Anti-diabetic potential of Gaultheria trichophylla in mice
Abstract
This study evaluated Gaultheria trichophylla for its in vitro and in vivo anti-diabetic activity and its effect on biochemical parameters. The extract inhibited α-glucosidase with IC50 = 17.5 ± 0.1 μg/mL). The difference of body weight of the extract-treated diabetic groups and diabetic control was significant (p<0.05). The extract-treated and the standard-treated groups showed significant (p<0.001) decrease in the fasting blood glucose levels compared to diabetic control. The extract-treated groups after 15 days of treatment showed a significance (p<0.001 and p<0.05) decrease of the urea and creatinine levels respectively. The extract and standard-treated diabetic groups showed a significant decreased in the serum level of cholesterol, LDL, and triglycerides compared to the diabetic control group (p<0.001). The extract and standard drug showed a significance improvement of HDL level in diabetic mice compared to the diabetic control (p<0.001).
Introduction
Diabetes is a predominant public health concern and its prevalence is increasing all over the world. To treat type 2 diabetes mellitus and associated obesity, the potent glucosidase inhibitor from the natural source can be used (Ozaki et al., 2008). The people are diverting toward the herbal products because they are easily available, have low cost and some people are of the view that these are safe. Some of the allopathic medicines, like insulin and hypoglycemic agents taken orally are mainly used, but they bear some complications. These problems are expected to be partially overcome by the use of medicinal herbs because of their adverse effects and more accessibility (Shruthi et al., 2012).
Recently many plant species have been studied for their antidiabetic effects. For example, Cephalaria gigantean (Mbhele et al., 2015), Oncocalyx glabratus (Ahmed et al., 2015) and Pistacia lentiscus (Saad Ur Rehman et al., 2015) showed to possess antidiabetic activity.
Gaultheria trichophylla Royle (Ericaceae) is also known as Himalayan snowberry. The plant of genus Gaultheria is employed in the management of arthritis in traditional medicine (Liu et al., 2013), as anti-inflammatory agents (Zhang et al., 2011), and antibacterial activity (Cybulska et al., 2011). The author has explored this plant for its cytotoxic potential on cancer cell lines (Alam et al., 2015).
There are a paucity of information on the Gaultheria plants for their antidiabetic potential. For example, study has been carried out on the Gaultheria hispidula (Harbilas et al., 2009). Keeping in view the utilization of natural products as safe and effective agents for diabetes treatment, it is necessary to further explore natural sources. This study was, therefore, conducted to evaluate the antidiabetic potential of the methanol extract of G. trichophylla for in vivo and in vitro models.
Materials and Methods
Chemicals
Chemicals used were of analytical with high purity grade procured from the standard commercial sources. Methanol, diethyl ether, ethanol, ethyl acetate, chloroform, n-hexane were purchased from the Merck (Germany); Glibenclamide and alloxan monohydrate from the Sigma Aldrich; DMSO from the Fluka Chemicals; Glucose 5%, normal saline 0.9% from the Shahzeb Pharma, Pakistan; Cholesterol kit, triglycerides kit, acarbose and α-glucosidase from the Sigma–Aldrich Co., USA.
Plant material
G. trichophylla plant (5 kg) was collected in November 2013 from the Kaghan valley (Latitude:34° 54' 14.99" N, Longitude:73° 38' 30.59" E, altitude of 8,202 feet), District Mansehra, KPK, Pakistan. The plant was identified by the second author. It was placed at the herbarium of the institute labeled with a voucher specimen number (CTPHM-GT01, 13). The plant material was washed, shade dried and pulverized to powder. The powder material was kept in closed container before the process of extraction with organic solvent.
Preparation of the plant extract
The plant material in powder form (100 g) was extracted using soxhlet extractor for 20 hours each with organic solvents i.e. methanol, chloroform and n-hexane. It was preceded with the filtration process using Whatman grade 1 filter paper. In the next step, the filtrate was reduced to concentrated residue under reduced temperature (40°C) and pressure on vacuum rotary apparatus. The extraction yield was measured. The respective percentage yield for methanol, chloroform and n-hexane extracts were 21.9%, 16.4% and 7.0%. The desiccator was used to remove the remaining moisture, and finally the extracts were stored in the air tight containers at 4°C for further use.
