Trifluoromethyl-phenyl-triazolyl derivative of beta-bisabolol induces cell death in ME-180 cervical cancer cells through induction of apoptosis and ROS generation
Abstract
The aim of the current investigation was to design, synthesize and demonstrate the anti-cancer and apoptotic activity of trifluoromethyl-phenyl-triazolyl derivative of beta-bisabolol (TTB) in ME-180 human cervical cancer cells. MTT and clonogenic assays were used to evaluate the cell viability and colony formation tendencies of the cells respectively. Phase contrast and fluorescence microscopic investigations were used to evaluate the effect of TTB on cellular morphology and apoptosis. Flow cytometric analysis using fluorescent CM-DCFH2-DA were used to study the effect of TTB on reactive oxygen species (ROS) formation. The results revealed that TTB significantly inhibited the proliferation of ME-180 human cervical cancer cells in a time-dependent as well as dose-dependent manner. TTB has the capacity to inhibit both anchorage dependent as well as anchorage independent growth of ME-180 cervical cancer cells. TTB-treated cells revealed chromatin condensation, fragmented nuclei and nuclear shrinkage. A 3-fold increase of ROS production was seen after 72 uM TTB treatment.
Introduction
Apoptosis, also known as programmed cell death, occurs through activation of several signalling pathways. Many of the anti-cancer drugs induce cell death though apoptosis pathway (Brown and Attardi, 2005; Kerr et al., 1972; Wyllie et al., 1980). Apoptosis activation results after a complex interaction of several molecular pathways. The process is characterized by DNA fragmentation, fragmentation of a cell into several small apoptotic bodies. Cytoplasmic and nuclear chromatin condensation eventually followed by the annihilation and removal of the dying cells through the process of phagocytosis. Apoptosis is believed to be the principal method of cell death in numerous physiological events (Hockenbery et al., 1990; Gross et al., 1999). Cervical cancer is a common cancer in women and has been found to be associated with human papillomavirus infection. Human papillomavirus (HPV) infection plays a vital part in the development of more than 80% of cases. It is the second most common form of cancer targeting women and majority of these cases (70-80%) are found in developing nations (Parkin et al., 2005; Bosch et al., 1995). Different treatment options are currently available for cervical cancer including surgery, radiotherapy, chemotherapy or a combination of these three. Each of these treatment strategies has eminent serious adverse effects coupled with the problem of frequent recurrence. One-third of patients will grow advanced or recurrent cancers, the pelvis being the most common site of failure (Dornhofer and Hockel, 2008; Eskander and Tewari, 2014).
Chemotherapy treatment is based on cytotoxicity of compounds and as such always has deleterious adverse effects. As a result, there is a pressing need of novel anticancer agents with minimal adverse effects. Presently, attention is being given to non-toxic anticancer agents from natural sources especially plant derived natural compounds for the treatment of different tumors including cervical cancer. Majority of the anti-cancer drugs such as taxol, vincristine, vinblastine, etoposide, camptothecin are plant-based natural drugs. These drugs have been reported to exert their anti-cancer effect through a variety of mechanisms including apoptosis induction, cell cycle arrest, altered carcinogen metabolism, immune activation etc (Corrie and Pippa, 2008; Skeel, 2003; Siddik, 2005). The objective of the present research work was to evaluate the anticancer and apoptotic effects of trifluoromethyl-triazolyl derivative of beta-bisabolol (TTB) in ME-180 cervical cancer cells.
Materials and Methods
Chemicals and reagents
TTB was dissolved in DMSO (Merck, Germany) at a stock solution of 100 mM and stored at -20ºC. DMEM, RPMI 1640 medium, PI, Triton X-100, 3-(4,5-dimethyl-thiazol-2-yl)-2,5-diphenyltetrazolium bromide (MTT), penicillin/streptomycin solution and Hoechst 33342 were obtained from Sigma Chemical Co. (USA). Fetal bovine serum was obtained from Gibco BRL (USA). All other chemicals and solvents used were of the highest purity grade.
Synthesis of TTB
To a solution of beta-bisabolol (1) (100 mg) in THF was added cesium carbonate (165 mg) and propargyl bromide (175 mg) and the reaction mixture was stirred at room temperature for about 4 hours. Reaction was monitored by thin layer chromatography (ethyl acetate : n-hexane, 2:8 v/v) and the crude product was subjected to column chromatography to give pure compound (2) (65 mg). Afterwards, to a solution of compound 2 (50 mg) in tertiary butanol-water (2:1, 5 mL) was added sodium ascorbate (2.5 mg) and copper sulfate (1.3 mg). To this mixture, trifluorophenyl azide (2 equivalents) was added and the reaction mixture was sonicated at 40ºC till its completion and monitored by TLC. After completion, the reaction mixture was diluted with water and extracted with ethyl acetate, then organic layer was dried over sodium sulfate and purified through column chromatography to give compound 3 (25 mg).
