Combination of axitinib and dasatinib for anti-cancer activities in two prostate cancer cell lines
Abstract
Prostate cancer is the major cause of cancer-related deaths worldwide in men. There are new treatment methods and drugs are being developed with promising results in two of the prostate cancer cell lines (PPC-1 and TSU-Pr1). These two cells were treated with 20 uM of axitinib combined with dasatinib for 6-72 hours. The cell viability assessed by the cytotoxicity assay. Various regulatory genes such as c-KIT, cell cycle and apoptosis and angiogenic factors were also studied. The enzyme activity of apoptosis effector caspase-3 was colorimetrically determined. Axitinib and dasatinib combination lowered the survival rate of PPC-1 cells but enhanced the survival rate of TSU-Pr1 cells. The protein expression levels in apoptosis and angiogenesis factors were also found to be in contrast between the two cell lines. PPC-1 and TSU-Pr1 cells displayed a different response to axitinib with dasatinib, which explains different expression levels of regulators of cell-cycle, apoptosis and angiogenesis.
Introduction
Prostate cancer is one of the major types of cancer especially among the aging men in the United States (Greenlee et al., 2001). Androgen receptor signaling is essential in cancer progression and also for normal prostate differentiation (Gelmann et al., 2002). But prostate cancer has a tendency to become refractory to hormonal therapy. Therapy involving the withdrawal of androgen remains the most efficient way of treating advanced prostate. All the deaths related to prostate cancer are mainly due to the progressive, hormone refractory prostate cancer.
Several new drugs and combinations of drugs have shown good results against hormone-refractory prostate cancer. Axitinib (AG-013736) is a multi-target tyrosine kinase inhibitor undergoing clinical trials along with other chemotherapeutic drugs (Spano et al., 2008) and found to be an efficient anti-angiogenic drug administered orally and targeting the vascular endothelial growth factor receptor-2) tyrosine kinase domain (Kelly et al., 2009; Solowiej et al., 2009).
Dasatinib is a broad spectrum multi-kinase inhibitor that exhibits little efficacy in cancer patients (Colombel et al., 1993; Pienta et al., 2005). Dasatinib inhibited the action of SRC, a non-receptor tyrosine kinase and other tyrosine kinases involved in phosphorylation of the androgen receptor in prostate tissue and cell lines. These results show that combination therapy with axitinib and dasatinib can beneficially act as drugs on two different stages of the disease.
We investigated the cytotoxic effect of combination therapy in this study with axitinib and dasatinib in two hormone refractory prostate cancer cells and the activity of apoptotic and proliferative regulators were analyzed.
Materials and Methods
Materials
RPMI 1640 medium and fetal bovine serum were obtained from GIBCO, Life Technologies (USA). Chemical compounds were obtained from Sigma-Aldrich (USA). Axitinib was obtained from Pfizer (tradename: Inlyta) and dasatinib was purchased from LC Laboratories.
Cell lines
Hormone refractory prostate cancer cell lines, PPC-1 and TSU-Pr1 were obtained from American Type Culture Collection (USA). Both PPC-1 and TSU-Pr1 cell lines are insensitive to androgen and known models of human prostate cancer at advanced stages. TSU-Prq produces low amount of prostatic acid and phosphatase and they don’t have any nuclear androgen receptors.
Cell culture and antibiotic treatment
PPC-1 and TSU-Pr1 cells were cultured in RPMI 1640 medium supplemented with 10% heat-inactivated fetal bovine serum, penicillin and streptomycin at 100 units/mL and 100 mg/mL respectively. Cultures were maintained in a 37°C incubator with 5% CO2. To treat the cells with the drug, cells are grown up to 60% confluence. After 24 hours, the cells were treated with 20 mM of axitinib and dasatinib for 6-72 hours. After treatment, trypsin was used to detach the cells and collected for posterior analysis.
Cell viability assay
Cells (5,000 cells/well) were grown in 24 well plates (Corning, USA) and cell viability was determined by viability/cytotoxicity kit for mammalian cells (Life Technologies, USA) at 6, 12, 24, 48 and 72 hours after treatment with axitinib and dasatinib. The viability of cells was calculated by normalizing the absorbance to the control and results were represented as the percentage of the control.