Experimental animals
Healthy adult albino mice (26-30 g) of either sex were selected for the study. The animals were obtained from the National Institute of Health (NIH) and then bred in the Animal house of CIIT Abbottabad. Mice were kept in polypropylene cages (47 × 34 × 20 cm) lined with husk (changed every 24 hours). They were given a standard diet and water ad libitum. The pellets consisted of protein (23%), lipids (5%), crude fiber (4%), ash (8%), calcium (1%), phosphorus (0.6%), glucose (3.4%), and carbohydrates (5%).
α-Glucosidase inhibitory assay
The assay was carried out according to the method described elsewhere with slight modification (Matsui et al., 1996) . All the samples were dissolved in DMSO. An enzyme solution consisting of α-glucosidase (0.8 units/ml) in 50 mM phosphate buffer (pH 7), containing 100 mM NaCl was prepared fresh. The solution was maintained cool throughout the test. The substrate, pNP-G (0.7 mM) in phosphate buffer, was prepared fresh earlier to use. The test solution (20 µL) and enzyme solution (80 µL) was pre-incubated at 37°C for 5 min. The reaction was initiated with 1.9 mL of substrate solution and incubated for 15 min at 37°C. The reaction was stopped by adding 2.0 mL (0.5M) aqueous Tris solution, and the absorbance of PNP released from the PNP-G was noted at 400 nm. DMSO (20 µL) was kept as blank (without addition of the test solution). Acarbose was used as a positive control. Analysis was carried out in triplicates, and the results were calculated as ± SEM.
The α-glucosidase inhibitory activity was presented as the %age, calculated as
= 100 - (AB-AS)/AB
Where AB = absorbance of blank, AS = absorbance of the sample
Oral glucose tolerance test
The oral glucose tolerance test was conducted before the induction of diabetes in 18 hours fasted normal mice as per (Weir and Bonner-Weir, 2004). Healthy mice were randomly selected and distributed into five groups (n=6). Glucose 2 g/kg was fed. Blood glucose levels were measured from the vein of mice tail at 0, 60, 90, 120 and 150 min of glucose administration.
Induction of diabetes and experimental design
Antidiabetic activity was carried out on selected healthy albino mice (Ou et al. 2013). Diabetes was induced in mice using freshly prepared solution of alloxan in 0.9% w/v of NaCl. For inducing diabetes, the mice were kept on fasting for 12 hours and were given a single i.p. injection of alloxan monohydrate (150 mg/kg). To prevent fatal hypoglycemia initially due to massive pancreatic insulin release, the mice were provided with 5% glucose solution after six hours supplied in water bottles in their cages for next 24 hours. Animal were kept at room temperature (27 ± 2°C), humidity (55 ± 5%), and a 12 hours’ cycle of light and dark.
The glucose level of the fasting animals was measured after 72 hours. After acclimatization, the animals were separated into following the groups (six mice in each group); Groups A, Normal control treated with saline; B, Diabetic control; C, Diabetic mice treated with 500 mg/kg body weight of the extract; D, Normal mice given 500 mg/kg of methanol extract and E, reference control treated with glibenclamide (10 mgâ„kg). An identification mark was given to the mice of each group on the tail with a permanent marker. Each of mice was weighed and the dose was calculated accordingly. The extract was given orally. All the groups were given respective treatments daily for 15 days. To check the effect of the extracts on the weight of animals, weight of the mice was recorded prior to the administration of the extracts and at the end of the study as well i.e. on the day 15.
The blood samples were collected in glass test tubes and allowed to clot for 1 hour at 37°C. The blood was collected using capillary tubes into the Eppendorf Tubes® containing heparin for analysis of plasma profile. With a glass Pasteur, carefully, the clot was loosened from the sides of the tube. The serum was centrifuged at 5,000 rpm for 5 min at 4°C. A micropipette was used gently to separate the serum from the clot. The serum was labeled with the animal number and the estimations were made (Phuong et al., 2004).
Biochemical analysis
The blood sugar level was measured using Accu-Chek Active test meter by withdrawing the blood on the test strips from the vein of mice tail. Total cholesterol (TC) and triglycerides (TG) were assayed using the protocol described elsewhere (Burtis et al., 2012). The level of serum urea and creatinine were assayed using the protocol described elsewhere (Thomas et al., 1998). High density lipoprotein (HDL) and low density lipoprotein (LDL) were measured by the protocol described elsewhere (Burstein et al., 1970).
Statistical analysis
All the data obtained from the body weight, fasting blood sugar, and other biochemical assessments were represented as mean ± standard deviation and analyzed for ANOVA–Dunnet’s test. The differences between groups were considered significant at p<0.001 and p< 0.05 levels. The normal control was compared with the normal extract treated groups while diabetic control was compared with the diabetic extract treated and glibenclamide treated groups.