Cell lines and cell culture
ME-180 human cervical cancer cells were obtained from Shanghai Institute of Cell Resource Center of Life Science (Shanghai, China). Cells were grown in Dulbecco's Modified Eagle's Medium (DMEM) supplemented with 10% fetal bovine serum and 100 U/mL penicillin and 100 ug/mL streptomycin. Cells were cultured in CO2 incubator (New Brunswick, Galaxy 170R, eppendroff) with an internal atmosphere of 95% air and 5% CO2 gas and the cell lines were maintained at 37ºC.
MTT assay for cell proliferation
The effects of TTB on ME-180 cell viability were studied by MTT assay. Cells (2 x 106 cells/well in 100 μL medium) were seeded into 96-well plates for 24 hours before drug treatment. Subsequent treatment with numerous doses of TTB (0, 3, 6, 9, 18, 36 and 72 uM) for 48 hours, the cell plates were treated with MTT solution (10 uL; 5 mg/mL in phosphate-buffered solution for an additional 4 hours at 37ºC. The formazan crystals in viable cells were solubilized with DMSO (150 uL) and the absorbance was measured on a microplate reader (ELX 800; Bio-tek Instruments, Inc., USA) at a wavelength of 490 nm.
Cancer cell colony inhibition assay
The colony formation by cancer cells in soft agar is an excellent and sensitive test to evaluate cell viability and cytotoxic potential. Cells were suspended in 1 mL of DMEM containing 0.5% agarose (Amresco, USA) and 10% fetal bovine serum, and plated on a bottom layer containing 0.5% agarose and 5% fetal bovine serum in 6-well plate in triplicate. After 2 week, plates were stained with 0.5% gentian violet and the number of colonies was counted under light microscope (Nomura et al., 1990).
Morphological study of cervical cancer cells by phase contrast microscopy
ME-180 cervical cancer cells were seeded into 6-well plates at a density of 2 x 106 cells/well in 10 mL medium. The cells were treated with varying concentrations (0, 9, 36 and 72 µM) of triazolyl TTB for 48 hours. The morphological alterations were witnessed and the images were captured under an inverted light microscope (Olympus, USA) after 48 hours. The same spot of cells was noticed and captured. The images were captured at a magnification of x200.
Morphological and apoptotic study of cervical cancer cells by fluorescence microscopy
ME-180 cervical cancer cells were seeded into 12-well plates at a density of 2 x 105 cells/well. Following treatment with 0, 9, 36 and 72 µM dose of TTB for 48 hours, cell apoptosis was evaluated by the Hoechst staining kit as per instructions of the manufacturer. After drug treatment, the cells were fixed with 5% polyoxymethylene and then incubated in Hoechst solution for 10-15 min in the dark. The staining images were recorded using a UV fluorescence microscope (Olympus, Olympus Optical Co., Ltd, Japan) using UV filter at x200 magnification to detect morphological evidence of apoptosis.
Measurement of intracellular ROS generation
Intracellular ROS generation was evaluated using fluorescent CM-DCFH2-DA. ME-180 cells were seeded in 6-well plates and after adhesion the cells were pretreated with 20 µM CM-DCFH2-DA for 30 min followed by coincubation with various concentrations of TTB for another 3 hours and washed with ice-cold phosphate buffer solution twice. The cells were collected and analyzed using a flow cytometry (Becton Dickinson FACS CantoTM, USA) with wavelength of excitation and emission at 488 nm and 525 nm respectively.
Statistical analysis
All data were derived from at least three independent experiments. The results were expressed as the mean ± SD. Differences between groups were analyzed using the Student's t-test. p<0.05 was considered statistically significant.
Results
Time-dependent and dose-dependent antiproliferative effects of TTB in ME-180 cervical cancer cells
The MTT assay was used for testing the growth inhibitory effects of TTB on ME-180 cervical cancer cells. The synthetic route to TTB from beta-bisabolol is depicted in Figure 1. Results indicated that TTB considerably inhibited the proliferation of ME-180 (Figure 2) human cervical cancer cell line. Furthermore, time- and concentration-dependent inhibition curves were observed in these cells. The calculated IC50 values at 24 and 48 hours were 17.2 and 13.1 uM respectively. In addition, the colony formation assay revealed that TTB inhibits the colony formation tendency of ME-180 cells in a dose dependent manner (Figure 3 and 4). So, TTB has the capacity to inhibit both anchorage dependent as well as anchorage independent growth of ME-180 cervical cancer cells.
