Antibacterial, antifungal and anti-inflammatory activities of Melia azedarach ethanolic leaf extract
Abstract
The aim of the present study is to examine the antibacterial, antifungal and anti-inflammatory activities of the ethanolic leaf extract of Melia azedarach. It was tested in vitro for its antibacterial and antifungal activities against E. coli (ATCC 8739), Enterococcus faecalis (Ec P07) and Bacillus subtilis (Bs) bacteria as well as against pathogenic fungi (Alternaria alternate, Fusarium solani, Fusarium oxysporum sp. melonis, F. oxysporum f. sp. lycopersici, F. sambucinum and Botrytis cinerea) with different techniques similtaneously with anti-inflammatory activity with carageaneen method. All tested concentrations of M. azedarach extract showed significant antibacterial and antifungal activities with low IC50 compared to ethanol 95% and a high anti-inflammatory activity compared with indomethacin as drug. Our investigation pointed that M. azedarach could be considered as a good medicinal agent.
Introduction
In recent years, there are increasing hazardous effects of chemical pesticides on plant and animal health (Aktar et al., 2009) as well as the problem of drugs microorganisms’ resistance have alarmed scientists to search for efficient and eco-friendly alternative ways. In fact, the problem of microbial resistance is growing and the outlook for the use of antimicrobial drugs in the future is still uncertain (Nascimento et al., 2000). Furthermore, the treatment of chronic inflammatory diseases is still problematic, since, anti-inflammatory used drugs have numerous adverse effects (Li et al., 2003). Similarly, infection due to fungal pathogens has become more frequent (Fleming et al., 2002) and affects humans as well as plants. These infections are the leading cause of death in both advanced and developing countries (Murtaza et al., 2015). This is due to the use of immunosuppressive treatments, long-term use of antibiotics and longer survival of immunocompromised individuals (Jain et al., 2010).
Therefore, an increasing demand to evaluate the antimicrobial and antifungal activities of medicinal plant is gaining ground and this for their use in green medicine as it is believed to be safer and cost less than synthetic drugs (Rajkumar and Malathi, 2015; Canli et al., 2015). In fact, plant extracts may represent an ideal solution to these problems; they could be easily tested in vitro (Dissanayake, 2014) and may be an alternative to chemical fungicides and bactericides (Tsair-Bor and Shang-Tzen, 2008). Among the numerous families with recognized pharmacological activities, those belonging to Meliaceae family deserve mention. M. azedarach L. -one of species belonging to Meliaceae family- is wide-spread in Tunisia as an ornamental shade tree. M. azedarach leaves are well-known for their ethnobotanical uses (Gopal et al., 2015).
The present investigation was designed to evaluate the antibacterial, antifungal and anti-inflammatory properties of the leaves ethanolic extract of M. azedarach.
Materials and Methods
Plant material and extraction
M. azedarach mature leaves powder was extracted with soxhlet apparatus as elsewhere (Akacha et al., 2016).
Antibacterial and antifungal assays
The antibacterial and antifungal analysis were carried out using a new tool, phenotype microarrays (Biolog, USA). It offers a unique way to identify product and to infer a mode of action by which the novel inhibitor prevents microbial growth (Bochner et al., 2001). The assays are pre-filled and dried in 96-well microplates that can monitor chemical sensitivities. Cell response in each assay well is determined by the amount of color development produced by the reduction of a tetrazolium compound (a redox indicator) during cell respiration (Bochner, 2003). To identify the method of action of compounds on Gram-negative, Gram-positive bacteria and fungi, phenotype microarrays were employed to screen various sources of carbon, nitrogen, sulfur and phosphorous. The methods were done according to the phenotype microarrays procedure for E. coli and other Gram negative bacteria and the phenotype microarrays procedure for B. subtilis and other Gram-positive bacteria provided by Biolog Inc., USA. Kinetic data were analyzed with OmniLog PM software. The results were expressed by the differences of the treated bacterial cells from untreated bacterial cells.