Results
α-Glucosidase inhibitory activity
The methanol extract of G. trichophylla demonstrated high activity against α-glucosidase (98.4 ± 2.5% inhibition and with IC50 = 17.5 ± 0.1 μg/mL, the chloroform and hexane extracts showed no prominent effect (49.5 ± 2.0 and 33.1 ± 3.2% inhibition respectively) (Table I). The standard drug acarbose was used as control (92.2 ± 0.1% (0.5 mM) inhibition and with IC50 = 38.2 ± 0.1 μg/mL. Therefore, it was decided to test the methanol extract for in vivo activity on animal model.
Table I: Alpha-glucose inhibitory activity of different extracts of G. trichophylla
Sample | %Alpha-glucosidase inhibition (0.5 mL/min) |
IC50 (µg/mL) |
---|---|---|
Methanol extract | 98.4 ± 2.5 | 17.5 ± 0.1 |
Chloroform extract | 49.5 ± 2.0 | - |
Hexane extract | 33.1 ± 3.2 | - |
Acarbose | 92.2 ± 0.1 (0.5 mM) |
38.2 ± 0.1 |
Data represented as mean ± S.D values of 6 animals each ap<0.001, bp<0.05 (One-way NOVA, Dunnet’s t-test, GraphPad Prism software) |
Effect on body weight
The effect of extract of G. trichophylla on body weight of mice showed that the change in the body weight of the normal groups (27.7 ± 2.3 g) (untreated) increased (34.3 ± 5.3 g) after 15 days (Table II). Similarly, the body weight of the normal groups (29.6 ± 2.3 g) treated with the methanol extract also increased (34.0 ± 1.8 g). The body weight of the diabetic control groups (32 ± 1.8 g), as expected, decreased (25 ± 3.8 g) significantly (p<0.05) after 15 days. The body weights of diabetic mice treated with methanol extract (33.0 ± 2.1 to 30.0 ± 3.1 g) of G. trichophylla and the standard (glibenclamide) (30 ± 1.8 to 29.3 ± 1.8 g) did not reduced to extent as observed in diabetic mice group and this difference between the groups was also significant (p<0.05).
Table II: Effect of G. trichophylla on body weight
Groups | Before treatment (g) | After treatment (g) |
---|---|---|
Normal | 27.7 ± 2.3 | 34.3 ± 5.3 |
Methanol extract | 29.7 ± 2.3 | 34.0 ± 1.8 |
Diabetic control | 32.0 ± 1.8 | 25.0 ± 3.8a |
Diabetic plus methanol extract | 33.0 ± 2.1 | 30.0 ± 3.1a |
Diabetic plus glibenclamide | 30.0 ± 1.8 | 29.3 ± 1.8a |
Data represented as mean ± S.D values of 6 animals each ap<0.001, bp<0.05 (One-way NOVA, Dunnet’s t-test, GraphPad Prism software) |
Effect on blood glucose level
The alloxan-induced diabetes had caused significant initial increase in the blood glucose levels (fasting) of all animal groups (Table III). The diabetic control group showed significant increase in blood glucose level throughout the study period as compared with the normal control group (p<0.001). However, the methanol extract treated and the glibenclamide-treated groups showed significant (p<0.001) reduction in the fasting blood glucose as compared to diabetic control.
The effect was more pronounced in the standard treated group, which showed significance decrease in the blood glucose level (p<0.001) from day 3 to day 15 of the experiment. The methanol extract of G. trichophylla caused a significance (p<0.05) decrease in the glucose level on day 12-15.
