In vitro anthelmintic effects of Artemisia afra and Mentha longifolia against parasitic gastro-intestinal nematodes of livestock
Abstract
Gastrointestinal nematodes are the most widespread and economically important class of the helminths. The major threat posed by nematode infections is the productivity loss due to their ability to disrupt nutrient utilization of the livestock resulting in hunger and malnutrition. Acetone and aqueous extracts were prepared from Mentha longifolia and Artemisia afra and tested on egg hatch, larval development and larval mortality assays at three concentrations 2.5, 5.0 and 7.5 mg/mL. Certain percentages of egg hatch and larval mortality were inhibited by both plants; however, larval development was totally inhibited at all concentrations as compared to the negative control. Generally the acetone extracts from the two plants were most effective against parasitic gastrointestinal nematodes of livestock. This report therefore validates the folkloric use of these species in the management of internal parasites by the Basotho of the eastern Free State Province of South Africa.
Introduction
Gastrointestinal nematodes are mostly known to invade the digestive tract, the abomasums or even the small intestines (Maphosa and Masika, 2009). They are the worst parasites for livestock production worldwide (Diehl et al., 2004). They totally depend on their host for nourishment, nutrition and protection (Tsotetsi and Mbati, 2003). Nematode infection is therefore of economic importance for it is a major cause of disease and results in decreased productivity. All types of livestock are at risk to gastrointestinal nematode infection which varies in their host range, life cycle and severity. However, small stock is the most susceptible to gastrointestinal nematode infections (Tsotetsi and Mbati, 2003).
Nematode infection results in several clinical symptoms, however, in most cases no symptoms develop under high quantity of gastrointestinal nematodes in a single host. Some species of nematodes such as the Haemonchus and Trichostrongylus are blood sucking parasites whose activity usually results in anemia. Most common symptoms of nematode infection are irritation, loss of appetite, inflammation of the mucous membrane, diarrhea, loss of body mass, and death (Hendrix, 1998; Hunter, 1996). High rate of infection can cause reduction feed intake, loss of condition, tissue damage, weight loss, skin pealing and eventually death (Bogish and Cheng, 1990). Another major effect of high infection level is mortality and the survivors are less fertile and produce less milk, less quality of meat, less wool and lower calf birth percentage (Hunter, 1996; Diehl et al., 2004).
Commercially available anthelmintics have widely been used for a long time throughout the world to minimize the loss due to helminth infection. From the usage of the anthelmintic drugs, livestock production obtained considerable benefits. These drugs may not have been effective enough to totally cure the infection but at least they reduced the aggressiveness of the disease (Eguale et al., 2011). These treatments are however synthetic, they require chemicals to be synthesized (Pessoa et al., 2002). Apart from these synthetic anthelmintics, there are no other known treatments. However, anthelmintic drugs are expensive; it therefore takes a huge amount of money to combat helminth infections in livestock. Furthermore, there are specific stores where anthelmintic drugs are available, not every farmer particularly those in villages have access to them since they are mostly sold at the agriculture or veterinary services in bigger towns or cities (Eguale et al., 2011, Tsotetsi and Mbati, 2003). Otherwise, repeated administration of anthelmintic drugs is required in treating the internal parasites (Kamaraj and Rahman, 2010; Ademola and Eloff, 2011).
Nematodes are well documented for their good adaptability to various environments. Repeated administration of anthelmintic drugs leads to the development of nematode resistance against the drugs (Kotze et al., 2005). Nematode infections on livestock is increasing due to built-up resistance against anthelmintics and it only means that livestock is getting much susceptible to the infections (Hunter, 1996). According to Eguale et al. (2011), farmers started using the ethnoveterinary medicine as an alternative and a suitable way of treating gastrointestinal nematodes when they could not access the anthelmintic drugs due to their high costs. Medicinal plants are not synthetic, they are accessible, affordable and environmentally friendly (Pessoa et al., 2002). Medicinal plants also provide a potential of new pharmaceutical substances (Diehl et al., 2004). Other alternative ways of treating parasitic nematode infections have been taken into consideration as well, however, not much has been said about them. Diehl et al. (2004) suggested other possible ways such as breeding the resistant livestock against nematodes or improving food quality, whilst Ademola and Eloff (2011) suggested prevention, control and treatment. However, there are obstacles to these suggestions; nematodes have their way of adapting to a new environment. It is quite hard to predict the infected grazing areas and treating hectares of land with anthelmintic drugs would be a huge waste of time and money.