Phenotype microarrays of compounds with bacteria
E. coli (ATCC 8739), Enterococcus faecalis (Ec P07) and Bacillus subtilis (Bs) were grown overnight at 37°C on nutrient agar plates. In order to prepare the control group bacteria (untreated bacteria), colonies were harvested from the surface of an agar plate with a sterile cotton wool swab and suspended in 16 mL of Inoculating Fluid-0a (IF-0a), in a 16 mL sterile capped glass tube. The cell density must be equaled to 42% transmittance (T) on a biolog turbidimeter. The IF-0a with Biolog Redox Dye Mix (A for E. coli or F for Ec and Bs ) (1:5 dilution) was prepared by adding 0.9 mL of Dye A or F to 62.5 mL of IF-0a and completed the volume to 75 mL by sterile distilled water. To prepare the bacterial cell suspension, 15 mL of IF-0a with bacteria was added to 75 mL of IF-0a with Dye Mix A or F; the cell density must be 80-85% T. Later, 22 mL of this 85% T suspension were transferred to a sterile reservoir. To prepare the treated group bacteria (bacteria treated by ethanolic extract), the same previous steps were followed except the addition of ethanolic extract at different concentrations (0.001-0.1 mg/mL) instead of distilled water in IF-0a plus Dye Mix A or F. In order to prepare the bacteria cell suspension, colonies were harvested from the surface of an agar plate with a sterile cotton wool swab and suspended in 16 mL of inoculating Tryptic Soy Broth (TSB), in a 20 mL sterile capped glass tube. The cell density must be equaled to 80-85% transmittance (T) on a Biolog turbidimeter. The TSB with Biolog Redox Dye Mix (A for E. coli or F for Ec and Bs) was also prepared.
Later, 250 μL/well using Biolog multichannel pipette were added to phenotype microarrays plates as 100 μL/well bacteria cell suspension + 130 μL/well TSB with Biolog Redox Dye Mix correspondent + 20 μL/well of ethanolic extract (at different concentration 0.001-0.1 mg/mL). All the plates were incubated at 37°C in the OmniLog plate incubator and reader and were monitored for any color change of the wells. Readings were recorded for 48 hours for all phenotype microarrays plates.
phenotype microarrays of compounds with fungi
Alternaria alternate and Fusarium solani were grown on nutrient agar plates overnight at 30°C. In order to prepare the control group fungus (untreated fungus), colonies were harvested from the surface of an agar plate with a sterile cotton wool swab and suspended in 16 mL of inoculating Fluid-0a (IF-0a), in a 20 mL sterile capped glass tube. The cell density must be equaled to 62% transmittance (T) on a Biolog turbidimeter. In order to prepare the fungus cell suspension, colonies were harvested from the surface of an agar plate with a sterile cotton wool swab and suspended in 16 mL of inoculating Malt extract (ME), in a 20 mL sterile capped glass tube. The cell density must be equaled to 62% transmittance (T) on a Biolog turbidimeter. The Malt extract with Biolog Redox Dye Mix F was also prepared.
Later, 250 μL/well using Biolog multichannel pipette were added to phenotype microarrays plates as 20 μL/well fungus cell suspension + 210 μL/well Malt extract with Biolog Redox Dye Mix F + 20 μL/well ethanolic extract (at different concentration 0.001-0.1 mg/mL). All the plates were incubated at 37°C in the OmniLog plate incubator and reader and were monitored for any color change of the wells. Readings were recorded for 48 hours for all phenotype microarrays plates.
Antifungal activity with disc-methods
Ethanolic residue was dissolved in sterilized distilled water. Four plants pathogenic fungi were used for this test namely F. oxysporum f. sp. melonis, F. oxysporum f. sp. lycopersici, Botrytis cinerea, and F. sambucinum. Targeted fungi were grown on Potato Dextrose Agar (PDA) medium amended with adequate volumes of M. azedarach extracts. Concentrations tested were 0.04, 0.06, 0.08 and 0.1% for ethanolic extract. Agar plugs (6 mm in diameter) removed from a fast growing fungal colony was plated in 9 cm petri dishes amended or not with the extracts tested (three plugs per plate). The colony diameter was noted after 3 days of incubation at 25°C. Control received the same volume of sterilized distilled water.