Total RNA preparation and real-time PCR
Total RNA was isolated from PPC-2 and TSU-Pr1 cells using TRIzol reagent (Life Technologies, USA) as per the manufacturer’s instruction. The total RNA was assessed quantitatively at 260 and 280 nm in NanoVue Plus spectrophotometer (GE Healthcare Life Sciences, USA) and qualitatively by agarose gel electrophoresis. 1 mg of total RNA was reverse-transcribed as previously described (Vaz et al., 2012) in a final volume 20 mL. The expression of p21 and VEGF in PPC-1 and TSUPr1 cells in response to axitinib and dasatinib treatment were studied by 1 mL of cDNA. Specific primers for p21 (50–30, Sense: TCCAGCGACCTTC CTCATC; antisense: AGCCTCTACTGCCACCATC), VEGF (5030, Sense: CGAAACCATGAACTTTCTGC; antisense: CCTCAGTGGGCACACACTCC), b-2-microglobulin (50–30, Sense: ATGAGTATGCCTGCCG TGTG; antisense: CAAACCTCCATGATGCTGCTT AC), GAPDH (50-30, sense: CGCCAGCCGAGCCA CATC; antisense: CGCCCAATACGACC AAATCCG), and 18S (50–30, Sense: AAGACGAACCAGAGCGAA AG; antisense: GGCGGGTCATGGGAATAA) were used. To normalize gene expression, the b-2-microglobulin, GADPH, or 18S were used as internal controls. qPCRs were carried out in an MiniOpticon Real-Time PCR system (Bio-Rad, USA) and efficiency of the amplification was determined for all primer sets using several dilutions of PPC-1 and TSU-Pr1 cells cDNA (1; 1:5; 1:25). Primer concentration and annealing temperature for each primer set were optimized and the specificity of the amplicons was determined by melting curve analysis. qPCR reactions were carried out with Takara Bio SYBR Premix Ex Taq (Takara, Japan) and 300 nM (p21 and VEGF) or 200 nM (housekeeping genes) of sense and antisense primers for each gene. Annealing temperature was 60°C for all primer sets. Samples were run in triplicate in each qPCR assay.
Protein extraction
RIPA buffer was used to isolate total proteins from PPC-1 and TSU-Pr1 hormone refractory prostate cancer cells containing 150 mM NaCl, 1% Nonidet-P40 substitute, 0.5% Na-deoxycholate 0.1% SDS, 50 mM Tris pH 8.0 and 1 mM EDTA supplemented with 1% protease inhibitor cocktail and 10% PMSF (Sigma-Aldrich). The cells harvested were homogenized with RIPA buffer and kept on ice for 20 min with occasional mixing and centrifuged at 14,000 rpm for 20 min at 4°C. Total proteins were separated and quantified by Bradford assay (Bio-Rad).
Western blot
50 mg of protein extracts were separated on 10% gel SS-PAGE. Proteins were then transferred to a PVDF membrane and incubate with rabbit anti-c-KIT (1:1000, Cell Signaling Technology), rabbit anti-Bax (1:500, CST), rabbit anti-Bcl-2 (1:1000 CST) or rabbit anti-p53 (1:1000, CST) as described previously (Correria et al., 2014a, Correia et al., 2014b). Anti-tubulin monoclonal antibody from mouse (1:5000, Sigma-Aldrich) was used as protein loading control in all WB analyses. Goat anti-rabbit IgG-AP (1:5000, Sigma-Aldrich) or goat anti-mouse IgG. IgM-AP (1:5000, Invitrogen) used as secondary antibodies. Membranes were incubated with chemiluminscent substrate for 5 min and scanned with Image Quant LAS4000 (GE Life Sciences). The density of the bands was estimated by standard methods and normalized by division with the density of control protein, a-tubulin bands.
Capase-3 activity assay
Caspase-3 activity was colorimetrically determined at 405 nm by detecting the yellow product, p-nitroaniline (pNA). 50 mg of total protein extract was incubated overnight at 37°C with 25 mM HEPES, 0.1% 3CHAPS, 10% sucrose and 10 mM DTT, pH 7.5 and 200 mM of caspase-3 substrate (Ac-DEVD-pNA).
