Anti-cancer effect of Atractylodes macrocephala extract by double induction of apoptotic and autophagic cell death in head and neck cancer cells
Abstract
The apoptosis resistance restricts the efficacy of traditional therapies of head and neck squamous cell carcinoma (HNSCC). To overcome this problem, Atractylodes macrocephala, a traditional Korean and Chinese herbal medicine, has been used in clinical practice. In the present study, the anticancer effect of A. macrocephala extract was investigated and its underlying mechanisms using head and neck cancer KB cell line. The extract-induced cytotoxicity was mediated by autophagic cell death as well as apoptosis in HNSCC cells though extrinsic apoptotic pathway and the Akt/mTOR autophagic pathway. This study suggests that A. macrocephala extracts can be a potential drug to overcome side effects and susceptibility for head and neck carcinoma by double induction of apoptosis and autophagy.
Introduction
Head and neck squamous cell carcinoma (HNSCC) is the malignancy with low survival rates and poor quality of life of patients (Johansen et al., 2000). Currently preferred therapy for HNSCC patients including surgery, radiotherapy, chemotherapy and biotherapy. Surgical resection is the main treatment for head and neck cancer. Chemoradiation therapy is applied to improve the quality of life in patients through organ preservation (Clark et al., 2001). The 5-years survival rate is less than 50%. The long-term survival rate is insignificantly improved (Hoffman et al., 2006). In addition, conventional chemotherapeutic agents have been associated with numerous adverse effects and complications. Moreover, apoptosis resistance is an important feature of head and neck cancer and it restricts the efficacy of traditional therapies (Hanahan and Weinberg, 2000). Consequently, it is critical to identify and develop new alternative drugs to overcome side effects and susceptibility for head and neck carcinoma.
Both cell apoptosis and autophagy are effective and secure methods ensuring a reduction in the number of cancer cells. Apoptotic cell death is a cellular self-destruction mechanism involved in various regulations of biological events, such as embryonic development, tissue homeostasis, normal cell turnover and removal of damaged cells (Hengartner, 2000). Molecular biological studies of apoptosis began in the late 1980s, and apoptosis has been recognized as an ideal method of cancer therapy. Chemotherapy and irradiation, which are used to treat cancer, induce apoptosis and decrease the number of cancer cells. Therefore, modulation the key elements of the apoptosis pathway affects the death of cancer cells. However, if cancer cells are resistant to apoptosis, it becomes an important clinical problem (Igney and Krammer, 2002).
The role of autophagy in the physiology of cancer cells remains controversial. Autophagy can prevent cancer cells from degrading damaged cells. Abnormal cells can prevent cell death and potentially contribute to the growth of cancer cells. Although many studies have been conducted at present, it is difficult to conclude whether autophagy is a tumor promoter or a tumor suppressor and autophagy-mediated cell death mechanism contributes to the efficacy of anti-cancer drugs (Sannigrahi et al., 2015). Therefore, double targeting apoptosis and autophagy will provide a promising therapeutic strategy to circumvent resistance and enhance the anticancer effect.
Atractylodes macrocephala Koidzumi, a traditional Korean and Chinese herbal medicine, has been widely used in clinical practice for the treatment of gastrointestinal diseases, abdominal pain, and obesity. It has been shown that A. macrocephala extract has anti-inflammatory (Ravipati et al., 2013), antiulcer (Dong et al., 2008), antioxidant (Igney and Krammer, 2002), and immunostimulant (Lee et al., 2007). Recently, A. macrocephala has been reported to show anti-tumor effect in human lung carcinoma cells (Liu et al., 2013), glioma C6 cells (Li et al., 2014), leukemia cells (Huang et al., 2005), and other various cancer cell lines (Choi, 2009). However, the anti-cancer effects and the cell death mechanism on head and neck cancers have not been studied.
Therefore, the present study was designed to investigate whether the extract of A. macrocephala could induce apoptosis and autophagy in KB cells and then checked apoptosis and autophagy-associated signals to clarify its underlying molecular mechanisms.