Fungal growth was measured by averaging the three diameters taken at right angles for each colony. Percentage growth inhibition (%) of fungal colonies was calculated according to the following formula (Jabeen and Javaid, 2008):
Growth inhibition (%) = [(Growth in control - Growth in treatment) /Growth in control] × 100
Anti-inflammatory activity
Anti-inflammatory activity of M. azedarach ethanolic leaves extract and indomethacin, as standard drug, was determined by carrageenan-induced hind paw edema (Winter et al., 1962). Extract at 150 mg/kg dose (Jain et al., 2015) and indomethacin at 25 mg/kg dose were given to rats intraperitoneally. The same volume of ethanol 10% used as vehicle was given to the control group. One hour after treatment, carrageenan 0.1 mL (1%, w/v) solution in ultrapure water was subcutaneously injected into the planter surface of the right hind paw of all rats. The paw volume was measured with a plethysmometer before injection of carrageenan. Carrageenan-induced paw edema was measured at 30, 60 and 120 min. Anti-inflammatory activities of M. azedarach leaves ethanolic extract and indomethacin were determined by comparing their results with the ones obtained in the control group. The anti-inflammatory effect of compounds was calculated by the following equation:
Anti-inflammatory activity (I %) = (1 − D/C) × 100
where D represents the percentage difference in paw volume after extract or drug administration to the rats, and C represents the percentage difference of volume in the control group.
Results
Antifungal and antibacterial activity
M.azedarach ethanolic leaves extract had shown an important antibacterial and antifungal activities with Biolog phenotype microarray technology against Enterococcus faecalis, Bacillus subtilis, Escherichia Coli, Alternaria alternata and Fusarium solani. Results showed a high antibacterial activity for M. azedarach leaves extract (IC50) compared to absolute ethanol: E. coli 6.6 µg/mL vs. 10.7%, Enterococcus faecalis 1 µg/mL vs. 61.0 % and Bacillus subtilis 1.3 µg/mL vs. 44.9%. While antifungal activity of M. azedarach ethanolic leaves extract compared with absolute ethanol was as followed Alternaria: 1.0 µg/mL vs. 32.5% and Fusarium 53 µg/mL vs. 37.8% (Figure 1, Table I).
Figure 1: Photo of antibacterial and antifungal assays of M. azedarach leaves extract with Biolog phenotype microarray technology
Table I: Antibacterial and antifungal assays of M. azedarach extract with phenotype microarray technology
Ethanolic extract (IC50 µg/mL) |
Ethanol (95%) I% |
|
---|---|---|
E. coli | 6.6 | 10.7 |
Enterococcus f | 1.0 | 61.0 |
Bacillus S. | 1.3 | 44.9 |
Fusarium S. | 53.9 | 37.8 |
Alternaria A. | 1 | 32.5 |
With disc-methods, M. azedarach ethanolic leaves extracts exhibited a significant (p≤5%) inhibitory effects on growth of tested fungal pathogens. In fact, 0.1% concentration of M. azedarach ethanolic leaves extract was been the highest concentration that gave a mycelium growth inhibition at around 8%, 19%, 36% and 38% respectively against F. oxysporum f. sp. melonis, F. sambucinum, F. oxysporum f. sp. lycopersici and B cinerea (Figure 2). The remaining concentrations exhibited an antifungal activities (Table II). Eventually, F. oxysporum f. sp. melonis has the greatest resistance toward M. azedarach leaves extract at different concentrations. Therefore, among fungal plant pathogens tested, F. oxysporum f. sp. lycopersici and B. cinerea were found to be more sensitive to M. azedarach leaf ethanolic extract than F. oxysporum f. sp. melonis and F. sambucinum.
Table II: Antifungal assays of M. azedarach leaves extract with disc method
Fungi | M. azedarach ethanolic extract concentration (%) | |||
---|---|---|---|---|
0.02 | 0.04 | 0.06 | 0.1 | |
Inhibition (%) | ||||
F.O. melonis | 3.9 ± 0.2 | 5.2 ± 0.2 | 6.1 ± 0.3 | 7.8 ± 0.4 |
F. sambucinum | 6.4 ± 0.3 | 7.2 ± 0.3 | 14 ± 0.7 | 19.1 ± 0.9 |
F. O. Lycopercisi | 11.2 ± 0.6 | 26.4 ± 1.3 | 30.5 ± 1.5 | 36.1 ± 1.8 |
Botrytis cinerea | 31.1 ± 1.5 | 33.3 ± 1.7 | 33.0 ± 1.6 | 37.7 ± 1.9 |
Data are mean ± SEM |
Figure 2: Antifungal activity of Melia azeadarch ethanolic leaves extract. A: Antifungal activity against FO. melonis, F. sambucinum, FO. Lycopersici and B. cinerea with disc-methods at the concentrations 0.04, 0.06, 0.08 and 0.1%; B: Photo of antifungal assays of M. azedarach leaves extract with disc method
Anti-inflammatory activity
M. azedarach extract was screened, for their anti-inflammatory activity by carrageenan-induced hind paw edema, using female Wister rats. The investigated extract showed a good inhibition of the carrageenan-induced paw edema evolution in comparison with indomethacin as standard drug. It was found that M. azedarach extract at a dose of 150 mg/kg demonstrate a highest anti-inflammatory activity at around 25% when compared with indomethacin at 32% at 10 mg/kg after 2 hours of treatment (Figure 3).
