induced cognitive effects.
The AChE activity has been shown to be increased with in and around hypoxic brain. The calcium influx followed by oxidative stress is involved in the increase in activity of AChE induced by sodium nitrite, decreasing cell membrane order and ultimately leading to the exposure of more active sites of the enzyme. Increasing in AChE activity and ROS production indicates that it can be possible to ameliorate cholinergic function by inhibiting sodium nitrite, which induces increase in AChE activity.
The AChE activity in the brain was increased in rats treated with sodium nitrite when compared with the normal; in addition, the sodium nitrite induced increase in AChE was attenuated by aqueous extract treatment.
Conclusion
This study suggests that markedly aqueous extract improves antihypoxic effects induced by sodium nitrite and this effect may be mediated by its antioxidant properties.
References
Aebi H. Catalase In: Methods in enzymatic analysis, Bergmeyer HU (ed), Vol 3. New York, Academic Press, 1983, pp 276-86.
Ales H, Norbert Z, Karel S. Changes in diffusion parameters energy related metabolites and glutamate in the rat cortex after transient hypoxia. Neurosci lett. 2006; 404: 137-42.
Ambawade, Kasture KD, Kasturi VS. Anxiolytic activity of Glycyrrhiza glabra Linn. J Nat Remedies. 2001; 2: 130-34.
Anonymous. The wealth of India Raw material, Vol X, (Sp-W), New Delhi, India, Publication and Information Directorate, 1995, p 281.
Bernt E, Bergmeyer HU. L-glutamate UV assay with glutamate dehydrogenase and NAD. In: Methods of enzymatic analysis. 2nd ed. New York, Academic Press, 1965, pp 1704-08.
Dinesh D, Milind P, Kulkarni SK. Memory enhancing activity of Glycyrrhiza glabra in mice. J Ethnopharmacol. 2004; 91: 361-65.
Dobler RE, Anderson BM. Simultaneous inactivation of the catalytic activities of yeast glutathione reductase by N-alkyl melimides. Biochem Biophys Acta. 1981; 70: 659.
Ecobichon DJ. The basis of toxicology testing. 2nd ed. New York, CRC Press, 1997, pp 43-60.
Ellman GL, Courtney KD, Anders U, Feather Stone RM. A new and rapid colorimetric determination of acetylcholinesterase activity. Biochem Pharmacol. 1961; 7: 88-95.
Hirneth H, Classen HG. Inhibition of nitrate-induced increase of plasma nitrite and ethemoglobin in rats by simultaneous feeding of ascorbic acid or tocopherol. Arzneimittelforschung 1984; 34: 988-91.
Holingan S, Jain KL. Effect of glucose concentration on redox status in rat primary cortical neurons under hypoxia. Neurosci Lett. 2006; 410: 57-61.
Khare CP (ed). Indian medicinal plants. 1 ed, Berlin, Heidelberg, Springer-Verlag, 2007, pp 289-90.
Kokate CK, Purohit AP, Gokhale SB. Analytical pharmacognosy In: Textbook of pharmacognosy. 29th ed. Pune, Nirali Prakasan, 2004, pp 108-09.
Lawrence RA, Burk RF. Glutathione peroxidase activity in selenium deficient rat liver. Biochem Biophys Res Comm. 1976; 71: 952-58.
Luck H. In: Methods of enzymatic analysis. 2nd ed. New York, Academic Press, 1965, pp 885-90.
Marklund S, Marklund G. Involvement of superoxide anion radical in autoxidation of pyrogallol and a convenient assay of superoxide dismutase. Eur J Biochem. 1974; 47: 469-74.
Morris R. Developments of a water maze procedure for studying spatial learning in the rat. J Neurosci Methods 1984; 11: 47-60.
Ravichandra V, Ahalyadevi, Adiga S. Evaluation of the effect of Glycyrrhiza glabra Linn root extract on spatial learning and passive avoidance response in rats. Indian Drugs 2007; 44: 214-19.
Schlumpf M, Lichtensteiger W, Langemann H, Waser PG, Hefti F. A fluorimetric micromethod for the simultaneous determination of serotonin, noradrenaline and dopamine in milligram amounts of brain tissue. Biochem Pharmacol. 1974: 23: 2337-46.
Srinivasan V. Melatonin oxidative stress and neuro-degenerative diseases. Indian J Exp Biol. 2002; 40: 668-79.
Tao F, Lu SD, Zhang LM, Huang YL. Role of excitatory amino acid transporter in neonatal rat neuronal damage induced by hypoxia. Neuroscience 2001; 102: 503-13.
Titus ADJ, Harsha HN, Ramkumar K. Hypobaric hypoxia induced dendric atrophy of hippocampal neurons is associated with cognitive impairment in adult rats. Neuroscience 2007; 145: 265-78.
Toshio F, Kazue S, Taro N. Preliminary evaluation of anti nephritis and radical scavenging activities of glabridin from Glycyrrhiza glabra Linn. Fitotherapia 2003; 74: 624-29.
Turner RA. Depressants of the central nervous system, In: Screening methods in pharmacology. Turner RA (ed). Vol 1. New York, Academic Press, 1965, pp 69-86.
Vaya J, Belinky PA, Aviram M. Structural aspects of the inhibitory effect of glabridin on LDL oxidation. Free Rad Biol Med. 1998; 24: 1419-29.
Zhan C, Yang J. Protective effects of isoliquiritigenin in transient middle cerebral artery occlusion induced focal cerebral ischemia in rats. Pharmacol Res. 2006; 53: 303-09.
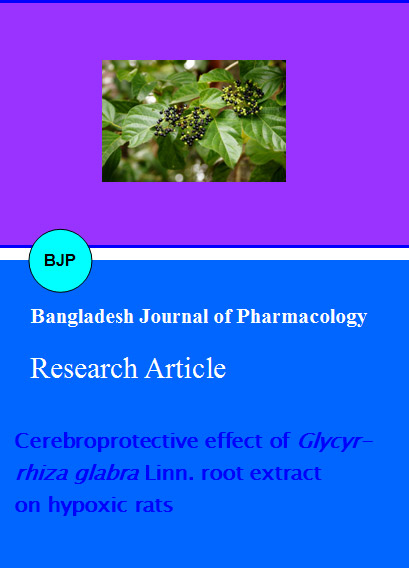