Artemisinic acid exhibits antitumor activity in MCF-7 breast cancer cells through the inhibition of angiogenesis, VEGF, m-TOR and AKT signalling pathways
Abstract
The aim of the present study was to evaluate the antitumor and anti-angiogenic effects of artemisinic acid in MCF-7 human breast cancer cells. Various cell signalling pathways (VEGF, m-TOR and AKT signalling pathways) and MTT assay were used. The in vivo antitumor activity of artemisinic acid was evaluated by means of tumor xenograft mouse model. Transwell cell migration assay was used to examine the chemotactic motility of the human umbilical vascular endothelial cells (HUVECs), while as endothelial cell capillary-like tube formation assay was used to evaluate the effect of artemisinic acid on the tube formation in HUVECs. We found that artemisinic acid considerably reduced both the volume and weight of concrete tumors and reduced angiogenesis in a xenograft mouse tumor model in vivo. Further, artemisinic acid suppressed the VEGF-induced cell migration and capillary-like tube formation of HUVECs in a dose-dependent manner. Artemisinic acid was found to suppress the VEGF-induced phosphorylation of VEGFR2 and also the activity of AKT and m-TOR.
Introduction
Tumor angiogenesis involves the growth and development of new blood vessels from already existing ones. Angiogenesis has a critical role to play in tumor growth and development, metastasis and invasiveness. The process of angiogenesis under normal circumstances is very crucial in growth and development, wound healing as well as in the formation of granulation tissues. However, this process also helps in transforming benign tumors into malignant ones thus contributing to more than 90% of all cancer deaths (Compagni and Christofori, 2000; Ferrara and Kerbel, 2005; Quesada et al., 2006).
As such it is believed that tumor angiogenesis is a probable therapeutic goal for cancer treatment. Therefore, most of the cancer research currently involves discovery, design and development of the anti-angiogenic agents which are safe and easily affordable (Kerbel, 2008). Cell proliferation and cell migration help in initiating the angiogenesis process.
Vascular endothelial growth factor (VEGF) is uttered in most cancers and plays a vital role in tumor angiogenesis by exerting its effects through binding to its receptor tyrosine kinase (Carmeliet, 2005; Kowanetz and Ferrara, 2006; Pyun et al., 2008). VEGF signalling concerned with angiogenesis is primarily intervened through VEGF receptor 2 (VEGFR2) activation leading to triggering of a range of intracellular signalling molecules including phosphoinositide 3-kinase (PI3K)/AKT kinase, mammalian target of rapamycin (mTOR)/ribosomal protein S6 kinase, Src family kinase etc (Falcon et al., 2011; Schlessinger, 2000; Liu et al., 2008). Consequently, VEGFR2 and these intracellular signalling molecules appear to be significant targets for the suppression of tumor angiogenesis.
The main motive of the present research work was to evaluate the antitumor and anti-angiogenesis property of artemisinic acid in MCF-7 human breast cancer cells and also express the role of various signalling pathways including VEGF, m-TOR and AKT signalling pathways.
Materials and Methods
Chemicals, other reagents and antibodies
Artemisinic acid (purity >98%) and 3-(4, 5-dimethylthiazol-2-yl)-2,5-diphenyltetrazolium-bromide (MTT) were obtained from Sigma-Aldrich (USA). A 20 mM solution of it was stored at -20ºC in small aliquots until required. These aliquots were protected from light and were diluted to the obligatory concentrations in cell culture medium. Bacteria-derived recombinant human VEGF (VEGF) and growth factor-reduced matrigel were purchased from Shanghai Tauto Biotech (China). The antibodies against beta-actin, AKT, VEGFR-2, mTOR, PI3K were purchased from Santa Cruz Biotechnology (USA).
Cell lines and culture conditions
Human breast cancer cell line (MCF-7) was obtained from the Shanghai Institute of Cell Resource Center of Life Science (China). The cells were cultured in Dulbecco's Modified Eagle's Medium supplemented with 10% (v/v) fetal bovine serum (FBS) under humidified atmosphere of 5% CO2 at 37ºC. Primary human umbilical vascular endothelial cells (HUVEC) were purchased from Sciencell (Carlsbad, USA) and cultured in endothelial cell growth medium (ECGM).
MTT assay of cell viability
The cells were cultured in Dulbecco's Modified Eagle's Medium supplemented with 10% (v/v) fetal bovine serum (FBS) under humidified atmosphere of 5% CO2 at 37oC. The medium was replaced every 3 days. Cells were subcultured every 4 days. The cells were seeded on a 96-well plate at 2 x 105 cells per well. After 24 hours, artemisinic acid was dissolved in DMSO at a variety of concentrations (0, 5, 10, 25, 50, 100 and 150 µM) before treated the cells with it. After incubation of 24 and 48 hours times, MTT solution was added. The number of viable cells is equal to the formation of formazan crystals which were dissolved in ethanol and the optical density was measured on a microplate reader (ELX 800; Bio-tek Instruments, Inc., USA) at a wavelength of 490 nm.