Figure 3: Anti-inflammatory effect of Melia azedarach leaves extract with carrageenan induced paw edema. A: Inhibition of the carrageenan-induced paw edema evolution during the treatment; B: percentage of anti-inflammatory activity at the end of treatment
Discussion
M.azedarach extract had shown important antibacterial activities against Enterococcus faecalis and Bacillus subtilis (Bs). Results could be due to the presence of phenolic compounds and flavonoids previously characterized (Akacha et al., 2016). The slight effect on E. coli bacteria is probably attributed to the higher resistance of E. coli (ATCC 8739) to many plant extracts (Oskay et al., 2009). The major component in the studied extract, which is the protocatechuic acid (one of metabolites of quercetin) had shown antibacterial effects in ground beef and apple juice (Chao and Yin, 2009). Similarly, Aziz et al. (1998) had proved that protocatechuic acid, among other phenolics, inhibited the growth of E. coli, Klebsiella pneumoniae, Bacillus cereus, Aspergillus flavus and Aspergillus parasiticus. Besides, isoquercitrin; second predominant component in studied ethanolic extract possess fungicidal action exerted by disturbing the membrane of cells as investigated by Yun et al. (2015).
Other phenolics present- even in lower doses- in our extract could contribute to the observed fungicidal and microbial effects such as gallic acid which had been proven to possess bactericidal and antifungal activity against F. solani (Nguyen et al., 2015) and Candida species (Alves et al., 2014). Therefore, syringic acid-known to have antimicrobial activity and fungitoxicity- as proven against Gano derma boninense by Chong et al. (2012) could result in observed antimicrobial effects. Similarly, chlorogenic acid also developed antifungal (Vandal et al., 2015) and antimicrobial effects on both Gram-positive and Gram-negative bacteria (Suárez-Quiroz et al., 2013).
Previous GC-MS analysis of the ethanolic extract revealed the presence of mainly stearic, palmitic, palmitoleic, then oleic and linoleic fatty acids. Indeed, stearic, palmitic, linoleic and oleic acids are known to be potential antibacterial and antifungal agents (McGaw et al., 2002; Seidel and Taylor, 2004). Moreover, oleic acid was reported to possess antimicrobial activity against human pathogens (Kabara et al., 1972). Furthermore, the antifungal activities exhibited by linolenic, linoleic and oleic acids were investigated by Walters et al. (2004). Also, the antibacterial activity of linoleic and oleic acids isolated from Helichrysum pedunculatum was previously demonstrated by Dilika et al. (2000) as well as synergistic effect between the two fatty acids. A further, palmitoleic acid exhibited the greatest antibacterial activity against meat spoilage organisms according to a study conducted by Ouattara et al. (1997).
In the current study, F. oxysporum f. sp. Lycopersici and B. cinerea were found to be more sensitive to M. azedarach leaves extracts than F. oxysporum f. sp. melonis. This result is interesting since B. cinerea is a necrotrophic fungal pathogen that attacks over 200 different plant species (Elad, 1997) and has became resistant to chemical pesticides (Bardas et al., 2010).
Our results are in line with the findings of some earlier workers reporting antibacterial/antifungal effects of M. azedarach alcoholic leaves extracts against many fungal species such as Ascochyta rabiei (Jabeen et al. 2011), Aspergillus flavus, Diaporthe phaseolorum var. meridionales, F. oxysporum, F. solani, F. verticillioides, and Sclerotinia sclerotiorum (Carpinella et al., 2003). More recently, Neycee et al. (2012) had shown that M. azedarach leaf extract effect on Sclerotium spp., F. oxysporum, and Rhizoctonia solani were not significant. However, Sen and Batra (2012) stated that M. azedarach leaf extracts were effective against some bacteria such as B. cereus, S. aureus, E. coli, Pseudomonas aeruginosa, and fungi namely Aspergillus niger, A. flavus, F. oxysporum, and Rhizopus stolonifer.