Table III: Effect of Gaultheria trichophylla extract on blood glucose level in diabetic mice
Groups | Glucose level (mg/dL) | ||||||
---|---|---|---|---|---|---|---|
Oth day | 3rd day | 6th day | 9th day | 12th day | 15th day | %Variance | |
Normal control | 110.5 ± 16.4 | 99.8 ± 17.2 | 99.3 ± 18.1 | 102.2 ± 28.8 | 107.5 ± 3.5 | 109.2 ± 2.9 | 4.7 |
Diabetic control | 333.7 ± 12.0a | 344.0 ± 20.9a | 345.3 ± 22.7a | 335.8 ± 40.3a | 358.0 ± 20.7a | 374.7 ± 16.3a | 6.8 |
Normal methanol extract-treated | 107.7 ± 19.0 | 96.0 ± 13.9 | 84.7 ± 6.7 | 88.17 ± 11.3 | 83.2 ± 14.3b | 82.8 ± 13.5b | 10.8 |
Diabetic plus methnol estract-treated | 336.3 ± 45.8 | 309.7 ± 51.3a | 301.7 ± 50.0a | 264.8 ± 54.9a | 233.7 ± 53.8a | 207.0 ± 30.7a | 17.9 |
Diabetic plus glibenclamide treated | 320.7 ± 22.9 | 271.5 ± 18.7a | 215.7 ± 18.8a | 154.2 ± 19.0a | 163.0 ± 13.0a | 136.3 ± 17.7a | 34.8 |
Data represented as mean ± SD values of 6 animals each ap<0.001, bp<0.05 (One-way ANOVA, Dunnet’s t-test, GraphPad Prism software). Normal control was compared with normal control and extract treated. The diabetic control was compared with diabetic extract/standard treated groups |
Effect on TC, TG, HDL and LDL
There was no significance difference in TC and TG levels when normal control (81.7 ± 2.1 and 75.3 ± 4.3 mg/dL) was compared with the normal extract treated group (81.0 ± 1.9 and 73.7 ± 3.9 mg/dL) (Figure 1). The diabetic control (163.2 ± 10.1 and 183.4 ± 7.2 mg/dL) showed hyperlipidemia compared with normal control as indicated by increased level of TC and TG in diabetic mice. The extract (113.7 ± 2.8 and 138.7 ± 4.5 mg/dL) and standard (122.6 ± 1.9 and 140 ± 3.9 mg/dL)-treated diabetic groups showed a significant decreased in the serum level of cholesterol and triglycerides compared to the diabetic control group (p<0.001). The results of hypolipidemic potential of the plant extract was comparable to standard drug.
Figure 1: Effect of extract and standard drug on total cholesterol (A), triglycerides (B), LDL (C) and HDL (D) levels of normal and alloxan-induced diabetic mice. Data represented as mean ± SD values of 6 animals each ap<0.001, bp<0.05 (One-way ANOVA, Dunnet’s t-test, GraphPad Prism software); “D + E†means extracted-treated in diabetic mice
Alloxan rendered diabetic groups showed a significant (p<0.001) decrease in HDL level (20.7 ± 1.0 mg/dL) compared to the normal control (42.5 ± 2.5 mg/dL). The extract (41.4 ± 1.4 mg/dL) and standard drug (37.7 ± 2.2 mg/dL) in the course of treatment for 15 days showed a significance improvement of HDL level in diabetic mice compared to the diabetic control ((p<0.001).
In contrast to HLD level, the LDL level was significantly increased in diabetic control (96.9 ± 3.0 mg/dL) compared to normal control (24.0 ± 1.0 mg/dL). The extract (65.8 ± 4.6 mg/dL) and standard drug (63.4 ± 3.8 mg/dL) treated groups showed to reduce the LDL level significantly (p<0.001) compared to the diabetic control after 15 days of treatment.
Effect on serum urea and creatinine level
Alloxan-treated diabetic mice showed a significance (p<0.001) increase in the serum urea level (17.7 ± 0.8 mmol/L) (Figure 2). Normal mice treated with the methanol extract for 15 days showed no prominent change in the urea level (4.6 ± 0.4 mmol/L) when compared with the normal control (5.1 ± 0.4 mmol/L). When compared with the diabetic control, the extract-treated groups after 15 days of treatment showed a significance (p<0.001) improvement (decrease) of urea level (11.9 ± 1.5 mmol/L). The standard drug (15.6 ± 0.6 mmol/L) also showed similar results.
Figure 2: Effect of extract and standard drug on serum urea (A) and creatinine (B) levels of normal and alloxan-induced diabetic mice. Data represented as mean ± S.D values of 6 animals each ap<0.001, bp<0.05 (One-way ANOVA, Dunnet’s t-test, GraphPad Prism software); “D + E†means extract-treated in diabetic mice
When compared with the normal group (28.2 ± 1.8 mmol/L) the diabetic control group displayed a significant (p<0.05) increase in serum creatinine level (37.3 ± 0.8 mmol/L). The normal mice groups treated with methanol extract showed no prominent effect on the serum creatinine level (29.5 ± 1.0 mmol/L). A significance (p<0.05) decrease in the serum creatinine level (33.7 ± 0.8 mmol/L) was observed with methanol extract, and (p<0.001) standard drug glibenclamide (32.8 ± 2.3 mmol/L) when compared with the diabetic control.
