Taraxerone triterpene inhibits cancer cell growth by inducing apoptosis in non-small cell lung cancer cells
Abstract
The objective of the present study was to evaluate the anti-cancer effects of taraxerone in non-small cell lung cancer cells (A-549). The effect of this compound on apoptosis and cellular morphology was also studied. MTT (3-(4, 5-dimethylthiazol-2-yl)-2, 5-diphenyltetrazolium bromide) assay was used to evaluate the effect of the compound on cell viability. Cellular morphology after taraxerone treatment was demonstrated by fluorescence microscopy and transmission electron microscopy. The results revealed that taraxerone induced dose-dependent as well as time-dependent growth inhibitory effects on A-549 cell line showing an IC50 value of 53.2 and 61.6 µM at 24 and 48 hours time intervals respectively. It also inhibited cancer cell colony formation in a dose-dependent manner and around 90% of the cancer cells were destroyed by the 125 µM dose of taraxerone treatment. Taraxerone also induced cell shrinkage, chromatin condensation and nuclear membrane rupture which are characteristic of apoptosis.
Introduction
Lung cancer is the leading cause of cancer related deaths in China and many other parts of the world (Jemal et al., 2010). The overall survival rate is 15%, and only 20-25% of cases present at an early stage. The standard care for first stage non-small-cell lung cancer is surgical resection followed by chemotherapy. However, the post-resection survival is only approximately 50% at 5 years. Approximately 75–85% of all lung cancers belong to non-small-cell lung cancer, an aggressive tumor type that has improved little over the last 35 years (Ferlay et al., 2010; Ferreira and Tolis, 1999). Lung cancer causes approximately 1.4 million deaths annually, as reported in 2008. Platinum-based drugs are widely used to treat patients with non-small-cell lung cancer in clinics. Nonetheless, drug-resistance frequently develops in these patients. For example, approximately 70% of non-small-cell lung cancer patients with advanced unresectable or prevalent incurable metastasis are candidates for neoadjuvant or palliative chemotherapy. However, approximately two-thirds of these patients are not benefitted by conventional chemotherapy (Ferreira and Tolis, 1999). Therefore, there is acrucial need to search for new chemotherapeutic agents with superior activity and less chances of getting resistance against lung cancer.
A number of studies have drawn attention to natural products extracted from Chinese medicinal herbs as anti-cancer agents in cancer therapy (Cragg et al., 1997; Newman and Cragg, 2007; Chen et al., 2012; Li et al., 2010). Among natural products, natural triterpenes represent a structurally diverse group of organic compounds with potent bioactivity including antitumor effects. Several triterpenoids, including ursolic and oleanolic acid, betulinic acid, celastrol, pristimerin, lupeol, and avicins have been reported to possess anti-cancer activity (Petronelli et al., 2009).
In the present research work, we report the anti-cancer and apoptotic inducing effects of taraxerone (Figure 1) triterpene in non-small cell lung cancer (A-549) cells. The current report constitutes the first such report on this naturally occurring triterpene.
Figure 1: Chemical structure of taraxerone
Materials and Methods
Chemicals and other reagents
Taraxerone was purchased from Sigma Chemical Company (USA). For in vitro studies, taraxerone was dissolved in dimethyl sulfoxide to create a stock solution (0.1 moL/L), which was stored at -20°C. To prepare working solutions, the stock solution was further diluted with culture media to yield the desired taraxerone concentrations. Control cells were treated with an equal volume of vehicle. The dimethyl sulfoxide concentration was kept below 0.1% in cell culture and did not show any noticeable effect on cell growth or cell death. Dulbecco’s modified Eagle’s medium, fetal bovine serum (FBS), penicillin-streptomycin were obtained from Hangzhou Sijiqing Biological Products Co. Ltd. China. MTT kit was obtained from Roche (USA). Hoechst 33258 was purchased from Beyotime (China). All other chemicals and solvents used were of the highest purity grade.
Cell line
Human non-small cell lung cancer cell line (A-549) was purchased from the Shanghai Institute of Cell Biology, Chinese Academy of Sciences (CAS, China) and was kept at 37°C in a humidified atmosphere containing 5% CO2. The cells were cultured in Dulbecco’s modified Eagle’s media supplemented with 10% fetal bovine serum and 100 U/mL penicillin and 100 μg/mL streptomycin.