Fluorescent immunocytochemistry
PPC-1 and TSU-Pr1 cells were fixed with 4% paraformaldehyde for 10 min and permeabilized with 0.1% Triton X-100 for 5 min. Then, a blocking step was performed by incubating cells with 20% fetal bovine serum in PBST for 1 hour at room temperature. Cells were incubated with anti-C-KIT antibody (CST) for 1 hour at room temperature. The Abberior Star 488-conjugated goat anti-rabbit IgG (Abberior) was used as secondary antibody. Primary antibody was omitted to increase the specificity of the staining. The nuclei of the cells were stained with Hoechst 33342 for 10 min. Lamelle were washed and mounted onto slides with Dako fluorescent mounting medium. Images were acquired using a Zeiss LSM710 last scanning confocal microscope (Carl Zeiss, Germany)
Results
Effect of axitinib and dasatinib on viability of PPC-1 and TSU-Pr1 cells
The effect of axitinib and dasatinib combination on the viability of PPC-1 and TSU-Pr1 cells was determined by the MTT assay. 48 and 72 hours after administration of 20 mM of the equimolar concentration of axitinib and dasatinib, the viability of PPC-1 cells significantly decreased to 88 and 82% respectively (Figure 1). Although the viability of TSU-Pr1 cells markedly decreased upon 6 hours of treatment (36% reduction vs control), thereafter, this effect was reversed (Figure 1)
Figure 1: Number of PPC-1 and TSU-Pr1 viable cells after treatment with axitinib and dasatinib (20 mM) for 6 to 72 hours. Results are expressed as % of control (dashed line). Error bars indicate mean ± S.E.M (n>5)
Axitinib and dasatinib induced apoptosis in PPC-1 and TSU-Pr1 cells
The apoptotic induction process was used as an approach for the treatment of various human cancers and process was caused by two different pathways, receptor-mediated and mitochondrial (Connor et al., 2006, McKenzie et al., 2006, Hensley et al., 2013). Apart from this activated pathway, caspase enzymes triggered apoptosis and both pathways came together at the initiation of caspase-3 and considered as a significant end point of apoptosis (Fiandalo et al., 2012). Therefore, the activity of caspase-3 was used to measure apoptosis triggered by the addition of axitinib and dasatinib in HRPC cells. In PC-1 cells, caspase-3 had increased significantly in response to drug treatment for 72 hours (20% relative to control, Figure 2). In TSU-Pr1 cells caspase-3 activity was decreased at both 48 hours and 72 hours after drug administration (59% and 62% reduction relative to control, Figure 2). As axitinib and dasatinib affected the viability and apoptosis of PPC-1 and TSU-Pr1 at 72 hours after treatment.
Figure 2: Caspase-3 activity in PPC-1 and TSU-Pr1 cells after axitinib and dasatinib treatment (20 mM) for 48 and 72 hours. Results are expressed as % of control (dashed line)
Caspase-8 interacted with death receptors and activated the down-stream effectors of apoptosis namely caspase-3 (Stupack et al., 2013). In PPC-1 treated cells, the expression of caspase-8 was significantly increased relative to control (Figure 3A). No significant differences were observed in caspase-8 expression in TSU-Pr1 cells treated with the drug (Figure 3B). Bax and Bcl-2 proteins regulated apoptosis and they were proapoptotic and antiapoptotic members of the protein family (Youe et al., 2008). The permeabilization of mitochondrial pores and release of cytochrome c were by Bax protein whereas Bcl-2 was known to repair this process (Jurgensmeier et al., 1998). Treatment with 20 mM of axitinib and dasatinib for 72 hours decreased the expression of antiapoptotic and axitinib decreased the Bcl-2 expression in PPC-1 cells but no effect was observed in TSU-Pr1 cells (Figure 4A and Figure 4B). PPC-1 cells were native for Bax and TSU-Pr1 cells did not show the difference on the expression of this proapoptotic protein in response to the drug (Figure 4C and 4D). Cytochrome c interacts with the apoptotic protease activating factor-1 (Aparf-1) once it is released to the cytosol and activates caspase-9 which in turn activates caspase-3 (Srinivasula et al., 1998). Western Blot analysis showed that the protein levels of caspase-9 increased in treated PPC-1cells (Figure. 3C). The expression of caspase-9 protein in treated TSU-Pr1 cells was not much different from the control (Figure 3D). The p53 (tumor suppressor protein) plays an important role in the regulation of the Bcl-2 family members and regulates the expression of cyclin dependant kinase inhibitor, p21 which induces cell cycle arrest (Waldman et al., 1995). TSU-Pr1 cells were negative for p53 and no differences were observed on the protein levels p53 in PPC-1 cells in response to axitinib and dasatinib (Figure 4E and 4F).