Medicinal plants on the other hand have not yet been documented with any resistance development of the gastrointestinal nematodes. Despite the huge dependence of farmers in the areas on the use of these species in the management of livestock diseases among others, there is dearth of information in the scientific literature validating these folkloric claims. The overall aim of the present study was to investigate the anthelmintic activity of A. afra and M. longifolia in order to validate their use in ethnoveterinary medicine of the eastern Free State Province of South Africa.
Materials and Methods
Sheep faecal sample collection: Faecal samples were collected on several occasions from a maximum of 50 sheep belonging to the Seotlong Agriculture and Hotel School at Qwaqwa in Maluti-A-Phofung Municipality of the eastern Free State Province of South Africa. Samples were collected directly from the recta of animals (Reinecke, 1983) and transported to the parasitology laboratory of the University of the Free State, Qwaqwa Campus in a cooler box.
Diagnostic methods: The McMaster technique (Soulsby, 1982) was used for nematode egg diagnosis in this study. Briefly, two grams of faecal samples were weighed, and 58 mL of 40% sugar solution was added to sheep feces as a flotation medium. Samples were thoroughly crushed and dissolved using a blender. Two chambers of a McMaster slide were filled with a Pasture pipette. The slides were allowed to stand for about 4 min so that the eggs can float on the surface of the flotation medium and to lie in contact with the upper glass of the chamber. A dissecting microscope (Nikon Eclipse E100 Company, Japan) was used for egg detection and egg counts in the two McMaster slide grids. The eggs of the not easily differentiated genera such as the Haemonchus, Trichostronglylus, Oesophagostomum and Chabertia were counted together, and recorded separately to those that are easily distinguished such as the Nematodirus, Strongyloides, Trichuris and Coccidia. Two McMaster chambers were filled and counted; the egg per gram counts of feces were done by counting all the eggs in the chambers of the slide and multiplied by 100 for sheep.
Plant material and preparation of extracts: Plant material were collected in May 2012 from a multiple populations of Artemisia afra and from a single population of Mentha longifolia around Qwaqwa township in Maluti-A- Phofung Municipality of the eastern Free State Province (28°32'0"S and 28°49'0" East; altitude 1, 673 m). The mean annual rainfall of the area is about 653 mm per annum with temperatures ranging from as low as 0.1ºC during July nights, 14.2°C during the day in June and maximum of 24.7ºC during the day in January. The plants were authenticated by Dr. AOT Ashafa of the Department of Plant Sciences; University of the Free State (UFS) Qwaqwa Campus and voucher specimen (MolMed/01/2012) was prepared and deposited at the UFS Qwaqwa Campus herbarium.
The shoots of the plants were separated and dried in an Ecortherm oven (Laboratory Consumables Pty, South Africa) at a temperature of 42°C to a constant weight before it was pulverized. Ten grams each of powdered material was extracted in acetone and distilled water. All extracts were filtered using Whatman No-1 filter paper (Whatman, United Kingdom). The filtrates from acetone were concentrated under reduced pressure 40ºC using rotary evaporator (Cole-Parmer, Laboratory Consumable and Chemical Supplies Co.Ltd, China). Acetone was of high analytical grade (Merck Chemicals Pty, Wadeville, South Africa). The water extract was freeze dried using freeze dryer (Virtis SP Scientific, United States of America). Yields of 0.8 g and 2.8 g for A. afra and 1.4 g and 1.9 g for M. longifolia acetone and water extracts respectively. Individual extract was reconstituted in their respective solvent to give a stock solution of 50 mg/mL (Ashafa and Afolayan, 2009). This was diluted to the required concentrations of 2.5, 5.0, 7.5 mg/mL for the bioassay analysis.