Materials and Methods
Preparation of A. macrocephala extract
Dried A. macrocephala was obtained from the Dong Kyung Pharma Co. Ltd. (Korea) and it was cut into pieces and powdered. A 300 g powder was accurately weighed and extracted with 1,500 mL of 70% ethanol at room temperature for 48 hours using a dynamoelectric stirrer (MTOPS, Korea). The resulting ethanol extract was filtered with filter paper (Advantec, Japan), then evaporated to concentrate at 45°C and dried by a rotary vacuum evaporator (EYELA, Japan). The dried powder obtained was stored at -80°C and dissolved in sterilized PBS prior to assay.
Cell viability assay
To determine the A. macrocephala concentration, KB cells were seeded at 1 x 105 /mL on a 96-well plate and cultured for 24 hours in DMEM supplemented with 5% CO2 at 37°C, 10% FBS and penicillin/ streptomycin. Cells were then treated with different concentrations of the extract for 24 hours. Then, the cells were washed with 1x PBS, and 10 mL of MTT solution was diluted in the cell culture medium and incubated in an incubator for 3 hours. After 3 hours, the formazan product was dissolved using dimethyl sulfoxide. When dissolved in DMSO, living cells became purple. Finally, the optical density was measured using an ELx 800 UV ELISA reader (Bio-Tek Instruments, USA) at 570 nm.
Diff-Quick® staining
To analyze the morphological changes of KB cells, cells were seeded in 4 well cell culture slide chamber at a cell density of 1x106/mL were treated with A. macrocephala at the different concentrations. After 24 hours, the cells were fixed at room temperature using paraformaldehyde (4%) for 5 min.
The fixed cells were treated with Diff-Quick® solution (Sysmex Co., Japan). Next, the fixed cells, add 100 uL of solution 1 (nuclear stain) and stain for 30 sec. After washing with 1x PBS for 10 sec, add 100 uL of solution 2 (cytoplasmic stain) and stain for 30 sec. Cell morphology was examined by an optical microscope (Olympus, Japan) for apoptotic cells if they showed condensation of the chromatin and/or the presence of cell membrane blebbing.
Detection of apoptosis by TUNEL assay
TUNEL assay (Promega, USA) was performed to detect the apoptosis induced A. macrocephala extract-treated KB cell. In brief, KB cell induced cell death by extract solution and the cells were fixed with 4% methanol-free formaldehyde 5 min and then washed using 1x PBS solution (potassium buffered saline) 4 times at room temperature. The cells made permeable with TritonX-100 solution (0.2%) and washed twice with 1x PBS. Then the cells were incubated with 100 uL reaction mixture containing biotinylated nucleotide mix and recombinant terminal deoxynucleotidyl transferase (rTdT) in equilibration buffer for 1 hour. To remove the unincorporated biotinylated nucleotides, the cells were incubated in 2 x SSC solution at 37°C for 5 min. After washing with 1x PBS, horseradish peroxidase-labeled streptavidin (HRPâ€streptavidin) diluted in 1x PBS was treated to the slides for 30 min at 37°C room temperature. A. macrocephala-induced apoptosis cell was stain 3, 3'-diaminobenzidine (DAB) solution. After washing with 1x PBS, the cells were mounted DAPI to stain the nuclei. The stained cells were analyzed using a confocal laser scanning microscope (Carl Zeiss).
Detection of autophagy by acridine orange-red staining
Autophagy was mediated by the autophagy-related genes and promotion of acidic vesicular organelles (AVO).
Acridine orange fluorescence green when it binds to DNA and fluorescence a red in AVO, therefore green intensity indicates DNA content and red intensity indicates AVO. The cytoplasm and nucleus of the stained cells were bright green color, whereas the acidic autophagic vacuoles showed bright red color. To detect the development of AVOs, we treated KB cells were treated with the extract for 24 hours, briefly KB cell washed with 1x PBS, and added acridine orange hydrochloride solution (0.5 ug/mL in doubled distilled water) for 20 min in extract-treated cells and analyzed by a confocal laser scanning microscope (Zeizz, Germany).