These differences in bioactivities of M. azedarach extracts between reports could result from the differences in chemical composition between species naturalized in different regions (Gottlieb et al., 2001; Szewczuk et al., 2003; Orhan et al., 2012). Based on phytochemical investigation of Tunisian M. azedarach leaves performed in previous work (Akacha et al., 2016) and taking into account the chemical profile, we can speculate that observed bactericide/antifungal effects may result partly from the action of chlorogenic acids as previously shown by Ling et al. (2013). In fact, chlorogenic and caffeic acids exhibit inhibitory effects against conidial germination and growth of F. oxysporum f. sp. niveum where the target pathogen is found to be much more susceptible to chlorogenic acid than to caffeic acid. Furthermore, protocatechic acid as shown by Nguyen et al. (2015) to display potent antifungal activity against B. cinerea and R. solani and is considered as a promising alternative to chemical fungicides for B. cinerea control in strawberry crops.
Besides, observed antifungal potency may be due to the presence of linoleic in the extract (Abdelillah et al., 2013).
Ethanolic M. azedarach leaves extract had shown high anti-inflammatory effect. This result corroborates with the findings of previous workers who investigate anti-inflammatory activity of M. azedarach flowers (Sumathi et al., 2014), roots (Vishnukanta, 2010) and seeds (Khadse and Kakde, 2014).
Besides, Aouadia et al. (2013) proved that M. azedarach water and aqueous ethanol (50%) leaf extracts exerted strong anti-inflammatory effects by inhibiting human monoacylglycerol lipase.
Observed anti-inflammatory effect might result of elementary and/or synergic action of present components in M. azedarach leaves extract such as gallic acid. In fact, the latter was found to possess anti-inflammatory activity towards zymosan-induced acute food pad swelling in mice (Kroes et al., 1992) and using human basophils (KU812 cells) which are crucial effector cells in allergic inflammation. Kim et al. (2006) explain this capacity by the aptitude of gallic acid to inhibit histamine release and pro-inflammatory cytokine production in mast cells. A further, structure-activity relationship analysis showed that the o-dihydroxy group of gallic acid is important for the inhibitory activity in vitro (Kroes et al., 1992). Also, catechins possess anti-inflammatory effects (Reto et al., 2014) as well as chlorogenic acid (dos Santos et al., 2006; Shin et al., 2015). The latter significantly inhibited not only nitric oxide production but also the expression of inducible nitric oxide synthase (iNOS), cyclo-oxygenase-2 (COX-2). Chlorogenic acid also attenuated pro-inflammatory cytokines and other inflammation-related markers such as interleukin-6 in a dose-dependent manner (Hwang et al., 2014).
Similarly, sinapic acid has anti-inflammatory activity by eventual suppression of the expressions of iNOS and COX-2, tumor necrosis factor (TNF)-alpha, and interleukin (IL)-1beta and this via nuclear factor-kappa B (NF-kappaB) inactivation (Yun et al., 2008). Furthermore, syringic acid possesses anti-obesity, anti-inflammatory and anti-steatotic effects via the regulation of lipid metabolic and inflammatory genes (Ham et al., 2016). Also, protocatechic acid has shown anti-inflammatory effect (Lin et al., 2009). Treatment with protocatechic acid inhibits significantly different biological parameters like hind paw edema, granuloma exudates formation, and arthritis index in carrageenan edema, cotton pellet granuloma, and Freund’s adjuvant arthritis, respectively. The biochemical parameters like glutathione, superoxide dismutase, catalase, lipid peroxidation and nitric oxide in edematous occurring during different types of inflammation were either significantly restored or inhibited with protocatechic acid pretreatment (Lende et al., 2011). In addition, isoquercitrin anti-inflammatory effect was investigated in a murine model of asthma. It was an effective eosinophilic inflammation suppressors, suggesting a potential for treating allergies (Rogerio et al., 2007). Finally, isorhamnetin was proved to have anti-inflammatory properties (Boesch-Saadatmandi et al., 2011; Chirumbolo, 2014).
Conclusion
This work provide scientific evidence for the traditional uses of M. azedarach as anti-inflammatory, antibacterial and antifungal agents.
References
Abdelillah A, Houcine B, Halima D, Meriem Cs, Imane Z, Eddine SD, Abdallah M, Daoudi Cs. Evaluation of antifungal activity of free fatty acids methyl esters fraction isolated from Algerian Linum usitatissimum L. seeds against toxigenic Aspergillus. Asian Pac J Trop Biomed. 2013; 3: 443-48.