Figure 1: Chemical synthesis of trifluoromethyl-phenyl triazolyl derivative of beta-bisabolol
Figure 2: Dose-dependent and time-dependent cytotoxic effect of triazolyl derivative of beta-bisabolol in human cervical cancer cells (ME-180). Data are shown as the mean ± SD of three independent experiments. *p<0.05, **p< 0.01, vs 0 µM (control)
Figure 3: Inhibitory effect of triazolyl derivative of beta-bisabolol on the growth and multiplication of human cervical cancer cells (ME-180). The colony formation assay of ME-180 cells treated with TTB at indicated doses
Figure 4: Effect of triazolyl derivative of beta-bisabolol on colony formation potential in ME-180 cervical cancer cells. The cells were treated with 0 µM (A), 9 µM (B), 36 µM (C) and 72 µM (D) dose of TTB respectively
Effect of TTB on the cellular morphology of ME-180 cells as detected by phase contrast and fluorescence microscopies
Exposure of ME-180 cells to 0, 9, 36 or 72 uM of TTB for 48 hours resulted in a substantial decrease in cell count and, furthermore, induced morphological changes that were characteristic of cytotoxicity in ME-180 cells under phase-contrasted microscopy following exposure to TTB (Figure 5A-D). The morphological changes induced by TTB included the detachment of the cells from substratum, cell shrinkage etc. The untreated control cells were uniformly distributed on the substratum. Decrease in the cell population was seen with the increase in the TTB concentration.
Figure 5: Triazolyl derivative of beta-bisabolol induced morphological changes in ME-180 cervical cancer cells as identified by phase contrast microscopy (magnification 200X). Rounded and contracted cells were observed in TTB-treated cells. A, represents control (untreated cells), B, C and D represent effect of 9, 36 and 72 µM of triazolyl derivative of beta-bisabolol on cell morphology of ME-180 cells
Further, apoptotic morphological changes in ME-180 cells were detected by fluorescence microscopy using Hoechst 33258. Following the treatment with different doses of TTB for 48 hours, the cells were investigated by fluorescence microscope. TTB-treated cells stained with Hoechst 33258 revealed chromatin condensation, fragmented nuclei and nuclear shrinkage which increased with the increasing dose of TTB (Figure 6A-D). The number of apoptotic cells increased after treatment with TTB for 48 hours.
Figure 6: Fluorescence microscopy images of ME-180 cervical cancer cells after treatment with 0 (A), 9 (B), 36 (C) and 72 (D) µM dose of triazolyl derivative of beta-bisabolol for 48 hours. The cells were stained with Hoechst 33258. Note the chromatin condensation, fragmented nuclei and nuclear shrinkage (white arrows) which increased with the increasing dose of TTB
TTB induced ROS formation in ME-180 cervical cancer cells
The effect of TTB on intracellular ROS production was measured by flow cytometry with a fluorescent probe CM-DCFH2-DA. As shown in Figure 7, after treating ME-180 cells with TTB for 2 hours, it profoundly induced ROS formation. A dose dependent ROS generation was witnessed and 3-fold of increase of ROS production was seen after 72 uM TTB treatment.
Figure 7: TTB-induced ROS generation in ME-180 cells. ME-180 cells were treated with TTB (0, 9, 36 and 72 mM) for 3 hours, and the ROS generation was evaluated using CM-DCFH2-DA staining by flow cytometry. **p<0.01; *p<0.05 vs. control group
Discussion
The findings of the current study reveal that TTB induced time-dependent as well as dose-dependent antiproliferative effects in ME-180 cervical cancer cells. The IC50 values of 17.2 and 13.1 uM indicate that TTB is a potential cytotoxic agent. In addition, TTB also inhibited ME-180 cervical cancer cell colony formation capability in a dose-dependent manner. Further, in order to reveal the antiproliferative mode of action of TTB, its effect on cellular morphology was demonstrated using phase contrast microscopy as well as fluorescence microscopy using Hoechst 33258 as a staining agent. Exposure of ME-180 cells to 0, 9, 36 or 72 uM of TTB for 48 hours resulted in a substantial decrease in cell count. After treatment the cells got detached from the substratum and cell shrinkage was observed. On the other hand, the untreated control cells showed normal morphology and uniform distribution. Fluorescence microscopy indicated that TTB-treated cells stained with Hoechst 33258 revealed chromatin condensation, fragmented nuclei and nuclear shrinkage which increased with the increasing dose of TTB. Further, the effect of TTB on ROS production in ME-180 cells was evaluated using flow cytometry with a fluorescent probe CM-DCFH2-DA.After treating ME-180 cells with TTB for 2 hours, it profoundly induced ROS formation and it was seen that there was 3-fold increase in ROS production after 72 µM TTB treatment. The process of apoptosis is a well-organized process which helps in eliminating unnecessary and injured cells from the body. A disruption of this process leads to various disorders including cancer. The process of apoptosis can take any one of the two routes viz., extrinsic or intrinsic routes (Adams and Cory, 2007; Cory and Adams, 2002). beta-Bisabolol is a naturally occurring monocyclic sesquiterpene alcohol. The compound is the main constituent of various essential oils. beta-bisabolol is found in Zea mays L. plant and is a major constituent of cotton. A structurally similar compound alpha-bisabolol has been reported to induce apoptosis in different leukemia models (Cavalieri et al., 2011; Thompson et al., 1974; Dickens, 1986).