Determination of caspase-like protease activity assays
Caspase-like protease activity (Casp-3, -8, and -9/6) was measured using ApoAlert apoptosis assay kits (Clontech, USA) according to the manufacturer's directions. In brief, untreated or extract-treated cells were washed with ice-cold 1x PBS and pelleted cells lysed used cell lysis buffer.
Cell lysates were incubated with specific colorimetric peptide substrates for each caspase-like and reaction buffer obtaining a final concentration 10 mM 1,4-dithiothreitol (DTT) solution. In each sample of reaction, a specific irreversible inhibitor of each protein was added 1 uL to cell lysates of extract-treated samples prior to the addition of substrate. Colorimetric detection for caspase-3 and caspase-8 were performed using a microplate reader at 405 nm, and fluorometric detection for caspase-9/6 was performed using excitation and emission wavelengths of 380 and 460 nm filter.
Western blot analysis
A. macrocephala extract-treated KB cells were harvested using a spatula, pelleted, and then RIPA buffer (pH 7.4, containing 1% protease, protease inhibitor cocktail) was prepared and then protein was extracted. Next, which included 100 ug of protein per sample, were separated by 12% SDS-PAGE gel and detected related apoptosis and autophagy antibodies. The antibodies anti-LC3, anti-mTOR, anti-ATG5, anti-Bcl-2, anti-BAX, anti-PARP, and anti-beta-actin were purchased from Cell signaling (MA, USA). The secondary antibodies used were HRP-conjugated anti-mouse or anti-rabbit antibodies). The chemiluminescence kit (Amersham Biosciences, UK) was used to detect the secondary antibody. Anti-beta-actin antibody was used to standardize the amount of total protein
Results
Effects on cell viability and cell morphology
The cell viability was significantly reduced in a dose-dependent manner (Figure 1A). KB cells treated with 3 mg/mL A. macrocephala extract for 24 hours showed about 40% cell survival rate whereas 15% cell viability at 4 mg/mL A. macrocephala. The biochemical hallmarks of apoptosis, the chromatic condensation, cell shrinkage and apoptotic bodies were shown in Figure 1B.
Figure 1: Effects of A. macrocephala (AMK) on the cell viability and histomorphologic evaluation of KB cells. (A) Cells were treated with various concentrations of A. macrocephala (1-4 mg/mL) for 24 hours. Cell viability was analyzed using an MTT assay. bp<0.005 and cp<0.0005 vs. the untreated-control (Con). (B) The cells were then stained with Diff-Quick and observed by fluorescence microscopy with low magnification (40x) and high magnification (200x)
Apoptotic and autophagic cell death
A. macrocephala caused an increase in the number of apoptotic cells in dose-dependent manner, compared with untreated KB cells (Figure 2, upper panel). It was consistent with Diff-Quick staining results. There were cytomorphological alterations corresponding to a typical morphology of apoptosis, cell shrinkage, loss of contact with neighboring cells, membrane blebbing, and formation of apoptotic bodies in KB cell treated with A. macrocephala in a dose-dependent manner. In addition, autophagic cell staining of KB cells with acridine orange showed the accumulation of AVO in the cytoplasm of cells exposed to 1-4 mg/mL A. macrocephala in a dose-dependent manner (Figure 2, lower panel). These results confirmed that A. macrocephala promoted both apoptotic and autophagic cell death of KB cell lines in vitro.