Egg recovery assay: Egg recovery was conducted as described by Maphosa et al. (2010) with some modifications. Four grams of collected faecal pellets were weighed, water was slowly added to the faeces and pellets mashed until a relatively liquid suspension (slurry) was obtained. The slurry was then filtered through sieves of 117, 70 and 25 μm. The contents of 25 µm sieve were backwashed with distilled water and transferred into 60 mL centrifuge tubes. The suspension was allowed to stand for 30 min, supernatant was decanted and the sediments suspended in 40% sugar solution. The suspension was transferred into another set of tubes, allowed to stand for another 30 min and the supernatant washed through a 25 μm pore mesh sieve using distilled water. The eggs were then washed off from the 25 um sieve with distilled water into a 1 liter conical flask where they were allowed to sediment for 2 hours. The concentration of eggs were estimated by counting the number of eggs in 2 aliquots of 0.5 mL of the suspension in a microscope slide, repeated and the mean number of eggs per 0.5 mL was determined.
Egg hatch assay: The egg hatch assay was conducted as published by McGaw et al. (2007) and Bizimenyera et al. (2006). The counted number of eggs in a 0.5 mL of egg suspension was pipetted into a 96-well microtitre plate. Wells 1 to 3 were used for A. afra experiments, well 5 to 7 for M. longifolia experiments and wells 9 to 11 were used for both negative and positive controls. In addition, 0.5 mL of plant extract at different concentrations of 7.5, 5 and 2.5 mg/mL were added. A commercial anthelmintic drug Tramisol® (Levamisole, Afrivet, South Africa) was used as the positive control at the same concentrations and distilled water used as a negative control. All tests were repeated 3 times.
The plate was incubated under humidified conditions at 25ºC temperature for 48 hours thereafter a drop of Lugol's iodine solution was added to each well to stop further hatching. All unhatched eggs and L3 larvae were then counted.
Inhibition percentages were calculated using a formula by Cala et al., 2012.
(Eggs + L1) - L1
E = x 100
Eggs + L1
Larval development assay: The larval development assay was conducted as described by Bizimenyera et al. (2006). The counted number of eggs in a 0.5 mL of the egg suspension was put into each well in a 96-microtitre plate with a 100 uL of lyophilized penicillin-streptomycin to combat fungal growth. The contents of the wells were then mixed, and the plates placed in an incubator under humidified conditions at ambient temperature for 48 hours for incubation of the eggs. After 48 hours, 0.5 mL of the water and acetone extracts of A. afra and M. longifolia as well as Tramisol® (Levamisole, Afrivet, South Africa) as a positive control at 7.5, 5 and 2.5 mg/mL were added to respective plates. The negative control plates received 0.5 mL of distilled water. All experiments were replicated three times. Incubation of the plates was continued for 5 days, after which all the plates were examined to determine the survival of larvae at different concentrations. All the L₃ stage larvae in each well were counted and a percentage inhibition of larval development was calculated using the formula (Cala et al., 2012):
(L1 + L2‚ + L3) - L3
E = x 100
L1 + L2‚ + L3
Larval mortality assay: The larval mortality assay was conducted adopting the method described by McGaw et al. (2000) and Zafar et al. (2006) with slight modifications. In vitro cultures were prepared from nematode eggs from microscopically positive faecal samples of sheep. After seven days of incubation the eggs hatched into larvae. L3 larvae were obtained from the in vitro cultures prepared and poured into a single petri dish. The 150 µL L3 larvae were placed in microtitre plate and crude extracts of the same volume were added at different concentrations, Tramisol® was used as positive control and distilled water as a negative control. After the addition of extracts, larval counts were done on daily basis and the larval mortality rates were recorded until all the larvae had died. All tests were repeated three times. All live and motile L3 stage larvae in each well were counted and a percentage inhibition of larval development was calculated using the formula described by Coles et al. (1992) and modified by Bizimenyera et al., 2006 with slight modifications:
Inhibition percentage (%) = 100 (1-X1/X2)
Where X1 is the initial number of larvae in test extracts pre-treatment, and X2 is the number of larvae obtained post-treatment.
Statistical analysis: Kruskal-Wallis test was used to analyse the egg hatch and larval mortality assays in order to determine if there was significant difference in the hatched eggs and larval mortality from different treatments with different concentrations.
