Inhibitory effect of Sphagnum palustre extract and its bioactive compounds on aromatase activity
Abstract
Sphagnum palustre (a moss) has been traditionally used in Korea for the cure of several diseases such as cardiac pain and stroke. In this research, the inhibitory effect of S. palustre on aromatase (cytochrome P450 19, CYP19) activity was studied. [1β-3H] androstenedione was used as a substrate and incubated with S. palustre extract and recombinant human CYP19 in the presence of NADPH. S. palustre extract inhibited aromatase in a concentration-dependent manner (IC50 value: 36.4 ± 8.1 µg/mL). To elucidate the major compounds responsible for the aromatase inhibitory effects of S. palustre extract, nine compounds were isolated from the extract and tested for their inhibition of aromatase activity. Compounds 1, 6, and 7 displayed aromatase inhibition, while the inhibition by the other compounds was negligible.
Introduction
Cytochrome P450, as a key enzyme in estrogen biosynthesis, is well-known for its conversion of C-19 androgens into C-18 estrogens (Chumsri et al., 2011). The enzyme is distributed in many tissues including gonads, brain, liver, adipose tissue, placenta, skin, bone, and endometrium as well as in the ovary and testis. In breast cancer patients, excess estrogen and aromatase levels have been observed, and it was previously reported that aromatase inhibition can be considered as one of the most efficient treatments in breast cancer therapy, especially for postmenopausal women (Cazzaniga and Bonanni, 2012; Renoir et al., 2013). In cases of metastatic breast cancer, aromatase inhibitor is used as first-line therapy, prior to the other options (Altundag and Ibrahim, 2006). However, there were reports on natural products with aromatase inhibition effects by several studies. Compared to aromatase inhibitors, these nature-deriving compounds exhibit higher tissue-specific inhibition as well as reduced toxicity (Balunas and Kinghorn, 2010; Balunas et al., 2008).
Sphagnum palustre (Sphagnaceae) is an aquatic moss. It is one of the most globally common types of moss. In Korea, S. palustre has long been used as a traditional medicine; the “Donguibogam†describes the use of S. palustre for the treatment of cardiac pain and stroke (Nam et al., 2011). A recent phytochemical investigation on S. palustre reported the isolation of phenolic, flavonoid, triterpenoid, and steroid compounds (Nam et al., 2011). However, in spite of its ubiquitous existence and medicinal usage, the phytochemical profile of S. palustre remains unclear.
In our previous study, the ethanol extract of S. palustre was reported to exhibit significant renoprotective effects against cisplatin-induced damage in kidney cells, with the relevant compounds having been identified (Kang et al., 2016). As part of our ongoing search for bioactive metabolites from S. palustre, we investigated the inhibition of S. palustre on aromatase (cytochrome P450 19, CYP19) and the active compounds. No scientific evidence has been reported related to its inhibitory activity on aromatase. In this study, we report the inhibitory effect of S. palustre extract and nine compounds (1-9) on aromatase activity using recombinant human CYP19 (Figure 1).
Figure 1: Chemical structures of the isolated compounds 1-9
Materials and Methods
General experimental procedures
Optical rotations were obtained on a Jasco P-1020 polarimeter. IR spectra were acquired on a Bruker IFS-66/S FT-IR spectrometer. Circular dichroism spectra were recorded with a JASCOJ-810 spectropolarimeter. Ultraviolet (UV) spectra were acquired on an Agilent 8453 UV−visible spectrophotometer. Electrospray ionization mass spectra were recorded on a Waters UPLC-QTOF Xevo G2-S mass spectrometer. Nuclear magnetic resonance spectra were recorded on a Bruker AVANCE III 700NMR spectrometer operating at 700 MHz (1H) and 175 MHz (13C),with chemical shifts given in ppm (δ). Semi-preparative high performance liquid chromatography (HPLC) used a Shimadzu Prominence HPLC System with SPD-20A/20AV Series Prominence HPLC UV-Vis Detectors. Column chromatography was conducted with a silica gel 60 (Merck, 70–230 mesh and 230–400 mesh) and RP-C18 silica gel (Merck, 230–400 mesh). The packing material for molecular sieve column chromatography was sephadex LH-20. Merck precoated silica gel F254 plates and reverse-phase (RP)-18 F254s plates (Merck) were used for thin-layer chromatography.
Plant material
Whole dried S. palustre harvested from the pristine environment of the Southern West coast of New Zealand was purchased as a commercial grade production in August 2014. S. palustre was identified by one of the authors (K.H.K.). A voucher specimen (SKK-Mek-2014) was stored in the herbarium of the School of Pharmacy, Sungkyunkwan University, Suwon, Korea.