Akacha M, Lahbib K, Ghanem Boughanmi N. Phytochemically evaluation and net antioxidant activity of Melia azedarach. L leaves extracts from their ProAntidex parameter. Bangladesh J Pharmacol. 2016; 11: 301-07.
Aktar Md W, Sengupta D, Chowdhury A. Impact of pesticides use in agriculture: Their benefits and hazards. Interdiscip Toxicol. 2009; 2: 1–12.
Alves CT, Ferreira IC, Barros L, Silva S, Azeredo J, Henriques M. Antifungal activity of phenolic compounds identified in flowers from North Eastern Portugal against Candida species. Future Microbiol. 2014; 9: 139–46.
Aoudia H, Oomah BD, Zaidi F, Zaidi-Yahiaoui R, Drover JCG, Harrison JE. Phenolics, antioxidant and anti-inflammatory activities of Melia azedarach extracts. IJARNP. 2013; 6: 2: 19-29.
Aziz NH, Farag SE, Mousa LA, et al. Comparative antibacterial and antifungal effects of some phenolic compounds. Microbios. 1998;93:43–54.
Bardas GA, Velouka T, Koutita O, Karaoglanidis GS. Multiple resistance of Botrytis cinerea from kiwifruit to SDHIs, QoIs and fungicides of other chemical groups. Pest Manag Sci. 2010; 66: 967–73.
Bochner BR, Gadzinski P, Panomitros E. Phenotype micro-arrays for high-throughput phenotypic testing and assay of gene function. Genome Res. 2001; 11: 1246-55.
Bochner BR. New technologies to assess genotype-phenotype relationships. Nat Rev Genet. 2003; 4: 309-14.
Boesch-Saadatmandi C, Loboda A, Wagner AE, Stachurska A, Jozkowicz A, Dulak J, Döring F, Wolffram S, Rimbach G. Effect of quercetin and its metabolites isorhamnetin and quercetin-3-glucuronide on inflammatory gene expression: Role of miR-155. J Nutr Biochem. 2011; 22: 293-99.
Canli K, Altuner EM, Akata I. Antimicrobial screening of Mnium stellar. Bangladesh J Pharmacol. 2015; 10: 321-25.
Carpinella MC, Giorda LM, Ferrayoli CG, Ferrayoli CG. Antifungal effects of different organic extracts from Melia azedarach L., on phytopathogenic fungi and their isolated active components. J Agric Food Chem. 2003; 51: 2506-11.
Chao CY, Yin MC. Antibacterial effects of roselle calyx extracts and protocatechuic acid in ground beef and apple juice. Foodborne Pathog Dis. 2009; 6: 201–06.
Chirumbolo S. Anti-inflammatory action of isorhamnetin. Inflammation 2014; 37, 4: 1200-01.
Chong KP, Atong M, Rossall S. The role of syringic acid in the interaction between oil palm and Ganoderma boninense, the causal agent of basal stem rot. Plant Pathol. 2012; 61: 953–63.
Dissanayake MLMC. Inhibitory effect of selected medicinal plant extracts on phytopathogenic fungu Fusarium oxysporum (Nectriaceae) Schlecht. Emend. Snyder and Hansen. Annu Res Rev Biol. 2014; 4: 133-42.
Dilika F, Bremner PD, Meyer JJM. Antibacterial activity of linoleic and oleic acids isolated from Helichrysum pedunculatum: A plant used during circumcision rites. Fitoterapia 2000; 71: 450-52.
dos Santos MD, Almeida MC, NP Lopes, GEP de Souza. Evaluation of the anti-inflammatory, analgesic and antipyretic activities of the natural polyphenol chlorogenic acid. Biol Pharm Bull. 2006; 29: 2236-40.
Elad Y. Responses of plants to infection by Botrytis cinerea. Plant J. 1997; 20: 485-92.
Fleming RV, Walsh TJ, Anaissie EJ. Emerging and less common fungal pathogens. Infect Dis Clin N Am. 2002; 16: 915–33.
Gopal V, Prakash Yoganandam G, Manju P. A concise review on melia dubia Cav. (Meliaceae). Euro J Environ Ecol. 2015; 2: 57-60.
Gottlieb OR, Kaplan MA, Sorin MR de MB. Biodiversidad: Un enfoque integrado entre la QuÃmica y la BiologÃa. Spanish version by Pomilio AB. Buenos Aires, Idecefyn, 2001.
Ham JR, Lee HI, Choi RY, Sim MO, Seo KI, Lee MK. Anti-steatotic and anti-inflammatory roles of syringic acid in high-fat diet-induced obese mice. Food Funct. 2016; 7: 689-97.