Figure 2: A. macrocephala (AMK) has dual induction effect to apoptosis and autophage in a dose-dependent manner. Apototic cell death and autophagosome formation was detected after 24 hours treatment of A. macrocephala using TUNEL staining (upper panel) acridine orange red staining (lower panel). Microscopic photos were taken at 100x magnification
Apoptotic pathway involved in the anti-cancer effect
The activity of caspase-3 was increased within 3 hours after exposure to 3-4 mg/mL of A. macrocephala, reaching a peak at 6 hours (3 mg/mL) and 12 hours (4 mg/mL). In 1-2 mg/mL of A. macrocephala treatments, the activity of caspase-3 did not increase until 6 hours and reaching a peak at 12 hours after exposure (Figure 3A). Moreover, the activity of caspse-8 increased in time-dependent and dose-dependent manners except for 1 mg/mL of A. macrocephala exposure. However, caspase-9 activity was not shown remarkable changes in KB cells (Figure 3C). At the onset of apoptosis, the activation of caspase-3 further causes the cleavage or degradation of several important substrates including poly ADP-ribose polymerase (PARP), a hall marker of apoptosis. After A. macrocephala treatment in a dose-dependent manner for 12 hours, we examined the effects on the levels of Bcl-2, Bax, and PARP cleavage by Western blotting (Figure 3D). A. macrocephala extract-induced PARP cleavage was increased, which was visible by the appearance of a protein band of 85 kDa and the disappearance of an 116 kDa band. Moreover, the ratio of Bax (proapoptotic protein) to Bcl-2 (antiapoptotic protein) expression was also measured to examine the commitment to apoptosis (Figure 3E). Increase in the Bax:Bcl-2 ratio after extract exposure can cause the mitochondrial release of proapoptotic molecules. The results suggested that extract induced-apoptosis was accompanied by the activation of caspase-3 and caspase-8, as well as proteolytic cleavage of PARP in KB cells.
Figure 3: A. macrocephala (AMK)-induced extrinsic apoptosis pathway. (A-C) Caspase-3, 8 and 9 activity were measured by colorimetric protease assays following exposure of KB cells to A. macrocephala in a dose-dependent manner. (D) The protein levels of PARP-1, Bax, and Bcl-2. Equal protein loading was confirmed by the antibody reaction to beta-actin. (E) The Bax:Bcl-2 radio was determined at the protein level. bp<0.001, and cp<0.0001 vs. non-treated control
Autophagic pathway involved in the anti-cancer effect
A. macrocephala reduced the expression level of mTOR, but induced expression of ATG5 and LC3 in a dose-dependent manner (Figure 4A). During the formation of autophagosomes, LC3 protein was synthesized and transformed from LC3-I (cytoplasm) to LC3-II (auto-phagosome) protein (Klionsky et al., 2008). A. macrocephala specifically induced the expression of LC3-II proteins. The down-regulation of mTOR and up-regulation of ATG5 and LC3-II supports autophagy induced by A. macrocephala in KB cells. In order to further understand and define the effects of A. macrocephala on the mTOR pathway, we investigated the phosphorylation levels of two activated upstream regulators of mTOR activity, AMP-activated protein kinase (AMPK) and Akt (protein kinase B). No changes in AMPK, the decreasing of Akt phosphorylation were observed in A. macrocephala exposed KB cells in a dose-dependent manner (Figure 4B). These results suggest the extract-induced autophagy in KB cells occurs through the down-regulation of pAkt/mTOR pathway not activation of AMPK pathway.