Results
The best results were obtained from the lowest concentration (2.5 mg/mL) of A. afra water and acetone extracts. At 2.5 mg/mL the water extract exhibited 13.5% while the acetone extract at the same concentration showed 30.1% inhibition of nematode egg hatchability (Table I). Similar to A. afra, M. longifolia acetone extract at 2.5 mg/mL suppressed the hatching of nematode eggs with 26.1% but water extract at the highest concentration (7.5 mg/mL) inhibited less than 2% eggs from hatching (Table II).
Table I: Effects of A. Afra extracts on egg hatch on gastrointestinal nematodes of livestock
Water extracts | Acetone extracts | Controls | ||||||
---|---|---|---|---|---|---|---|---|
Concentrations (mg/mL) | 2.5 | 5.0 | 7.5 | 2.5 | 5.0 | 7.5 | Positive | Negative |
No of eggs pre-treatment | 39.3 | 68.0 | 37.3 | 34.3 | 26.7 | 40.0 | 63.3 | 25.7 |
No. of eggs post-treatment | 5.3 | 1.7 | 3.7 | 10.3 | 5.3 | 9.0 | 63.3 | 0.0 |
No. of un-hatched eggs | 34.0 | 66.3 | 33.7 | 24.0 | 21.3 | 31.0 | 0.0 | 25.7 |
Inhibition percentages | 13.5 | 2.5 | 9.8 | 30.1 | 2.0 | 22.5 | 100.0 | 0.0 |
Table II: Effects of M. longifolia extracts an egg hatch of gastrointestinal nematodes of livestock
Water extracts | Acetone extracts | Controls | ||||||
---|---|---|---|---|---|---|---|---|
Concentrations (mg/mL) | 2.5 | 5.0 | 7.5 | 2.5 | 5.0 | 7.5 | Positive | Negative |
No of eggs pre-treatment | 33.7 | 49.3 | 64.0 | 23.0 | 23.3 | 36.0 | 63.3 | 25.7 |
No. of eggs post-treatment | 0.3 | 0.3 | 0.7 | 6.0 | 5.3 | 5.3 | 63.3 | 0.0 |
No. of un-hatched eggs | 33.3 | 49.0 | 63.3 | 17.0 | 18.0 | 30.7 | 0.0 | 25.7 |
Inhibition percentages | 1.0 | 0.7 | 1.0 | 26.1 | 22.8 | 14.8 | 100 | 0.0 |
A 100% larval development was inhibited at all concentrations of A. afra tested in this study. At 2.5 mg/ mL both the water and acetone extracts compared with the standard commercial drug (Tramisol). In a similar pattern, M. longifolia water and acetone extracts displayed 100% larval development inhibition at all concentrations tested.
The larval mortality assays of both water and acetone extracts from A. afra showed that the extracts at 2.5 mg/ mL exhibited 72.6 and 70.5% mortality rate, respectively at 24 hours. Generally, larval mortality rate was time dependent in both extracts, water extracts had a 100% mortality rate within 120 hours, whereas the acetone extended to 168 hours at 7.5 mg/mL and 192 hours at 2.5 and 5.0 mg/mL (Table III). The water extracts of M. longifolia at 2.5 mg/mL had 71.8% larval mortality rate at 24 hours, however, 100% mortality was observed at 120 hours. Contrary to what was obtained in the water extract, the acetone extract at 2.5 mg/mL showed 15.4% at 24 hours but surprisingly the total mortality of the larvae was observed at 120, 192 and 168 hours for 2.5, 5.0 and 7.5 mg/mL, respectively (Table IV).