Extraction and isolation
Dried S. palustre (827 g) was extracted for 1 day with 18 L of 100% ethanol at room temperature. The extract was then filtered and the filtrate was evaporated under reduced pressure using a rotavapor to obtain ethanol extract (5.2 g). The ethanol extract was successively divided by preparative reverse-phase HPLC equipped with a YMC-Pack Pro C18 RS column (250 mm x 20 mm i.d., 5 μm, 8 nm; YMC, Japan), using a gradient solvent system [water-acetonitrile (ACN): 0-20 min: 30% ACN; 20-40 min: 70% ACN; 40-60 min: 100% ACN, flow rate: 7 mL/min] to obtain six fractions (F1–F6). Fraction F4 (1 g) was further purified using sephadex LH-20 column chromatography with 100% methanol and afforded five subfractions (F4-1 to F4-5). Subfraction F4-4 (92 mg) was purified using semipreparative reversed-phase HPLC with a solvent system of 65% methanol using an ODS-M80 column (250 mm x 10 mm i.d., 4 μm, 8 nm: YMC) to furnish compound 1 (2.0 mg). Fraction F6 (800 mg) was purified using preparative reverse-phase HPLC with 100% methanol to yield six subfractions (F6-1 to F6-6). Sub-fraction F6-3 (262 mg) was subjected to a Sephadex LH-20 column chromatography and a solvent system of 100% methanol was used to give six subfractions (F6-3-1 to F6-3-6). Sub-fraction F6-3-3 (77 mg) was further separated using semipreparative reversed-phase HPLC with 90% methanol to furnish compounds 4 (0.1 mg) and 5 (0.7 mg). Compound 3 (0.7 mg) was re-crystallized from sub-fraction F6-3-3 (13 mg). Subfraction F6-4 (31 mg) was purified by semi-preparative reverse-phase HPLC using 95% methanol as solvent to give compounds 6 (0.3 mg), 8 (7.7 mg), and 9 (0.1 mg). Subfraction F6-5 (25 mg) was purified by semipreparative reverse-phase HPLC with 97% methanol as solvent to afford compound 2 (2.3 mg). Finally, subfraction F6-6 (74 mg) was separated using semipreparative reverse-phase HPLC utilizing a gradient solvent system (water-methanol: 0-40 min: 90% methanol; 40-50 min: 100% methanol) to yield compound 7 (0.3 mg).
Aromatase enzyme activity
Microsomal aromatase assay was conducted utilizing recombinant human CYP19 Supersomes® (BD Bio-sciences) with NADPH solution A and NADPH solution B (BD Biosciences). Solution A was composed of 31 mM NADP+, 66 mM glucose-6-phosphate, and 66 mM MgCl2 in H2O; Solution B was composed of 40 U/mL glucose-6-phosphate dehydrogenase in 5 mM sodium citrate. S. palustre extract and its isolates (1-9) were treated 25 nM [1β-3H] androstenedione and NADPH solutions A and B, the reaction was started by adding 2 pmol Supersomes®, and incubated for 1 hour at 37°C according to manufacturer’s protocol. The reactions were terminated by vortexing with chloroform. 5% charcoal/0.5% dextran was added to the aqueous supernatants to remove radioactive residues. The supernatant was obtained, and aromatase activity was calculated by measuring [3H] H20 using a liquid scintillation counter (LS-6500, Beckman counter).
Data analyses
Sigma Plot 12.0 (Jandel Science Software) and Excel 2007 (Microsoft) were employed for data analysis. Each in vitro assay was carried out at least in triplicate and the data from each assay are expressed as mean ± standard deviation (SD). Statistical analyses were carried out using SPSS version 21.0 software (SPSS Inc.). Differences between experimental groups were determined by Duncan’s post hoc test following one-way analysis of variance (ANOVA). Differences with p<0.05 were considered statistically significant.
Results
The S. palustre extract inhibited activity of aromatase in a concentration dependent manner, with significant inhibition observed at ≥20 µg/mL (Figure 2). At a concentration of 50 µg/mL of S. palustre extract, the aromatase activity was lowered to 36.3 ± 7.3 of the level observed with control (p< 0.01).