Figure 3: Effect of axitinib and dasatinib (20 mM) on protein levels of caspase-8 (A) and caspase-9 (C) in PPC-1 and TSU-Pr1cells determined by Western Blot analysis. Representative immunoblots for caspase-8 and -9 are shown, respectively, in panels B and D. Results are expressed as fold-variation relatively to control (dashed line) after normalization with a-tubulin
Figure 4: Effect of axitinib and dasatinib (20 mM) on protein levels of Bcl-2 (A, B), Bax (C, D), and p53 (E, F) in PPC-1 and TSU-Pr1 cells determined by Western Blot analysis. Results are expressed as fold-variation relatively to control (dashed line) after normalization with a-tubulin
VEGF and p21 expression in axitinib and dasatinib treated PPC-1 and TSU-Pr1 cells
The expression of p21 and VEGF was studied by real-time PCR analysis in PPC-1 and TSU-Pr1 cells after treatment with axitinib and dasatinib for 72 hours. The combined drug treatment significantly increased the p21 mRNA expression in both PPC-1 and TSU-Pr1 cells (Figure 5A). The VEGF is highly expressed in prostate cancer cells and it is a well-known stimulator of angiogenesis, and contributes to the distribution of prostate tumor cells to the skeleton or other tissues (Roberts et al., 2013). The mRNA expression of VEGF was slightly lowered in PPC-1 cells after treatment whereas the treated TSU-Pr1 cells displayed a strong increase in the mRNA levels of VEGF (Figure 5B).
Figure 5: Effect of axitinib and dasatinib on mRNA levels of p21 (A) and VEGF (B) in PPC-1 and TSU-Pr1 cells determined by real-time PCR. Results are expressed as fold-variation relatively to control (dashed line) after normalization with b-2-microglobulin, GAPDH and/or 18S housekeeping genes
Differential expression of full-length and truncated isoforms of c-KIT
The onset and progression of PCa correlated with c-KIT receptor (Paronetto et al., 2004) however, the expression of c-KIT isoforms in PCa cells is still unknown and needs further exploration. Therefore, the full-length c-KIT and its small isoforms expression were studied in PPC-1 and TSU-Pr1 cells. Western blot analysis showed that TSU-Pr1 cells had reduced levels of the full-length c-KIT (145 kDa) relatively to PPC-1 (Figure 6A). But the expression of 50 and 30 kDa c-KIT isoforms were higher in TSU-Prl cells when compared with PPC-1 (Figure 6A and 6B). The immunofluorescent labeling of c-KIT confirmed the expression of c-KIT in both PPC-1 and TSU-Pr1 cells (Figure 6C)
Figure 6: Expression of full-length (145 kDa), and 50 and 30 kDa isoforms of c-KIT in PPC-1 and TSU-Pr1 cells. (A) Expression of c-KIT proteins determined by WB analysis. Representative immunoblots are shown in panel B. (C) Representative confocal microscopy images showing c-KIT positive staining in PPC-1 and TSU-Pr1 cells. Nuclei are stained with Hoechst 33342 (blue, i and iv), and fluorescence c-KIT-positive staining is green (ii and v). Merged images are shown in iii and vi
Discussion
We investigated the cytotoxic effects of axitinib and dasatinib in PPC-1 and TSU-Pr1 cells which do not express the AR (Yu et al., 2009). Axitinib and dasatinib decreased the viability of PPC-1 cells after 24 hours but the TSU-Pr1 cells showed significant increase in cell viability from 12 hours and thereafter. So for there are no reports about this contradictory effect axitinib and dasatinib induces the viability of cancer cells. Kubler et al., (2005) showed that combination of imatinib and docetaxel produced antagonistic effects in DU145 and PC3. These results showed that difference in response to drugs by PPC-1 and TSU-Pr1 cells was due to distinct protein expression pattern related to apoptosis.