Discussion
α-Glucosidase is the key enzyme in the digestion of carbohydrates and it is present in the surface membranes of intestine. The α-glucosidase inhibitors suppress the postprandial hyperglycemia by retarding the liberation of glucose of oligosaccharides and disaccharides from complex carbohydrates in diet and therefore, delay the absorption of glucose (Gao et al., 2008). There is need to explore more natural sources which are safe and effective α-glucosidase inhibitors as potential agents against diabetes. Therefore, the methanol extract seemed to be the ideal inhibitor of this enzyme. In this study, the methanol extract of exhibited the strongest inhibition of α-glucosidase which was comparable with acarbose used as a positive control. This is depicted by the lowest IC50 generated with respect to the other extract and standard tested. This is in accordance with previous studies that an ideal anti-diabetic agent should have a strong inhibitory activity against α-glucosidases (Bairy et al., 2016). The inhibition of this enzyme slows down the breakdown of disaccharides to monosaccharides such as glucose. This results in reduced quantity of glucose absorbed into the blood and thus ameliorating hyperglycemia (Kazeem and Ashafa, 2015).
Antidiabetic potential of other Gaultheria species is also evident by the earlier studies (Harbilas et al., 2009; Karki et al., 2014; Nachar et al., 1990; Suman, 2014). The methanol extract of G. trichophylla at a concentration of 500 mg/kg displayed significant effect on the glucose tolerance of mice and the extracts also exhibited reduction in the fasting blood glucose levels of the normoglycemic mice, thus revealing the hypoglycemic effect of the extract. The hyperglycemic mice were treated for 15 days with the extracts and levels of glucose were measured. There was a gradual reduction of blood glucose levels in mice treated with the extract throughout the period of experiment. The results of the extract treated groups were compared statistically to glibenclamide and was observed as significant. Alloxan caused the destruction of β–cells of islets of Langerhans and stops the production of Insulin and results in induction of diabetes. Therefore, in this case the extracts might have produced the hypoglycemic effect by a mechanism not involving insulin. The other possible mechanism can be endogenous glucose generation inhibition or by the reduction of absorption of intestinal glucose and additionally enzyme inhibition mechanism cannot be over ruled as discussed earlier (Eddouks et al., 2004).
It is a common observation that the level of serum lipids is usually high in diabetes mellitus. This elevation can induce coronary heart disease. The hyperlipidemia that characterizes the diabetic conditions may be regarded as a result of the uninhibited actions of lipolytic hormones on the fat depots. Therefore, a drug therapy or a dietary provision can reduced the risk of vascular ailments by lowering the serum lipid concentration (Sikarwar and Patil, 2010). The decreased levels of TG and other cholesterol in diabetic animals that received glibenclamide revealed that these drugs do ameliorate dyslipidemia as described previously (Trivedi et al., 2004). The study revealed that the dose of 500 mg/kg of methanol extract recovered the level of serum TC and TG in a significant manner (p<0.001) compared to the diabetic control. The level of LDL, over a period of 15 days was significantly reduced (p<0.001) towards normal as compared with diabetic control. However, the level of cardioprotective lipid HDL was improved significantly by the extract in diabetic mice. The effect of extracts on HDL level of normal mice was not prominent. This shows significant hypolipidemic effect of the extract.
It has been observed that phenolic compounds could be responsible for the antidiabetic effect by preventing the destruction of β-cells through peroxidation chain reaction inhibition (Patel and Mishra, 2011). Previously, we have also evaluated the plant extract and have found to contain high phenolic contents (Alam et al., 2017). It is known fact that the alloxan inducted diabetes results in loss of body weight as indicated by diabetic control group. The results also revealed that extract and glibenclamide-treated groups did not show the loss in body weight as was observed in the diabetic control group. Increased serum levels of urea and creatinine are indicators of impaired renal function (Gerbes et al., 2002). Diabetic control mice showed an increased level of creatinine and urea and this level remained elevated throughout the period of study. The normal and diabetic groups treated with extract showed a little improvement (reduction) in both the urea and creatinine levels as compared with the diabetic control group over the 15 days of treatment.
Conclusion
To the best of our knowledge, the present study is the first to report on the anti-diabetic properties of Gaultheria trichophylla. The results show that oral administration of G. trichophylla extract can control increased glucose levels in diabetes and may be good source of α-glucosidase inhibitor and may be employed for the therapy of diabetes and its complication.