Figure 2: Inhibitory effects of S. palustre extract on aromatase activity. Tritiated androstenedione [1β-3H] was mixed with various concentrations (5–50 μg/mL) of S. palustre extract treated with recombinant CYP19 (CYP BD-SupersomesTM) for 1 hour. 10-6 M HA (4-androsten-4-ol-3,17-dione) was used as a positive control (P.C). The results are expressed as mean ± SD of three separate experiments for each group. *Represents the statistical difference from the vehicle control [Con, 0.1% Dimethyl sulfoxide (DMSO)]: ap< 0.01
To elucidate the bioactive compounds responsible for the aromatase inhibitory effects of S. palustre extract, total nine compounds were isolated and these were identified as (-)-naringenin (1) (Jeon et al., 2008; Prescott et al., 2002), ergosterol peroxide (2) (Krzyczkowski et al., 2009), betulinic acid (3) (Siddiqui et al., 1988), maslinic acid (4) (Taniguchi et al., 2002), oleanolic acid (5) (Seebacher et al., 2003), 8-monooxoelaidic acid (6) (Kawagishi et al., 2002), 8-monooxoelaidic acid methyl ester (7) (Dang et al., 2008), palmitic acid (8) (Couperus et al., 1978), and tetradecanoic acid (9) (Feldhues and Schafer, 1985; Narayanaswamy et al., 2006) by comparing their spectroscopic data including 1H and 13C NMR and MS data with those previously reported in other literatures. All of the isolates were tested for their inhibitory potential against aromatase activity. In the present study, compounds 1, 6 and 7 displayed aromatase inhibitory effects, with inhibition by the other compounds being negligible (Table I).
Table I: Aromatase inhibitory activity of compounds from S. palustre
Compound | Aromatase inhibitiona (% of control) |
---|---|
1 | 64.8a |
2 | 93.7 |
3 | 97.4 |
4 | 96.2 |
5 | 92.5 |
6 | 54.7 |
7 | 54.4 |
8 | 96.7 |
9 | 93.6 |
Discussion
The ethanol extract of S. palustre inhibited aromatase activity in a concentration-dependent manner. Aromatase inhibition of S. palustre extract is comparable with green tea extract (IC50 in microsome: 28 µg/mL), a recognized inhibitor of aromatase (Satoh et al., 2002). Fungi also inhibited aromatase activity (Fatima et al., 2014).
The results of this study showed that there was significant inhibition of aromatase activity by compound 1, (-)-naringenin, which is a main flavonoid component of S. palustre. Previous studies have reported that flavonoids inhibit the activity of aromatase (Campbell and Kurzer, 1993; Jeong et al., 1999; Ibrahim and Abul-Hajj, 1990). (-)-Naringenin has also been studied as a strong and moderate aromatase inhibitor in microsomes and recombinant human CYP19 (Balunas et al., 2008; Edmunds et al., 2005). Thus, one of flavonoids, the compound 1 is a potential aromatase inhibitor in S. palustre extract. In addition, two fatty acids, compounds 6 and 7 displayed potent aromatase inhibitor activity. A series of fatty acids have been explored for their potential aromatase inhibition, with aromatase inhibitor activity by several natural fatty acids being confirmed (Balunas et al., 2008). Presently, of the isolated fatty acids (compounds 6-9), compounds 6 and 7 which contain an unsaturated ketone moiety, exhibited aromatase inhibitor activity. This suggests that the unsaturated ketone unit in fatty acids may be a key functional moiety for inhibition of aromatase activity.
Conclusion
S. palustre extract decreased estrogen biosynthesis by inhibiting aromatase activity. The active compounds responsible for the inhibition effect were identified. The identification of the inhibitory effects on aromatase of S. palustre extract and identification of the bioactive compounds are novel findings. S. palustre extract could inhibit aromatase activity, with compounds 1, 6, and 7 identified as the active components. The findings provide the foundation for the development of pharmacologically useful aromatase inhibitors.
References
Altundag K, Ibrahim NK. Aromatase inhibitors in breast cancer: An overview. Oncologist 2006; 11: 553-62.
Balunas MJ, Kinghorn AD. Natural compounds with aromatase inhibitory activity: An update. Planta Med. 2010; 76: 1087-93.
Balunas MJ, Su B, Brueggemeier RW, Kinghorn AD. Natural products as aromatase inhibitors. Anticancer Agents Med Chem. 2008; 8: 646-82.
Campbell DR, Kurzer MS. Flavonoid inhibition of aromatase enzyme activity in human preadipocytes. J Steroid Biochem Mol Biol. 1993; 46: 381-88.
Cazzaniga M, Bonanni B. Breast cancer chemoprevention: Old and new approaches. J Biomed Biotechnol. 2012; 2012: 985620.
Chumsri S, Howes T, Bao T, Sabnis G, Brodie A. Aromatase, aromatase inhibitors, and breast cancer. J Steroid Biochem Mol Biol. 2011; 125: 13-22.
Couperus PA, Clague ADH, Van Dongen JPCM. Carbon-13 chemical shifts of some model carboxylic acids and esters. Org Magn Reson. 1978; 11: 590-97.