Tumor xenograft mouse model of human breast cancer cells
Eight-week-old female BALB/c nude mice weighing around 30 g (National Rodent Laboratory Animal Resources, China) were maintained in a laminar airflow cupboard under pathogen-free conditions and a 12-hours light-dark cycle.
The mice were arbitrarily divided into two groups containing five each. MCF-7 cells were injected subcutaneously into the mice (2 x 105 cells per mouse). The mice were injected with either artemisinic acid or vehicle (5 mg/kg, and 10 mg/kg) for 21 days after tumors growth was observed to be about 100 mm3. The tumor size and volume were measured with a caliper as follows:
Calculated size = Shortest diameter2 x longest diameter/2
Calculated volume = Shortest diameter2 x longest diameter/2
The body weight of mouse, tumor size and volume were measured at two day intervals.
Transwell migration assay
Transwell migration assay was used to evaluate the chemotactic motility of the HUVECs (Pang et al., 2010). HUVECs were allowed to grow to full convergence in 6-well plate. The assay was done in such a way so that the bottom chambers were filled with 500 uL of endothelial cell growth medium (ECGM) containing 1.5% FBS complemented with 20 ng/mL VEGF. The top chambers were filled with HUVECs suspended in 200 uL of ECGM plus different concentrations of artemisinic acid. After 4 hours migration period, the migrated cells were fixed with 5% cold paraformaldehyde and then stained with 1.5% crystal violet. The migrated cells were counted manually and the percentage of inhibition was shown in contrast to the untreated wells at 100%.
Endothelial cell capillary-like tube formation assay
This assay was done as described before (Pisanti et al., 2011). Growth factor-reduced matrigel (30 μL Matrigel/well) was transferred to 96-well plate and polymerized for 50 min at 37°C. HUVECs were initially incubated in ECGM containing 1.5% FBS for 4 hours, then these were subjected different doses of artemisinic acid (0, 10, 50 and 100 µM) for 60 min and finally seeded onto the matrigel layer in 96-well plates at a density of 2 x105 cells/well. Serum-free ECGM containing 1.5% FBS was added with or without 5 ng/mL VEGF. Photographs of tubular structures of the endothelial cells were captured by using an inverted light microscope (Olympus; magnification, 100x).
Western blot analysis
Next we involved Western blot assay to illustrate the main proteins involved in the biofunctions of endothelial cells. HUVECs were first starved in serum-free ECGM for 4 hours and were pretreated without or with artemisinic acid (0, 10, 50 and 100 µM) for 40 min. Initially the gel was cast (molded) according to the size of the protein. Then the loading of gel was done in appropriate buffer which is usually running buffer. The gel was set at a particular voltage usually 70 V to give better resolution. Then the gel was transferred to PDVF membrane. The membrane was blocked by using either milk or BSA for 1-2 hours. Then the membrane was incubated overnight with primary antibody of interest. The membranes were washed three times for 5 min after primary incubation and then again incubating them with HRP labelled secondary antibody. The membranes were washed again after secondary incubation three times and then these membranes were developed with luminol on X-ray.
Statistical analysis
Data is presented as the mean ± SEM of the control. All of the experiments were repeated at least three times. The differences between groups were analyzed by one-way ANOVA with Tukey's post hoc tests, significance of difference was indicated as p<0.05, p<0.01.
Results
In vitro and in vivo tumor angiogenesis in cellular and xenograft mouse models
The in vitro antitumor effects of artemisinic acid on MCF-7 human breast cancer cells was initially assessed by MTT cell viability assay which indicated that artemisinic acid induced both dose-dependent as well as time-dependent antitumor effects in these cells. Figure 1A shows the chemical structure of artemisinic acid while as Figure 1B shows the antitumor effects of artemisinic acid in MCF-7 cancer cells. In order to observe the effects of artemisinic acid on tumor angiogenesis and tumor growth in vivo, we used a xenograft human breast cancer model. The in vivo results showed that 5 mg/kg of artemisinic acid used every other day suppressed both tumor weight and tumor volume in a dose-dependent manner (Figure 2). The results revealed that 5 mg/kg and 10 mg/kg artemisinic acid injection reduced the tumor weight from 1.3 g in PBS-treated group (control) to 0.6 g and 0.3 g respectively. Similarly, 5 mg/kg and 10 mg/kg artemisinic acid injection reduced the tumor volume from 1.5 cm3 in PBS-treated group (control) to 0.9 cm3 and 0.32 cm3 respectively. This indicates that artemisinic acid considerably suppressed tumor growth in the xenograft mouse breast cancer model.