Conclusion
The findings of this study indicate for the first time that TTB exhibits antiproliferative activity in ME-180 cervical cancer cells through the process of apoptosis and generation of reactive oxygen species.
References
Adams J, Cory S. The Bcl-2 apoptotic switch in cancer development and therapy. Oncogene 2007; 26: 1324-37.
Bosch FX, Manos MM, Munoz N, Sherman M, Jansen AM, Peto J, Schiffman MH, Moreno V, Kurman R, Shah KV.
Prevalence of human papillomavirus in cervical cancer: A worldwide perspective. J Natl Cancer Inst. 1995; 87: 796-802.
Brown JM, Attardi LD. The role of apoptosis in cancer development and treatment response. Nat Rev Cancer. 2005; 5: 231-37.
Cavalieri E, Rigo A, Bonifacio M, Carcereri de Prati A, Guardalben E, Bergamini C, Fato R, Pizzolo G, Suzuki H, Vinante F. Proapoptotic activity of alpha-bisabolol in preclinical models of primary human acute leukemia cells. J Transl Med. 2011; 9: 45.
Corrie PG, Pippa G. Cytotoxic chemotherapy: Clinical aspects. Medicine 2008; 36: 24-28.
Cory S, Adams J. The Bcl-2 family: Regulators of the cellular life-of-death switch. Nat Rev Cancer. 2002; 2: 647-56.
Dickens JC. Orientation of boll weevil, Anthonomus grandis Boh., (Coleoptera: Curculionidae), to pheromone and volatile host compound in the laboratory. J Chem Ecol. 1986; 12: 91-98.
Dornhofer N, Hockel M. New developments in the surgical therapy of cervical carcinoma. Ann N Y Acad Sci. 2008; 1138: 233-52.
Eskander RN, Tewari KS. Chemotherapy in the treatment of metastatic, persistent, and re current cervical cancer. Curr Opin Obstet Gynecol. 2014; 26: 314-21.
Gross A, McDonnell JM, Korsmeyer SJ. BCL-2 family members and the mitochondria in apoptosis. Genes Dev. 1999; 13: 1899-911.
Hockenbery D, Nuñez G, Milliman C, Schreiber RD, Korsmeyer SJ. Bcl-2 is an inner mitochondrial membrane protein that blocks programmed cell death. Nature 1990; 348: 334–36.
Kerr JF, Wyllie AH, Currie AR. Apoptosis: A basic biological phenomenon with wide-ranging implications in tissue kinetics. Br J Cancer. 1972; 26: 239-57.
Nomura Y, Tashiro H, Hisamatsu K. Differential effects of estrogen and antiestrogen on in vitro clonogenic growth of human breast cancers in soft agar. J Natl Cancer Inst. 1990; 82: 1146-49.
Parkin DM, Bray F, Ferlay J, Pisani P. Global cancer statistics, 2002. CA Cancer J Clin. 2005; 55: 74-108.
Siddik ZH. Mechanisms of action of cancer chemotherapeutic agents: DNA-interactive alkylating agents and antitumour platinum-based drugs. The cancer handbook. John Wiley and Sons, Ltd. 2005.
Skeel RT. Handbook of cancer chemotherapy. 6th ed. Philadelphia, Lippincott Williams and Wilkins. 2003. pp 3-25.
Thompson AC, Hedin PA, Gueldner RC, Davis FM. Corn bud essential oil. Phytochemistry 1974; 13: 2029-32.
Wyllie AH, Kerr JF, Currie AR. Cell death: The significance of apoptosis. Int Rev Cytol. 1980; 68: 251-306.
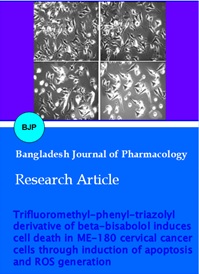