Dang HT, Lee HJ, Yoo ES, Shinde PB, Lee YM, Hong J, Kim DK, Jung JH. Anti-inflammatory constituents of the red alga Gracilaria verrucosa and their synthetic analogues. J Nat Prod. 2008; 71: 232-40.
Edmunds KM, Holloway AC, Crankshaw DJ, Agarwal SK, Foster WG. The effects of dietary phytoestrogens on aromatase activity in human endometrial stromal cells. Reprod Nutr Dev. 2005; 45: 709-20.
Fatima N, Kalsoom A, Mumtaz A, Muhammad SA. Computational drug designing of fungal pigments as potential aromatase inhibitors. Bangladesh J Pharmacol. 2014; 9: 575-79.
Feldhues M, Schäfer HJ. Selective mixed coupling of carboxylic acids (II). Photolysis of unsymmetrical diacylperoxides with alkenyl-, halo-, keto-, carboxyl-groups and a chiral α-carbon: Comparison with the mixed kolbe electrolysis. Tetrahedron 1985; 41: 4213-35.
Ibrahim AR, Abul-Hajj YJ. Aromatase inhibition by flavonoids. J Steroid Biochem Mol Biol. 1990; 37: 257-60.
Jeon SH, Chun W, Choi YJ, Kwon YS. Cytotoxic constituents from the bark of Salix hulteni. Arch Pharm Res. 2008; 31: 978-82.
Jeong HJ, Shin YG, Kim IH, Pezzuto JM. Inhibition of aromatase activity by flavonoids. Arch Pharm Res. 1999; 22: 309-12.
Kang HR, Lee D, Eom HJ, Lee SR, Lee KR, Kang KS, Kim KH. Identification and mechanism of action of renoprotective constituents from peat moss Sphagnum palustre in cisplatin-induced nephrotoxicity. J Funct Foods. 2016; 20: 358–68.
Kawagishi H, Miyazawa T, Kume H, Arimoto Y, Inakuma T. Aldehyde dehydrogenase inhibitors from the mushroom Clitocybe clavipes. J Nat Prod. 2002; 65: 1712-14.
Krzyczkowski W, Malinowska E, Suchocki P, Kleps J, Olejnik M, Herold F. Isolation and quantitative determination of ergosterol peroxide in various edible mushroom species. Food Chem. 2009; 113: 351-55.
Nam JH, Jeong JC, Yoon YH, Hong SY, Kim SJ, Jin YI, Lee YJ, Yoo DL, Lee KT, Park HJ. Phytochemical constituents and anticancer activity of Sphagnum palustre extract. Korean J Plant Res. 2011; 24: 40-47.
Narayanaswamy A, Xu H, Pradhan N, Kim M, Peng X. Formation of nearly monodisperse In2O3 nanodots and oriented-attached nanoflowers: Hydrolysis and alcoholysis vs pyrolysis. J Am Chem Soc. 2006; 128: 10310-19.
Prescott AG, Stamford NPJ, Wheeler G, Firmin JL. In vitro properties of a recombinant flavonol synthase from Arabidopsis thaliana. Phytochemistry 2002; 60: 589-93.
Renoir JM, Marsaud V, Lazennec G. Estrogen receptor signaling as a target for novel breast cancer therapeutics. Biochem Pharmacol. 2013; 85: 449-65.
Satoh K, Sakamoto Y, Ogata A, Nagai F, Mikuriya H, Numazawa M, Yamada K, Aoki N. Inhibition of aromatase activity by green tea extract catechins and their endocrinological effects of oral administration in rats. Food Chem Toxicol. 2002; 40: 925-33.
Seebacher W, Simic N, Weis R, Saf R, Kunert O. Complete assignments of 1H and 13C NMR resonances of oleanolic acid, 18α-oleanolic acid, ursolic acid and their 11-oxo derivatives. Magn Reson Chem. 2003; 41: 636–38.
Siddiqui S, Hafeez F, Begum S, Siddiqui BS. Oleanderol, a new pentacyclic triterpene from the leaves of Nerium oleander. J Nat Prod. 1988; 51: 229-33.
Taniguchi S, Imayoshi Y, Kobayashi E, Takamatsu Y, Ito H, Hatano T, Sakagami H, Tokuda H, Nishino H, Sugita D, Shimura S, Yoshida T. Production of bioactive triterpenes by Eriobotrya japonica calli. Phytochemistry 2002; 59: 315-23.
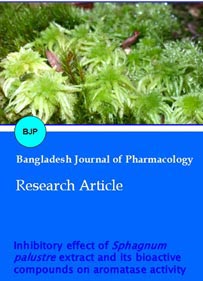