Figure 1: A) Chemical structure of artemisinic acid. B) Cytotoxic effect of artemisinic acid in human breast cancer cells (MCF-7). Data are shown as the mean ± SD of three independent experiments. ap<0.05, bp<0.01, vs 0 µM (control)
Figure 2: Effect of artemisinic acid on tumor growth in a mouse xenograft model. Images of cancerous tissues removed from the mice in control and drug-treated groups
Inhibition of the VEGF-induced chemotactic migration
One of the key steps in blood vessel formation during tumor angiogenesis is the migration of endothelial cells. In the current study, transwell cell migration assay was used to assess the effect of artemisinic acid on the chemotactic motility of HUVECs. It can be seen from Figure 3a, artemisinic acid considerably inhibited the migration of HUVEC cells. Artemisinic acid decreased the VEGF-induced HUVEC chemotactic motility in a dose-dependent manner.
Tubular structures of endothelial cells
The process of angiogenesis is also characterized by the tube formation by endothelial cells. In the current study, we also assessed the effect of artemisinic acid on capillary tube formation by HUVECs in matrigel assay. It was observed that HUVECs form capillary-like tube structures and became elongated on matrigel. Stimulation with 10 ng/mL VEGF stimulated the differentiation of HUVECs to produce strong tube-like structures (Figure 3b). Artemisinic acid considerably suppressed the VEGF-induced tube formation by HUVECs on matrigel in a dose-dependent manner, signifying the potential effect of this compound on tumor angiogenesis.
Figure 3: a) Effects of artemisinic acid on the migration of cells. The cells were treated with increasing doses of artemisinic acid (0, 10, 50 and 100 µM). b) Effects of artemisinic acid on capillary structure generation of endothelial cells. The cells were treated with 0 (A), 10 (B), 50 (C) and 100 (D) µM dose of artemisinic acid respectively
VEGFR2, AKT and m-TOR signalling pathways
Finally we demonstrated the effect of artemisinic acid on the protein expressions and cell signalling molecules linked with angiogenesis. These pathways were activated by the interaction of VEGFR2 and VEGF using Western blot test. The results of this test are shown in Figure 4 and indicate that artemisinic acid repressed the VEGF-activated VGEFR2 phosphorylation in a dose-dependent manner. VEGFR2 activation results in the activation of various intracellular signalling molecules that are liable for endothelial cell proliferation, cell migration and survival. Further, it was observed that artemisinic acid significantly inhibited the activation of AKT and m-TOR which suggests that artemisinic acid expresses its antiangiogenic effects via the suppression of VEGFR2 activation, AKT and m-TOR signalling pathways.
Figure 4: Effect of artemisinic acid on VEGFR2, AKT and m-TOR signalling pathways evaluated by Western blot assay. Artemisinic acid could suppressed the activation of VEGFR along with inhibiting m-TOR signalling pathways
Discussion
In the current study, the main purpose was to evaluate the antitumor activity of artemisinic acid in human breast cancer cells (MCF-7). The effect of artemisinic acid on in cell viability was assessed by in vitro MTT assay. The in vivo antitumor effect of artemisinic acid was evaluated by using tumor xenograft mouse model of human breast cancer cells. Western blot assay demonstrated how artemisinic acid affects the various cell signalling pathways including VEGF, m-TOR and AKT pathways. It was observed that artemisinic acid led to the inhibition of in vitro and in vivo tumor angiogenesis. Artemisinic acid showed dose- and time-dependent anti-cancer effects in these cells. In vivo results showed that 5 mg/kg of artemisinic acid used every other day suppressed both tumor weight and tumor volume in a dose-dependent manner. Artemisinic acid led to the inhibition of VEGF-induced chemotactic migration in a concentration-dependent manner. In addition, artemisinic acid also inhibited the VEGF-induced tube formation signifying the potential of this compound on tumor angiogenesis and growth suppression. In order to confirm the biochemical pathway which was involved in the anti-angiogenesis potential of this compound, Western blot assay was used to demonstrate the effect of artemisinic acid on VEGFR, AKT and m-TOR cell signalling. It was seen that artemisinic acid inhibited the VEGF-activated VEGFR2 phosphorylation along with inhibiting the activation of AKT and m-TOR. Like artemisinic acid, tangeretin effectively decreased the expression of Akt, p-Akt, pGSK3-beta and mTOR with up-regulation of PTEN expression levels (Guo et al., 2015). The polysaccharides from Pleurotus eous effectively suppressed angiogenesis by down-regulating VEGF, and induced apoptosis (Xu et al., 2015).