Hwang SJ, Kim YW, Park Y, Lee HJ, Kim KW. Anti-inflammatory effects of chlorogenic acid in lipopolysaccharide-stimulated RAW 264.7 cells. Inflamm Res. 2014; 63: 81-90.
Jain A, Jain S, Rawat S. Emerging fungal infections among children: A review on its clinical manifestations, diagnosis, and prevention. J Pharm Bioallied Sci. 2010; 2: 314–20.
Jain G, Pandit D, Gupta P, Jharia V. Evaluation of anti-inflammatory activity of seeds of M. azedarach (Linn.) in albino Wistar rats. Int J Sci Eng Res. 2015; 6: 1837-46.
Jabeen K, Javaid A, Ahmad E, Athar M. Antifungal compounds from Melia azedarach leaves for management of Ascochyta rabiei, the cause of chickpea blight. Nat Prod Res. 2011; 25: 264-76.
Jabeen K, Javaid A. Antifungal activity of aqueous and organic solvent extracts of allelopathic trees against Ascochyta rabiei. Allelopathy J. 2008; 22: 231-38.
Kabara JJ, Swieczkowski DM, Conley AJ, Truant JP. Fatty acids and derivatives as antimicrobial agents. Antimicrob Agents Chemother. 1972; 2: 23-28.
Khadse CD, Kakde RB. Anti-inflammatory activities of aqueous extract of fruits and their different fractions of Melia dubia. Res J Pharm Biol Chem Sci. 2014; 5: 780.
Kim SH, Jun CD, Suk K, Choi BJ, Lim H, Park S, Lee SH, Shin HY, Kim DK, Shin TY. Gallic acid inhibits histamine release and pro-inflammatory cytokine production in mast cells. Toxicol Sci. 2006; 91: 123-31.
Kroes BH, van den Berg A J, Quarles van Ufford HC, van Dijk H, Labadie RP. Anti-inflammatory activity of gallic acid. Planta Med. 1992; 58: 499–504.
Lende AB, Kshirsagar AD, Deshpande AD, Muley MM, Patil RR, Bafna PA, Naik SR. Anti-inflammatory and analgesic activity of protocatechuic acid in rats and mice. Inflammopharmacology 2011; 19: 255–63.
Li RW, Myers SP, Leach DN, Lin GD, Leach G. A cross-sectional study: Anti-inflammatory activity of Australian and Chinese plants. J Ethnopharmacol. 2003; 85: 25–32.
Lin CY, Huang CS, Huang CY, Yin MC. Anti-coagulatory, anti-inflammatory, and antioxidative effects of protocatechuic acid in diabetic mice. J Agric Food Chem. 2009; 12; 57: 6661-67.
Ling N, Zhang W, Wang D, Mao J, Huang Q, Guo S, Shen Q. Root exudates from grafted-root watermelon showed a certain contribution in inhibiting Fusarium oxysporum f. sp. niveum. PLoS One. 2013; 8: e63383.
McGaw LJ, Jäger AK, van Staden J. Antibacterial effects of fatty acids and related compounds from plants. S Afr J Bot. 2002; 68: 417-23.
Murtaza G, Mukhtar M, Sarfraz A. A review: Antifungal potentials of medicinal plants. J Bioresource Manage. 2015; 2: 23-31.
Nascimento GGF, Locatelli J, Freitas PC, GL Silva. Antibacterial activity of plant extracts and phytochemicals on antibiotic-resistant bacteria. Braz J Microbiol. 2000; 31: 247-56.
Neycee MA, Nematzadeh GHA, Dehestani A, Alavi M. Assessment of antifungal effects of shoot extracts in chinaberry (Melia azedarach) against 5 phytopathogenic fungi. Int J Agric Crop Sci. 2012; 4: 474–77.
Nguyen XH, Naing KW, Lee YS, Moon JH, Lee JH, Kim KY. Isolation and characteristics of protocatechuic acid from Paenibacillus elgii HOA73 against Botrytis cinerea on strawberry fruits. J Basic Microbiol. 2015; 55: 625-34.
Orhan IE, Guner E, Ozturk N, Senol FS, Erdem SA, Kartal M, Sener B. Enzyme inhibitory and antioxidant activity of Melia azedarach L. naturalized in Anatolia and its phenolic acid and fatty acid composition. Ind Crops Prod. 2012; 37: 213-18.