Cell viability evaluation by MTT assay
Inhibition of cell growth by taraxerone was evaluated by the MTT assay. Briefly, cells were plated in 96-well culture plates (1 × 105 cells/well). After 24 hours incubation, cells were treated with taraxerone (0, 5, 10, 25, 50, 75 and 125 μM, eight wells per concentration) for 24, 48 and 72 hours, MTT solution (5 mg/mL) was then added to each well. After 4 hours incubation, the formazan precipitate was dissolved in 100 μL dimethyl sulfoxide, and then the absorbance was measured in an ELISA reader (Thermo Molecular Devices Co., USA) at 570 nm.
Calculation of cell cytotoxicity
The cell viability ratio was calculated by the following formula:
Inhibitory ratio (%) = (OD control – OD treated) / OD control × 100%
Cytotoxicity was expressed as the concentration of taraxerone inhibiting cell growth by 50% (IC50 value).
Colony formation assay
A-549 cell lines were suspended in 1 mL of DMEM containing 0.5% agarose (Amresco, USA) and 10% FBS, and plated on a bottom layer containing 1.0% agarose and 10% FBS in 6-well plate in triplicate. After 1 week, plates were stained with 0.5% gentian violet and the colonies were counted under light microscope.
Morphological study of apoptosis using fluorescence microscopy
A-549 cell lines were seeded into 12-well plates at a density of 1 x 106 cells/ well in 1 mL medium. After the treatment with the different concentrations (0, 25, 75 and 125 µM) of taraxerone for 48 hours, cell apoptosis was determined by the Hoechst staining kit according to the manufacturer's instruction. After the treatment, cells were fixed with 3.5% polyoxymethylene and then incubated in Hoechst solution for 20 min in the dark. The resulting images were recorded using a UV fluorescence microscope (Olympus, Japan) using UV filter at 400x magnification to detect morphological evidence of apoptosis.
Transmission electron microscopy evaluation
A-549 cell lines were seeded and grown at 5 × 105/mL in three flasks. Cells after treated with different concentrations (0, 25, 75 and 125 µM) of taraxerone for 48 hours were harvested and washed with PBS twice, and then added to 2.5% glutaraldehyde fixative for microtome sectioning using ultramicrotome (LKB-V; JEOL Co; Japan). TEM was carried out with a Transmission Electron Microscope (JEM-2000EX; JEOL Co; Japan).
Statistical analysis
The experiments were performed in triplicate. Data were expressed as the mean ± standard deviation (SD). Statistical correlation of data was checked for significance by ANOVA and Student's t-test. p<0.05 was considered to indicate a statistically significant difference.
Results
Taraxerone inhibits cancer cell proliferation and colony formation in A-549 cell lines. Initially we verified the cytotoxic activity of taraxerone on A-549 cells by using MTT assay. The results revealed that taraxerone had power fulcytotoxic effects on A-549 cells. It revealed both concentration-dependent as well as time-dependent growth inhibitory effects against these cells (Figure 2). The IC50 value was calculated to be 53.2 and 61.6 µM at 24 hours and 48 hours time interval respectively. Taraxerone also inhibited the clonogenic activity of the A-549 cancer cells by reducing the number of cancer colony forming cells.
Figure 2: Cytotoxic effect of taraxerone in human non-small cell lung cancer cells (A-549). Data are shown as the mean ± SD of three independent experiments; ap< 0.05, bp< 0.01, vs 0 µM (control)
Clonogenic reduction also followed the concentration dependence on taraxerone (Figure 3). Around 90% of the cancer cells were destroyed by the 125 µM dose of taraxerone treatment. The results specify that taraxerone has the propensity to prevent both cell proliferation (anchorage-dependent) and colony formation (anchorage-independent) growth of A-549 cancer cells in vitro.
Figure 3: Inhibitory effect of taraxerone on human non-small cell lung cancer cells (A-549). The colony formation assay of A-549 cells treated with taraxerone at specified doses
Fluorescence microscopic study of taraxerone-induced cellular morphological changes
In case of fluorescence microscopy, A-549 cells were stained and evaluated for nuclear shape using a fluorescence microscope with Hoechst 33258 staining (Figure 4A-D). The results revealed that taraxerone-treated cells showed considerable chromatin condensation or dense staining fragmentation called apoptotic bodies, which inferred an early apoptotic event. The presence of such apoptotic bodies was related with taraxerone dose. A-549 cells underwent the morphologic changes typical of apoptosis after treatment with the compound.