Caspase enzymes involved in apoptosis are regulated by external or internal factors or by cell membrane receptors (Hensely et al., 2913). In PPC-1 cells, the combination of axitinib and dasatinib treatment showed 20% more casapase-3 activity which induced apoptosis. Caspase-3 activity was drastically reduced (62%) in TSU-Pr1 cells which corresponds to the increased cell viability. Caspase-8 and caspase-9 are involved in caspase-3 activation during apoptosis. Axitinib and dasatinib treatment showed improvement in caspase-8 and caspase-9 protein expression in PPC-1 cells confirming the activation of intrinsic and extrinsic pathways of apoptosis whereas no difference was observed in caspase-8 and caspase-9 expression in TSU-Pr1 cells. Caspase-3 was reduced upon administration of axitinib and dasatinib. The proapoptotic and antiapoptotic proteins, Bax/Bcl-2 from Bcl-2 family were used as an indicator of apoptosis. Axitinib and dasatinib treatment reduced the Bcl-2 expression in PPC-1 cells, with increased rate of apoptosis and TSU-Pr1 cells showed no difference after administration of axitinib and dasatinib. Regulating the Bcl-2 expression is a strategy for PCa treatment and we showed the effect of axitinib and dasatinib on the modulation of apoptotic machinery in PCa cells.
The mRNA expression of VEGF was decreased in PPC-1 cells which were sensitive to axitinib and dasatinib, whereas in TSU-Pr1 cells increased expression of VEGF was observed. Although the underlying molecular mechanisms are unknown, this study described the modulation of VEGF expression by axitinib and dasatinib in PCa cells. Nevertheless, the conflicting effects on PPC-1 and TSU-Pr1 cells raise concern in applying these drugs for PCa patients treatment. The contradictory response of PPC-1 and TSU-Pr1 cells were probably due to the difference in the molecular phenotype of the cell lines. Guo et al., (2010) explained the different sensibility of prostate cancer cells to apoptosis after exposed with a protein kinase C inhibitor. The TK receptors, PGDFR and c-KIT were inhibited by the biochemical activity of axitinib and dasatinib. Only 16% of prostate cancer cells express the PGDFR associated with cell growth, migration and angiogenesis (Claesson-Welsh, 1994), so the c-KIT expression was targeted. In this study, the western blot and fluorescent immunocytochemistry studies showed that both PPC-1 and TSU-Pr1 cells express the c-KIT protein. Although the c-KIT function remains unclear, its expression is associated with lateral stages of PCa (Paronetto, 2004). The combined drug treatment in TSU-Pr1 cells paradoxically enhanced cell viability and enhanced the expression of angiogenetic factor VEGF. This study also suggested that difference in response to axitinib and dasatinib between PPC-1 and TSU-Pr1 cells might be related to variance in expression of c-KIT isoforms such as tr-KIT.
Conclusion
The combination of axitinib and dasatinib showed a decrease in viability and increase in apoptosis in PPC-1 cells by altering the expression and activity of target regulators and it had no effect on TSU-Pr1 cells. The contradictory and paradoxical results obtained with axitinib and dasatinib in PPC-1 and TSU-Pr1 cells also proved that combined drug treatment do not always produce effective results in prostate cancer treatment.
References
Claesson-Welsh L. Platelet-derived growth factor receptor signals. J Biol Chem. 1994; 269: 32023–26.
Colombel M, Symmans F, O'toole KGS, Chopin D, Benson M, Olsson C, Korsmeyer S, Buttyan R. Detection of apoptosis suppressing oncoprotein bcl-2 in hormone-refractory human prostate cancers. Am J Pathol. 1993; 143: 390-400.
Connor K, Gill C, Tacke M, Rehmann FJ, Strohfeldt K, Sweeney N, Fitzpatrick JM, Watson RW. Novel titanocene anti-cancer drugs and their effect on apoptosis and the apoptotic pathway in prostate cancer cells. Apoptosis 2006; 11: 1205–14.
Correia S, Alves MG, Oliveira PF, Alves MR, van Pelt AM, Cavaco JE, Socorro S. Transgenic overexpression of regucalcin leads to suppression of thapsigargin- and actinomycin D-induced apoptosis in the testis by modulation of apoptotic pathways. Andrology 2014; 2: 290–98.
Correia S, Alves MR, Cavaco JE, Oliveira PF, Socorro S. Estrogenic regulation of testicular expression of stem cell factor and c-kit: Implications in germ cell survival and male fertility. Fertil Steril. 2014; 102: 299–06.
Fiandalo MV, Kyprianou N. Caspase control: Protagonists ofcancer cell apoptosis. Exp Oncol. 2012; 34: 165-75.