Table III: Effects of A. afra extracts on larval mortality of gastrointestinal nematodes of livestock
Time (hours) |
Water extracts | Acetone extracts | Controls | |||||
---|---|---|---|---|---|---|---|---|
2.5 mg/mL | 5.0 mg/mL | 7.5 mg/mL | 2.5 mg/mL | 5.0 mg/mL | 7.5 mg/mL | Positive (Tramisol) |
Negative (Water) |
|
0 | 0.0 | 0.0 | 0.0 | 0.0 | 0.0 | 0.0 | 0.0 | 0.0 |
24 | 72.6 | 37.5 | 42.0 | 70.5 | 64.9 | 21.8 | 100.0 | 0.0 |
48 | 99.2 | 79.7 | 96.3 | 84.2 | 79.4 | 63.7 | 100.0 | 0.0 |
72 | 99.3 | 80.6 | 85.7 | 89.7 | 87.3 | 89.2 | 100.0 | 0.0 |
96 | 99.5 | 95.7 | 98.0 | 95.5 | 92.8 | 95.1 | 100.0 | 0.0 |
120 | 100.0 | 100.0 | 100.0 | 97.5 | 89.4 | 91.1 | 100.0 | 0.0 |
144 | NDa | NDa | NDa | 99.6 | 92.7 | 98.5 | 100.0 | 0.0 |
168 | NDa | NDa | NDa | 99.9 | 97.9 | 100.0 | 100.0 | 0.0 |
192 | NDa | NDa | NDa | 100.0 | 100.0 | NDb | 100.0 | 0.0 |
NDa = Not done (Because all larvae died within 120 hours); NDb = Not done (Because all larvae died within 168 hours) |
Table IV: Effects of M. longifolia extracts on larval mortality of gastrointestinal nematodes of livestock
Time (hours) | Water extracts | Acetone extracts | Controls | |||||
---|---|---|---|---|---|---|---|---|
2.5 mg/mL | 5.0 mg/mL | 7.5 mg/mL | 2.5 mg/ mL | 5.0 mg/ mL | 7.5 mg/ mL | Positive (Tramisol) | Negative (Water) | |
0 | 0.0 | 0.0 | 0.0 | 0.0 | 0.0 | 0.0 | 0.0 | 0.0 |
24 | 71.8 | 64.4 | 49.1 | 15.4 | 43.8 | 41.2 | 100.0 | 0.0 |
48 | 84.0 | 83.3 | 83.2 | 70.9 | 86.1 | 81.0 | 100.0 | 0.0 |
72 | 85.8 | 79.3 | 75.2 | 87.6 | 90.2 | 84.5 | 100.0 | 0.0 |
96 | 98.0 | 99.1 | 98.5 | 91.1 | 92.1 | 96.7 | 100.0 | 0.0 |
120 | 100.0 | 100.0 | 100.0 | 100.0 | 99.2 | 99.3 | 100.0 | 0.0 |
144 | NDa | NDa | NDa | NDa | 98.3 | 98.5 | 100.0 | 0.0 |
168 | NDa | NDa | NDa | NDa | 99.3 | 100.0 | 100.0 | 0.0 |
192 | NDa | NDa | NDa | NDa | 100.0 | NDb | 100.0 | 0.0 |
NDa = Not done (Because all larvae died within 120 hours); NDb = Not done (Because all larvae died within 192 hours) |
Discussion
The rate of development and longevity of nematode eggs and larvae vary with temperature, rainfall and humidity in different geo-ecological regions (Tembely, 1998). According to Hounzangbe - Adote (2005), the egg is the stage disseminated into the environment hence is protected by a thick wall making it resistant to unfavorable conditions such as the climatic and environmental conditions and can therefore remain infective for a long period of time, several years to be exact.
Results obtained from the present study showed that low level of egg hatch inhibition in treatments, which could be attributed to the reported ability of nematode eggs to withstand adverse conditions (Salih, 2003). The reason why eggs are not susceptible to medicinal components as compared to the larval development is because during the experiment larvae are exposed to the medicinal components as compared to eggs (Molan et al., 2003). The eggs are only exposed to the components for 24 to 48 hours whereas the larval stages L1 and L2 are exposed to medicinal component for 5 to 7 days. L1 and L2 stage larvae are the feeding stages, and therefore they could even ingest or absorb medicinal components (Ferhat et al., 2011).
The results from the present study showed acetone extracts led to high egg hatch inhibition percentages than water extracts. This indicates that acetone is more effective in extracting the anthelmintic compounds in the two plant materials, and has previously been reported as a good extractant for anthelmintic compounds of plant origin (Suteky et al., 2011). According to Rao et al. (2002) during water extraction or water distillation not all of the medicinal components are dissolved but others are condensed into a hydrosol which is discarded at the later state of extraction, therefore during water extract some components that did not dissolve
are lost. The inability of water extracts to supress microbial infections have been widely reported (Lewu et al., 2006; Koduru et al., 2006; Ashafa et al., 2008; 2009), but little is known about the activity of water extracts against nematode infection. Traditionally, plant extracts are prepared with water as infusions, decoctions and poulties, however it seems unlikely that water is able to extract those compounds which are responsible for activity in the acetone extracts (Ashafa et al., 2008).