Figure 4: A. macrocephala (AMK)-induced autophage via inhibition of Akt/mTOR pathway. (A) After incubation with A. macrocephala for 24 hours in a dose-dependent manner, cells lysates were prepared, then subjected to Western blot to measure pmTOR, mTOR, ATG5, and LC3. Data represent 2 independent experiments. (B) Cell were treated as above. Western blot performed to measure the expression levels of pAMPK, AMPK, pAkt, and Akt. Equal protein loading was confirmed by the antibody reaction to beta-actin. (C) Summary of effects of A. macrocephala on the expression of autophage related proteins. Protein levels were measured by densitometry and their relative levels were compared with the non-treated control. ap<0.01, bp<0.001, and cp<0.0001 vs. non-treated control
Discussion
The present study shows that A. macrocephala is effective against the proliferation of head and neck cancer KB cell line. A. macrocephala induces the KB cells to undergo apoptosis and autophagy. The cell viability significantly decreased in a dose-dependent manner. In addition, A. macrocephala induced a dose-dependent cell shrinkage, chromatic condensation and apoptotic bodies in KB cells. These results were consistent with the TUNEL colorimetric assay that A. macrocephala induced DNA fragmentation in a dose-dependent manner during programmed cell death. In addition, A. macrocephala induces the activation of PARP, caspase-3, and caspase-8, which preceded the onset of apoptosis. A. macrocephala results in an increase in proapoptotic Bax expression and a decrease in antiapoptotic Bcl-2 expression. There is a change in the ratio of Bax to Bcl-2 contributing to the apoptosis-promotion activity of A. macrocephala. Two apoptosis signaling pathways have been proposed: the death receptor pathway (extrinsic) and the mitochondrial pathway (intrinsic). In both pathways, the induction of apoptosis leads to activation of an initiator caspase, caspase-8 for the extrinsic pathway and caspase-9 for the intrinsic pathway (Igney and Krammer, 2002). Interestingly, A. macrocephala activated caspase-8, an initiator for extrinsic apoptosis pathway, but it did not activate caspase-9, an initiator for intrinsic apoptosis pathway. These results suggested that A. macrocephala initiates apoptotic cell death from extrinsic pathway through death receptors on the cell surface. However, extrinsic and intrinsic pathways eventually interact to amplify the apoptotic signal including activation of caspase-3, Bax, and PARP proteins (Fluda, 2009). Indeed, A. macrocephala induced the activation of Bax, caspase-3, and PARP.
In HNSCC, apoptosis resistance restricts the efficacy of traditional therapies (Hanahan and Weinberg, 2000). Therefore, two or more cellular processes are usually targeted in therapy. Basal autophagy plays an important role in maintaining the cellular homeostasis and genomic integrity by degrading aged/malfunctioning organelles and damaged/misfolded proteins. It is often suggested that prolonged activation of autophagy may lead to cell death by cellular self-degradation and the autophagy plays a tumor-suppressing role in cancer (Choi, 2012). The serine/threonine kinase mTOR has been known to be a key regulator of autophagy to inhibit cell autophagy (Jung et al., 2010). Our results show that A. macrocephala treatment decreases mTOR activity and it was correlated with the increase of ATG5, and LC3-II which interfere with the formation of autophagosomes. The Akt/mTOR signaling pathway is important in regulating autophagy and suppression of Akt decreases mTOR activity and promotes autophagy (Song et al., 2005). The anti-cancer effect of naringenin chalcone is mediated via the induction of autophagy, apoptosis and activation of PI3K/Akt signaling pathway (Zhang et al., 2016).
AMPK-mediated mTOR inhibition also promotes autophagy. When KB cells treated with A. macrocephala, the phosphorylation of Akt was decreased in a dose-dependent manner, but the phosphorylation of AMPK did not show significant changes.
Conclusion
A. macrocephala induces autophagy via inhibition of mTOR requires Akt inhibition, not the AMPK activation in KB cells.
References
Choi EJ, Kim GH. Atractylodes japonica rhizome inhibits cell proliferation and induces apoptosis in vitro. Food Sci Biotechnol. 2009; 18: 1019-21.
Choi KS. Autophagy and cancer. Exp Mol Med. 2012; 44: 109-20.
Clark J, Hofmeister C, Choudhury A, Matz G, Collins S, Bastian R, Meloan E, Emami B, Petruzzelli G. Phase II evaluation of paclitaxel in combination with carboplatin in advanced head and neck carcinoma. Cancer 2001; 92: 2334-40.
Dong H, He L, Huang M, Dong Y. Anti-inflammatory components isolated from Atractylodes macrocephala Koidz. Nat Prod. 2008; 22: 1418-27.