Figure 4: Effect of taraxerone on the nuclear morphology of A-549 cancer cells using Hoechst 33258 staining at actual magnification 200x. A-549 cells were treated without (A) and with taraxerone 25 μM (B), 75 μM (C), and 125 μM (D) for 48 hours. White arrows represent apoptotic cells exhibiting chromatin condensation and nuclear fragmentation
Further, the effects of taraxerone on A-549 cancer cell morphology were verified by acridine orange/ethidium bromide staining using fluorescence microscopy. The results of this experiment (Figure 5A-D) were similar to other two experiments indicating that taraxerone induces cell death through the intervention of apoptosis.
Figure 5: Fluorescence microscopic study of human non-small cell lung cancer cells (A-549) stained with a combination of acridine orange: Ethidium bromide (1:1 ratio). A shows untreated control cells, B shows A-549 cells treated with 25 μM dose of taraxerone, C shows cells treated with 75 μM dose and D shows cells treated with 125 μM dose of taraxerone
Transmission electron microscopy of A-549 cells after taraxerone treatment
Transmission electron microscopy was used to observe the ultrastructural changes induced by taraxerone in A-549 cells and to assess the intracellular destruction caused by this compound for 48 hours. Taraxerone-treated cells revealed morphological changes characteristic of apoptosis. As shown in Figure 6A-D, clear nuclear fragmentation was found in the treated A-549 cells, while the untreated cells exhibited a fairly intact morphology. These ultrastructural changes in their morphology was strongly dose-dependent.
Figure 6: Effect of taraxerone on cell apoptosis observed by transmission electron microscopy (TEM). Human lung cancer cells (A-549) were treated with (B) 25 μM, (C) 75 μM and (D) 125 μM taraxerone for 48 hours. (A) shows the control (untreated) group
Discussion
Current study showed the antitumor effects of taraxerone, a naturally occurring triterpene, against A-549 cells. The study also focused on the effect of this triterpene on apoptosis induction. MTT assay showed that the compound exerted a potent antiproliferative effects which showed strong dose-dependence as well as time-dependence. Taraxerone also led to a significant reduction of cancer cells following treatment with varying doses. Further, fluorescence microscopy revealed that taraxerone was capable of inducing cellular shrinkage and chromatin condensation. This cell shrinkage and chromatin condensation is a hallmark of apoptosis. We used two staining dyes viz., Hoechst 33258 and acridine orange/ethidium bromide, both of which confirmed that taraxerone is a potent apoptosis inducing agent. Transmission electron microscopy showed that taraxerone could induce powerful and dose-dependent nuclear membrane rupture.
Literature survey revealed that taraxerone has been reported to exhibit anticancer effects in human chronic myelogenous leukemia cell line K562 (Biswas et al., 2009). However, the authors did not report the mode of action of this compound in this cell line i.e., whether this compound shows anticancer activity by inducing apoptosis or not. Our current findings report the anticancer and apoptotic effects of taraxerone on a different cell line (non-small cell lung cancer cell line). We also demonstrated its mode of action by studying its effect on apoptosis, and cellular membrane rupture.
Inhibition of cancer development often implicates modulation of signal transduction pathways, resulting in alterations in gene expression, arrest in cell cycle phase distribution or apoptosis. Apoptotic cell death characterizes a universal and highly proficient suicide pathway, considered as a perfect method for eradication of damaged cells (Earnshaw, 1995; Elmore, 2007). Recently, the apoptosis signaling pathway has been revealed to deliver encouraging targets for the development of novel anticancer agents (Khoo et al., 2010). Several plant-based bioactive compounds are known to be chemopreventive agents capable of inducing apoptosis in a number of experimental models of carcinogenesis. Thus induction of apoptosis is considered as a possible mechanism of chemopreventive agents (Moongkarndi et al., 2004). Regulation of apoptotic signaling pathways incorporates a complicated system consisting of several pathways. Many conventional anticancer drugs are currently used in anticancer chemotherapy which are thought to induce cell apoptosis through the stimulation of these pathways (Adams and Cory, 2007). Consequently, the ability of chemotherapeutic agents to induce the apoptotic cell suicide has been recognized as one of the major mechanisms which might serve for the development of novel approaches to treat cancer. There are two apoptotic pathways which have been documented; the intrinsic pathway is principally controlled by the members of the Bcl-2 family proteins (Reed, 2000). Several anticancer drugs function primarily to induce apoptosis in cancer cells and inhibit tumor development. The morphological changes of apoptosis observed in most cell types primarily start with a reduction in cell volume and condensation of the nucleus. In several cases extensive DNA damage leads to activation of cell cycle check points and leads to cell cycle arrest and apoptosis. Dysregulation in the cell division and apoptosis are connected to the development of most cancers (Pietenpol and Stewart, 2002).