In the present study, there was no larval development from L1 to L3 as all died as first stage larvae without developing to the infective stage (L3 larvae) in all the treatment extracts. The first stage larvae are the more sensitive ones to medicinal components than eggs (Cala et al., 2012).
The infective larvae, L3 larvae, of nematode species have high capacity to survive even in adverse conditions. High temperatures with high rainfall favour the development of the larvae from the pre-infective stage (L1 stage larvae) to infective stage (L3 larvae) throughout the year (Bizimenyera et al., 2006). The results from our study showed that water extracts from both A. afra and M. longifolia were much effective than acetone extracts against the larval mortality. Eguale et al., (2011) stated that the difference in effectivity of the extract solvent might be due to the variation in solubility of active constituents. Acetone is a suitable extractant as it extracts compounds of a wide variety of polarity range (Bizimenyera et al, 2006), however, nematodes have been documented with the ability to quickly adapt to their environments, therefore it is either the acetone extracted components provided a suitable medium for the survival of the larvae or the larvae quickly adapted to the extracts.
The M. longifolia has been documented with menthone, 1.8 cineole, pulegone, caryophyllene and sesquiterpenes (Asekum et al., 2007) while A. afra was documented with artemisin which actually protects the plant against bacteria, viruses, insects and herbivores by making the plant unpalatable with an unpleasant flavour (Nibret and Wink, 2010) other components of A. afra are camphor, cinnamate, davanone, thujone, alkaloids, flavonoids and tannins which have been reported to possess strong insecticidal compound with mutagenic activity (Chauhan et al., 2009). The anthelmintic effectiveness of medicinal extracts from both plants may be due to their phytochemical composition which might be from single or combined effect of the compounds or chemical groups. Ferhat et al. (2011) stated that medicinal compounds might interfere with the osmotic balance of the worm hence death will result. There are two mechanisms of nematode uptake of drugs, it can either be diffusion of the drug through the eggshell or through the larval cuticle or by diffusing through the intestinal cells (Suteky et al., 2011; Eguale et al., 2007). The presence of tannins in plants is characterized with the connection to free proteins or connection to the larval cuticle reducing the availability of nutrients therefore resulting in death of the larvae due to starvation; this is because tannins are rich in glycoproteins (Cala et al., 2012).
The anthelmintic activity exhibited by A. afra and M. longifolia crude extracts against gastrointestinal nematodes of sheep (Haemonchus, Oesophagostomum, Ostertagia, Chabertia, Nematodirus, Trichostrongylus/Telardosagia, Bunostomum,) in this study has a significant importance considering the worldwide emergence of anthelmintic resistance. Based on the results obtained from this study it can be concluded that the tested medicinal plant extracts showed significant in vitro anthelmintic activity at different concentrations and therefore, the extracts can be considered larvicidal. The two plants tested are readily available in the eastern Free State Province, where they can be easily accessed by local farmers. According to South African National Biodiversity Institute report (2012), it is interesting to note that these plants are not endangered but endemic to this part of the country.
Conclusion
M. afra and M. longifolia are therefore good candidates for treatment of gastrointestinal larval infections. However, the mechanisms of their effectiveness still remain to be tested in vivo. Furthermore, safety and toxicity studies must be conducted in vivo to determine the minimum non-lethal concentrations needed for the treatment of nematode infections.
Acknowledgement
We are grateful to Seotlong Agriculture and Hotel School for cooperation during sampling.
References
Ademola IO, Eloff JN. Anthelminthic activity of acetone extract and fractions of Vernonia amygdalina against Haemonchus contortus eggs and larvae. Trop Health Prod. 2011; 43: 521-27.
Asekum OT, Grierson DS, Afolayan AJ. Effects of drying methods on the quality of Mentha longifolia L. subsp. capensis. Food Chem. 2007; 101: 995-98.