Feng Z, Xu Q, Chen W. Epigentic and genetic alteration-based molecular classification of head and neck cancer. Exper Rev Mol Diagn. 2012; 12: 279-90.
Fluda S. Tumor resistance to apoptosis. Int J Cancer. 2009; 124: 511-15.
Hanahan D, Weinberg RA. The hallmarks of cancer. Cell 2000; 100: 57-70.
Hengartner MO. The biochemistry of apoptosis. Nature 2000; 407: 770-76.
Hoffman HT, Porter K, Karnell LH, Cooper JS, Weber RS, Langer CJ, Ang KK, Gay G, Stewart A, Robinson RA. Laryngeal cancer in the United States: Changes in demographics, patterns of care, and survival. Laryngoscope 2006; 116: 1-13.
Huang HL, Chen CC, Yeh CY, Huang RL. Reactive oxygen species mediation of baizhu-induced apoptosis in human leukemia cells. J Ethnopharmacol. 2005; 97: 21-29.
Igney FH, Krammer PH. Death and anti-death: Tumour resistance to apoptosis. Nat Rev Cancer. 2002; 2: 277-88.
Johansen LV, Grau C, Overgrrard J. Hypopharyngeal squamous cell carcinoma treatment results in 138 consecutively admitted patients. Acta Oncol. 2000; 39: 529-36.
Jung CH, Ro SH, Cao J, Otto NM, Kim DH. mTOR regulation of autophagy. FEBS Lett. 2010; 584: 1287-95.
Klionsky DJ, Abeliovich H, Agostinis P, Agrawal DK, Aliev G, Askew DS, Baba M, Baehrecke EH, Bahr BA, Ballabio A, Bamber BA, Bassham DC, Bergamini E, Bi X, Biard-Piechaczyk M. Guidelines for the use and interpretation of assays for monitoring autophagy in higher eukaryotes. Autophagy 2008; 4: 151-75.
Lee JC, Lee KY, Son YO, Choi KC, Kim J, Kim SH, Chung GH, Jang YS. Stimulating effects on mouse splenocytes of glycoproteins from the herbal medicine Atractylodes macrocephala Koidz. Phytomedicine 2007; 14: 390-95.
Li X, Liu F, Li Z, Ye N, Huang C, Yuan X. Atractylodes macrocephala polysaccharides induces mitochondrial-mediated apoptosis in glioma C6 cells. Int J Biol Macromol. 2014; 66: 108-12.
Liu H, Zhu Y, Zhang T, Zhao Z, Zhao Y, Cheng P, Li H, Gao H, Su X. Anti-tumor effects of atractylenolide I isolated from Atractylodes macrocephala in human lung carcinoma cell lines. Molecules 2013; 18: 13357-68.
Ravipati AS, Zhang L, Koyyalamudi SR, Jeong SC, Reddy N, Bartlett J, Smith PT, de Pedro N, Melguizo Ã, Cantizani J, Asensio F, Vicente F. Antiproliferative activities of selected Chinese medicinal herbs against human cancer cell lines. Phytopharmacology 2013; 4: 206-19.
Sannigrahi MK, Singh V, Sharma R, Panda NK, Khullar M. Role of autophagy in head and neck cancer and therapeutic resistance. Oral Dis. 2015; 21: 283-91.
Song G, Ouyang G, Bao S. The activation of Akt/PKB signaling pathway and cell survival. J Cell Mol Med. 2005; 9: 59-71.
Zhang S, Jiang ZF, Pan Q, Song CY, Zhang WH. Anti-cancer effect of naringenin chalcone is mediated via the induction of autophagy, apoptosis and activation of PI3K/Akt signalling pathway. Bangladesh J Pharmacol. 2016; 11: 684-90.
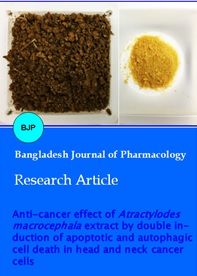