Gelmann EP. Molecular biology of the androgen receptor. J Clin Oncol. 2002; 20: 3001-15.
Greenlee RT, Hill-Harmon MB, Murray T, Thun M. Cancer Statistics 2001. CA Cancer J Clin. 2001; 51: 15-36.
Guo J, Zhu T, Chen L, Nishioka T, Tsuji T, Xiao ZX, Chen CY. Differential sensitization of different prostate cancer cells to apoptosis. Genes Cancer. 2010; 1: 836-46.
Hensley P, Mishra M, Kyprianou N. Targeting caspases in cancer therapeutics. Biol Chem. 2013; 394: 831-43.
Jurgensmeier JM, Xie Z, Deveraux Q, Ellerby L, Bredesen D, Reed JC. Bax directly induces release of cytochrome c from isolated mitochondria. Proc Natl Acad Sci USA. 1998; 95: 4997–02.
Kelly RJ, Rixe O. Axitinib-a selective inhibitor of the vascular endothelial growth factor (VEGF) receptor. Target Oncol. 2009; 4: 297–05.
Kubler HR, van Randenborgh H, Treiber U, Wutzler S, Battistel C, Lehmer A, Wagenpfeil S, Hartung R, Paul R. In vitro cytotoxic effects of imatinib in combination with anticancer drugs in human prostate cancer cell lines. Prostate 2005; 63: 385–94.
McKenzie S, Kyprianou N. Apoptosis evasion: The role of survival pathways in prostate cancer progression and therapeutic resistance. J Cell Biochem. 2006; 97: 18–32.
Paronetto MP, Farini D, Sammarco I, Maturo G, Vespasiani G, Geremia R, Rossi P, Sette C. Expression of a truncated form of the c-Kit tyrosine kinase receptor and activation of Src kinase inhuman prostatic cancer. Am J Pathol. 2004; 164: 1243-51.
Pienta KJ, Smith DC. Advances in prostate cancer chemotherapy: A new era begins. CA Cancer. J Clin. 2005; 55: 300-18.
Pinto AC, Angelo S, Moreira JN, Simoes S. Schedule treatment design and quantitative in vitro evaluation of chemotherapeutic combinations for metastatic prostate cancer therapy. Cancer Chemother Pharmacol. 2011; 67: 275–84.
Roberts E, Cossigny DA, Quan GM. The role of vascular endothelial growth factor in metastatic prostate cancer to the skeleton. Prostate Cancer. 2013; 2013: 418340.
Solowiej J, Bergqvist S, McTigue MA, Marrone T, Quenzer T, Cobbs M, Ryan K, Kania RS, Diehl W, Murray BW. Characterizing the effects of the juxtamembrane domain on vascular endothelial growth factor receptor-2 enzymatic activity, autophosphorylation, and inhibition by axitinib. Biochemistry 2009; 48: 7019–31.
Srinivasula SM, Ahmad M, Fernandes-Alnemri T, Alnemri ES. Autoactivation of procaspase-9 by Apaf-1-mediated oligomerization. Mol Cell. 1998; 1: 949–57.
Stupack DG. Caspase-8 as a therapeutic target in cancer. Cancer Lett. 2013; 332: 133–40.
Vaz CV, Alves MG, Marques R, Moreira PI, Oliveira PF, Maia CJ, Socorro S. Androgen-responsive and nonresponsive prostate cancer cells present a distinct glycolytic metabolism profile. Int J Biochem Cell Biol. 2012; 44: 2077–84.
Waldman T, Kinzler KW, Vogelstein B. P21 is necessary for the p53-mediated G1 arrest in human cancer cells. Cancer Res. 1995; 55: 5187–90.
Youle RJ, Strasser A. The BCL-2 protein family: Opposing activities that mediate cell death. Nat Rev Mol Cell Biol. 2008; 9: 47–59.
Yu SQ, Lai KP, Xia SJ, Chang HC, Chang C, Yeh S. The diverse and contrasting effects of using human prostate cancer cell lines to study androgen receptor roles in prostate cancer. Asian J Androl. 2009; 11: 39–48.
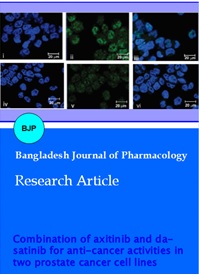