Ashafa AOT, Afolayan AJ. Screening the root extracts from Biden pilosa L. var. radiata (Asteraceae) for antimicrobial potentials. J Med Plants Res. 2009; 3: 568-72.
Ashafa AOT, Grierson DS, Afolayan AJ. Antimicrobial activity of extracts of Felicia muricata Thunb. J Biol Sci. 2008; 8: 1062- 66.
Bizimenyera ES, Githiori JB, Eloff JN, Swan GE. In vitro activity of Peltophorum africanum Sond. (Fabaceae) extracts on the egg hatching and larval development of the parasitic nematode Trichostrongylus colubriformis. Vet Parasitol. 2006; 142: 336-43.
Bogish BJ, Cheng TC. Intestinal nematodes. In: Human parasitology. Bogish BJ, Cheng TC (eds). New York, Saunder College Publisher, 1990, pp 269-91.
Cala AC, Chagas ACS, Oliveira MCS, Matos AP, Borges LMF, Sousa LAD, Souza FA, Oliveira GP. In vitro anthelmintic effect of Melia azedarach L. and Trichilia classenii C. against sheep gastrointestinal nematodes. Exper Parasitol. 2012; 130: 98-102.
Chauhan RS, Kaul MK, Shahi AK, Kumar A, Ram G, Tawa A. Chemical composition of essential oils in Mentha spicata L. accession [IIIM(J)26] from North-West Himalayan region, India. Ind Crops Prod. 2009; 29: 654–56.
Coles GC, Bauer C, Borgsteede F, Geerts S, Klei T R, Taylor MA, Waller PJ. World association for advancement in veterinary parasitology (WAAVP) methods for the detection of anthelmintic resistance in nematodes of veterinary importance. Vet Parasitol. 1992; 44: 35–43.
Diehl MS, Atindehou KK, Tere H, Betschart B. Prospect for anthelminthic plants in the Ivory Coast using ethnobotanical criteria. J Ethnopharmacol. 2004; 95: 277–84.
Eguale T, Tadese D, Giday M. In vitro anthelmintic activity of crude extracts of five medicinal plants against egg-hatch and larval development of Haemonchus contortus. J Ethnopharmacol. 2011; 137: 108-13.
Eguale T, Tilahun G, Debella A, Feleke A, Makonnen E. In vitro and in vivo anthelmintic activity of crude extracts of Coriandrum sativum against Haemonchus contortus. J Ethnopharmacol. 2007; 110: 428-33.
Ferhat M, Muhammad Q, Zaheer-Ud-Din K, Nameen I, Shahid M, Muhammad L, Phool S. In vitro evaluation anthelmintic activity of essential oils from different parts Skimma laureola (DC.) zucc.ex walp., ver. Nair. Pakistan J Bot. 2011; 43: 2915- 18.
Hendrix CM. Introduction to the nematodes. In: Diagnostic Veterinary Parasitology. Hendrix CM (ed). 2nd ed. Times Mirror Company, United State of America, Mosby Inc, 1998, pp 12, 108-16.
Hounzangbe-Adote MS, Paolini V, Fouraste I, Moutairou K, Hoste H. In vitro effects of four tropical plants on three life-cycle stages of the parasitic nematode, Haemonchus contortus. Res Vet Sci. 2005; 78: 155-60.
Hunter A. Animal health: General principles. Vol. 1. Netherlands, The tropical agriculturalist series, the Netherlands, Macmillan, 1996, p 167.
Kamaraj C, Rahuman AA. Efficacy of anthelmintic properties of medicinal plant extracts against Haemonchus contortus. Res Vet Sci. 2010; 10: 1-5.
Koduru S, Grierson DS, Afolayan AJ. Antimicrobial activity of Solanum aculeastrm (Solanaceae). Pharmacol Biol. 2006; 44: 284-86.
Kotze AC, Coleman GT, Mai A, McCarthy JS. Field evaluation of anthelmintic drug sensitivity using in vitro egg hatch and larval motility assays with Necator americanus recovered from human clinical isolates. Int J Parasitol. 2005; 35: 445–53.