The process of cancer metastasis consists of a series of interrelated sequential steps. Each step is rate-limiting step and it may be a target for therapy. The development of new blood vessels from pre-existing ones is called as the tumor angiogenesis. This process plays vital roles in cancer growth, metastasis and invasiveness of the tumors. About 90% of deaths which are cancer related arise due to angiogenesis process. The process of angiogenesis is triggered by cancer proliferation along with cell migration in response to chemotactic agents like VEGF. It has been observed that VEGF is expressed commonly in most of the tumors and as such it plays a key role in angiogenesis process (Kerbel, 2008; Weis and Cheresh, 2011; Carmeliet and Jain, 2000; Pyun et al., 2008). Keeping this in view, it is believed that targeting tumor angiogenesis is a potential therapeutic target for cancer treatment. The current study clearly explains the anti-angiogenesis potential of artemisinic acid.
Conclusion
Artemisinic acid inhibited the in vitro and in vivo tumor growth in MCF-7 cancer cells as well as in xenograft mice model. The compound also led to the inhibition of angiogenesis as evaluated by various assays including transwell migration assay and matrigel assay. Finally, Western blot assay showed that the mode of action behind the anti-angiogenesis potential of artemisinic acid is the inhibition of various cellular pathways like VEGFR, AKT and m-TOR pathways.
Ethical Issue
Mice were maintained according to NIH guidelines for animal research and care and all of the experimental protocols were approved by the Animal Investigation Committee of the Southern Hospital, Southern Medical University, Guangzhou 510515, China.
References
Carmeliet P, Jain RK. Angiogenesis in cancer and other diseases. Nature 2000; 407: 249-57.
Carmeliet P. Angiogenesis in life, disease and medicine. Nature 2005; 438: 932-36.
Compagni A, Christofori G. Recent advances in research on multistage tumorigenesis. Br J Cancer. 2000; 83: 1-5.
Falcon BL, Barr S, Gokhale PC, Chou J, Fogarty J, Depeille P, Miglarese M, Epstein DM, McDonald DM. Reduced VEGF production, angiogenesis, and vascular regrowth contribute to the antitumor properties of dual mTORC1/mTORC2 inhibitors. Cancer Res. 2011; 71: 1573-83.
Ferrara N, Kerbel RS. Angiogenesis as a therapeutic target. Nature 2005; 438: 967-74.
Guo J, Li Y, Xin L. Tangeretin prevents prostate cancer cell proliferation and induces apoptosis via activation of Notch signalling and regulating the androgen receptor (AR) pathway and the phosphoinositide 3-kinase (PI3k)/Akt/mTOR pathways. Bangladesh J Pharmacol. 2015; 10: 937-47.
Kerbel RS. Tumor angiogenesis. N Engl J Med. 2008; 358: 2039-49.
Kowanetz M, Ferrara N. Vascular endothelial growth factor signaling pathways: Therapeutic perspective. Clin Cancer Res. 2006; 12: 5018-22.
Liu LZ, Zheng JZ, Wang XR, Jiang BH. Endothelial p70 S6 kinase 1 in regulating tumor angiogenesis. Cancer Res. 2008; 68: 8183-88.
Pang X, Yi Z, Zhang J, Lu B, Sung B, Qu W, Aggarwal BB, Liu M. Celastrol suppresses angiogenesis-mediated tumor growth through inhibition of AKT/mammalian target of rapamycin pathway. Cancer Res. 2010; 70: 1951-59.
Pisanti S, Picardi P, Prota L, Proto MC, Laezza C, McGuire PG, Morbidelli L, Gazzerro P, Ziche M, Das A, Bifulco M. Genetic and pharmacologic inactivation of cannabinoid CB1 receptor inhibits angiogenesis. Blood 2011; 117: 5541-50.
Pyun BJ, Choi S, Lee Y, Kim TW, Min JK, Kim Y, Kim BD, Kim JH, Kim TY, Kim YM, Kwon YG. Capsiate, a non-pungent capsaicin-like compound, inhibits angiogenesis and vascular permeability via a direct inhibition of Src kinase activity. Cancer Res. 2008; 68: 227-35.
Quesada AR, Munoz-Chapuli R, Medina MA. Anti-angiogenic drugs: From bench to clinical trials. Med Res Rev. 2006; 26: 483-530.
Schlessinger J. New roles for Src kinases in control of cell survival and angiogenesis. Cell 2000; 100: 293-96.
Weis SM, Cheresh DA. Tumor angiogenesis: Molecular pathways and therapeutic targets. Nat Med. 2011; 17: 1359-70.
Xu J, Yuan Q, Luo P, Sun X, Ma J. Pleurotus eous polysaccharides suppress angiogenesis and induce apoptosis via ROS-dependent JNK activation and mitochondrial mediated mechanisms in MCF-7 human breast cancer cells. Bangladesh J Pharmacol. 2015; 10: 78-86.
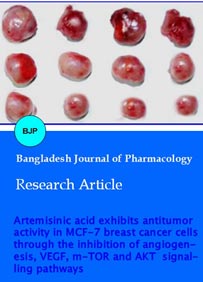