Oskay M, Oskay D, Kalyoncu F. Activity of some plant extracts against multi-drug resistant human pathogens. Iranian J Pharmacol Res. 2009; 8: 293–300.
Ouattara B, Simard RE, Holley RA, Piette GJP, Begin A. Antibacterial activity of selected fatty acids and essential oils against six meat spoilage organisms. Int J Food Microbiol. 1997; 37:155-62.
Rajkumar K, Malathi R. Phytochemical investigation GC-MS analysis and in vitro antimicrobial activity of Coleus forskohlii. Bangladesh J Pharmacol. 2015; 10: 924-30.
Reto, M, Almeida C, Rocha J, Sepodes B. Figueira ME. Green Tea (Camellia sinensis): Hypocholesterolemic effects in humans and anti-inflammatory efects in animals. Food Nut Sci. 2014; 5: 2185-94.
Rogerio AP, Kanashiro A, Fontanari C, da Silva EV, Lucisano-Valim YM, Soares EG, Faccioli LH. Anti-inflammatory activity of quercetin and isoquercitrin in experimental murine allergic asthma. Inflamm Res. 2007; 56: 402-08.
Sen A, Batra A. Evaluation of antimicrobial activity of different solvent extracts of medicinal plant: Melia azedarach L. Int J Curr Pharm Res. 2012; 4: 67-73.
Seidel V, Taylor PW. In vitro activity of extracts and constituents of Pelargonium against rapidly growing mycobacteria. Int J Antimicrob Agents. 2004; 23: 613-19.
Shin HS, Satsu H, Bae MJ, Zhao Z, Ogiwara H, Totsuka M, Shimizu M. Anti-inflammatory effect of chlorogenic acid on the IL-8 production in Caco-2 cells and the dextran sulphate sodium-induced colitis symptoms in C57BL/6 mice. Food Chem. 2015; 168: 167-75.
Suárez-Quiroz, ML, Alonso Campos A, Alfaro V, Gonzáles-Rios O, Villeneuve P, Figueroa-Espinoza MC. Anti-Aspergi-llus activity of green coffee 5-O-caffeoyl quinic acid and its alkyl esters. Microb Pathog. 2013; 61–62: 51–56.
Sumathi S, Selvi M, Sinthanai. Phytochemical studies and in vitro Anti-inflammatory activity of Melia azedarach (L) flower. Res J Pharmacogn Phytochem. 2014; 6: 19-21.
Szewczuk VD., Mongelli ER, Pomilio AB. Antiparasitic activity of Melia azedarach growing in Argentina. Mol Med Chem. 2003; 1: 54-57.
Tsair-Bor Y, Shang-Tzen C. Synergistic effects of cinnamaldehyde in combination with eugenol against wood decay fungi. Biores Technol. 2008; 99: 232-36.
Vandal J, Abou-Zaid MM, Ferroni G, Leduc LG. Antimicrobial activity of natural products from the flora of Northern Ontario, Canada. Pharm Biol. 2015; 53: 800-06.
Vishnukanta RAC. Evaluation of hydroalcoholic extract of Melia azedarach Linn roots for analgesic and anti-inflammatory activity. Int J Phytomed. 2010; 2: 341-44.
Walters D, Raynor L, Mitchell A, Walker R, Walker K. Antifungal activities of four fatty acids against plant pathogenic fungi. Mycopathology 2004; 157: 87–90.
Winter CA, Risley EA, Nuss GW. Carrageenin-induced edema in hind paw of the rat as an assay for anti-inflammatory drugs. Proc Soc Exp Biol Med. 1962; 111: 544-47.
Yun KJ, Koh DJ, Kim SH, Park SJ, Ryu JH, Kim DG, Lee JY, Lee KT. Anti-inflammatory effects of sinapic acid through the suppression of inducible nitric oxide synthase, cyclooxygase-2, and proinflammatory cytokines expressions via nuclear factor-kappa B inactivation. J Agric Food Chem. 2008; 56: 10265-72.
Yun J, Lee HJ, Ko HJ, Woo ER, Lee DG. Fungicidal effect of isoquercitrin via inducing membrane disturbance. BBA-Biomembranes. 2015; 1848: 695–701.
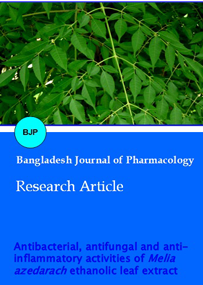