Lewu FB, Grierson DS, Afolayan AJ. The leaves of Perlagonium sidoides may substitute for its roots in the treatment of bacterial infection. Biol Conservation. 2006; 128: 582-84.
Maphosa V, Masika PJ, Bizimenyera ES, Eloff JN. In vitro anthelmintic activity of crude aqueous extracts of Aloe ferox, Leonotis leonorus and Elephantorrhiza elephantine against Haemochus contortus. Trop Animal Health Prod. 2010; 42: 301-07.
Maphosa V, Masika PJ. Ethnoveterinary uses of medicinal plants: A survey of plants used in the ethnoveterinary control of gastrointestinal parasites of goats in the Eastern Cape Province, South Africa. Pharm Biol. 2009; 48: 697-702.
McGaw LJ, Rabe T, Sparg SG, Jager AK, Eloff JN, van Staden J. An investigation on the biological activity of Combretum species. J Ethnopharmacol. 2000; 75: 45-50.
McGaw LJ, van Der Merwe D, Eloff JN. In vitro anthelmintic, antibacterial and cytotoxic effects of extracts from plants in South Africa ethnoveterinary medicine. Vet J. 2007; 173: 366-72.
Molan EL, Meagher LP, Spencer PA. Effects of flavan-3-ols on the in vitro egg hatching, larval development and viability of infective larvae of Trichostrongylus colubriformis. Int J Parasitol. 2003; 33: 1691-98.
Nibret E, Wink M. Volatile components off our Ethiopian Artemisia species extracts and their in vitro antitrypanosomal and cytotoxicactivities. Phytomedicine 2010; 17: 369–74.
Pessoa LM, Morais SM, Bevilaqua, CML Luciano JHS. Anthelmintic activity of essential oil of Ocimum gratissimum Linn. And eugenol against Haemonchus contortus. Vet Parasitol. 2002; 109: 59-63.
Rao BR, Kaul PN, Syamasundar KV, Ramesh S. Water soluble fractions of rose-scented geranium (Pelargonium species) essential oil. Bioresource Technol. 2002; 84: 243–46.
Reinecke RK. Veterinary helminthology. Butterworths, Durban, 1983, pp 325-26.
Salih NE. A brief review on the development of strongylid nematode eggs and larvae under constant and changing temperature condition I. egg development. J Thermal Biol. 2003; 6: 287-95.
Soulby EJL. Helminths, arthropods and protozoa of domesticated animals. In: Transactions of the royal society of tropical medicine and hygiene. Longstaffe JA (ed). London, Bailliere Tindall, 1982, p 809.
Suteky T, Dwatmadji T. Anthelmintic activity of Melastoma malabatricum extract of Haemonchus contortus in vitro. Asian J Pharm Clin Res. 2011; 4: 68-70.
Tembely S. Development and survival of infective larvae of nematode parasites of sheep on pasture in a cool tropical environment. Vet Parasitol. 1998; 79: 81-87.
Tsotetsi AM, Mbati PA. Parasitic helminths of veterinary importance in cattle, sheep and goats on communal farms in the northeastern Free State, South Africa. J South African Vet Assoc. 2003; 74: 45-48.
Zafar I, Muhammad L, Abdul J, Muhammad NG, Anwarul HG. In vitro and in vivo anthelmintic activity of Nicotiana tabacum L. leaves against gastrointestinal nematodes of sheep. Phytother Res. 2006; 20: 46-48.
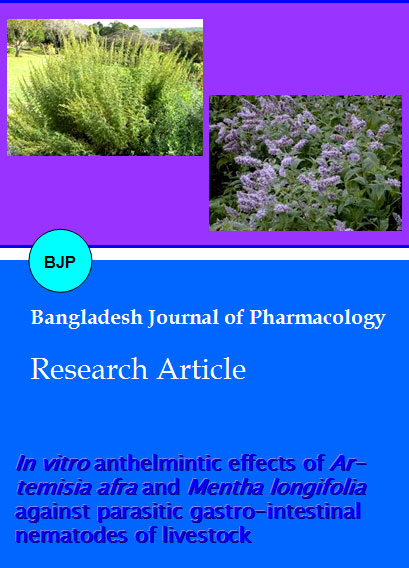