Conclusion
Taraxerone exerts anticancer effects in A-549 lung cancer cells by inducing both early and late apoptosis, chromatin condensation and cell membrane rupture.
References
Adams J, Cory S. The Bcl-2 apoptotic switch in cancer development and therapy. Oncogene 2007; 26: 1324-37.
Biswas M, Mandal NB, Palit P, Ghosh AK, Bannerjee S, Haldar P K. In vitro anti-leishmanial and anti-tumour activities of a pentacyclic triterpenoid compound isolated from the fruits of Dregea volubilis Benth Asclepiadaceae. Trop J Pharm Res. 2009; 8: 127-31.
Chen J, Shi DY, Liu SL, Zhong L. Tanshinone IIA induces growth inhibition and apoptosis in gastric cancer in vitro and in vivo. Oncol Rep. 2012; 27: 523-28.
Cragg GM, Newman DJ, Weiss RB. Coral reefs, forests, and thermal vents: The worldwide exploration of nature for novel antitumor agents. Semin Oncol. 1997; 24: 156-63.
Earnshaw WC. Nuclear changes in apoptosis. Curr Opin Cell Biol. 1995; 7: 337-43.
Elmore S. Apoptosis: A review of programmed cell death. Toxicol Pathol. 2007; 35: 495-516.
Ferlay J, Shin HR, Bray F, Forman D, Mathers C, Parkin DM. Estimates of worldwide burden of cancer in 2008: GLOBOCAN 2008. Int J Cancer. 2010; 127: 2893–917.
Ferreira CG, Tolis C, Giaccone G. p53 and chemosensitivity. Ann Oncol. 1999; 10: 1011–21.
Jemal A, Siegel R, Xu J, Ward E. Cancer statistics, 2010. CA Cancer J Clin. 2010; 60: 277–300.
Khoo BY, Chua SL, Balaram P. Apoptotic effects of chrysin in human cancer cell lines. Int J Mol Sci. 2010; 11: 2188–99.
Li N, Fan LL, Sun GP, Wan XA, Wang ZG, Wu Q, Wang H. Paeonol inhibits tumor growth in gastric cancer in vitro and in vivo. World J Gastroenterol. 2010; 16: 4483-90.
Ma L, Wen ZS, Liu Z, Hu Z, Ma J, Chen XQ, Liu YQ, Pu JX, Xiao WL, Sun HD, Zhou GB. Overexpression and small molecule-triggered down-regulation of CIP2A in lung cancer. PLoS One. 2011; 6: e20159.
Moongkarndi P, Kosem N, Kaslungka S, Luanratana O, Pongpan N, Neungton N. Antiproliferation, antioxidation and induction of apoptosis by Garcinia mangostana (mangosteen) on SKBR3 human breast cancer cell line. J Ethnopharmacol. 2004; 90: 161–66.
Newman DJ, Cragg GM. Natural products as sources of new drugs over the last 25 years. J Nat Prod. 2007; 70: 461–77.
Petronelli A, Pannitteri G, Testa U. Triterpenoids as new promising anticancer drugs. Anticancer Drugs. 2009; 20: 880-92.
Pietenpol JA, Stewart ZA. Cell cycle checkpoint signaling: Cell cycle arrest versus apoptosis. Toxicology 2002; 181–182: 475–81.
Reed J. Mechanisms of apoptosis. Am J Pathol. 2000; 157: 1415-30.
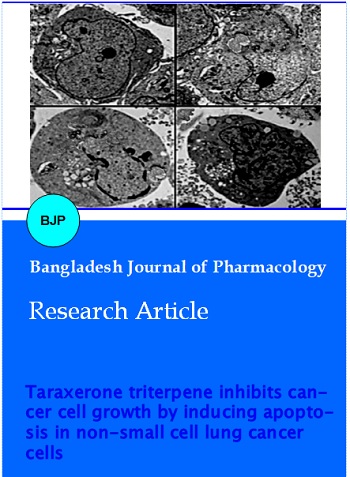