Ethical Issue
The approval of the Research, Ethical Committee (REC), department of Pharmacy, CIIT, Abbottabad was taken before the animal studies were conducted. The collection of plants from the Kaghan valley was made in such a way that the species’ abundance was not disturbed. Care and handling of animals followed the internationally accepted procedures according to the Institute for Laboratory Animal Research´s Guide for the Care and Use of Laboratory Animals.
References
Ahmed S, Mothana R, Al-Rehaily A. In vivo hypoglycemic and antidiabetic study of Oncocalyx glabratus. Bangladesh J Pharmacol. 2015; 10: 830-35.
Alam F, Saqib QN, Waheed A. Effect of crude saponins from Gaultheria trichophylla extract on growth inhibition in human colorectal cancer cells. Bangladesh J Pharmacol. 2015; 10: 160-65.
Alam F, us Saqib QN, Ashraf M. Gaultheria trichophylla (Royle): A source of minerals and biologically active molecules, its antioxidant and anti-lipoxygenase activities. BMC Complement Altern Med. 2017; 17: 3.
Bairy P, Das A, Nainwal L, Mohanta T, Kumawat M, Mohapatra P, Parida P. Design, synthesis and antidiabetic activity of some novel xanthone derivatives targeting α-glucosidase. Bangladesh J Pharmacol. 2016; 11: 308-18.
Burstein M, Scholnick H, Morfin R. Rapid method for the isolation of lipoproteins from human serum by precipitation with polyanions. J Lipid Res. 1970; 11: 583-95.
Burtis CA, Ashwood ER, Bruns DE. Tietz textbook of clinical chemistry and molecular diagnostics. Elsevier Health Sciences, 2012.
Cybulska P, Thakur SD, Foster BC, Scott IM, Leduc RI, Arnason JT, Dillon JAR. Extracts of Canadian first nations medicinal plants, used as natural products, inhibit Neisseria gonorrhoeae isolates with different antibiotic resistance profiles. Sex Trans Dis. 2011; 38: 667-71.
Eddouks M, Lemhadri A, Michel J-B. Caraway and caper: Potential anti-hyperglycaemic plants in diabetic rats. J Ethnopharmacol. 2004; 94: 143-48.
Gao H, Huang Y-N, Gao B, Xu P-Y, Inagaki C, Kawabata J. α-Glucosidase inhibitory effect by the flower buds of Tussilago farfara L. Food Chem. 2008; 106: 1195-201.
Gerbes A, Gülberg V, Bilzer M, Vogeser M. Evaluation of serum cystatin C concentration as a marker of renal function in patients with cirrhosis of the liver. Gut 2002; 50: 106-10.
Harbilas D, Martineau LC, Harris CS, Adeyiwola-Spoor DC, Saleem A, Lambert J, Caves D, Johns T, Prentki M, Cuerrier A. Evaluation of the antidiabetic potential of selected medicinal plant extracts from the Canadian boreal forest used to treat symptoms of diabetes: Part II. Can J Physiol Pharmacol. 2009; 87: 479-92.
Karki H, Upadhayay K, Pal H, Singh R. Antidiabetic potential of Zanthoxylum armatum bark extract on streptozotocin-induced diabetic rats. Int J Green Pharm. 2014; 8: 77.
Kazeem MI, Ashafa AOT. In-vitro antioxidant and antidiabetic potentials of Dianthus basuticus Burtt Davy whole plant extracts. J Herb Med. 2015; 5: 158-64.
Liu W-R, Qiao W-L, Liu Z-Z, Wang X-H, Jiang R, Li S-Y, Shi R-B, She G-M. Gaultheria: Phytochemical and pharmacological characteristics. Molecules 2013; 18: 12071-108.
Matsui T, Yoshimoto C, Osajima K, Oki T, Osajima Y. In vitro survey of a-glucosidase inhibitory food components. Biosci Biotechnol Biochem. 1996; 60: 2019-22.
Mbhele N, Balogun FO, Kazeem MI, Ashafa T. In vitro studies on the antimicrobial, antioxidant and antidiabetic potential of Cephalaria gigantea. Bangladesh J Pharmacol. 2015; 10: 214-21.
Nachar A, Vallerand D, Musallam L, Lavoie L, Badawi A, Arnason J, Haddad PS. The action of antidiabetic plants of the Canadian James Bay Cree Traditional. Diabetes Res Clin Pract. 1990; 10: S17-27.
Ou Y, Lin L, Yang X, Pan Q, Cheng X. Antidiabetic potential of phycocyanin: Effects on KKAy mice. Pharm Biol. 2013; 51: 539-44.
Ozaki S, Oe H, Kitamura S. α-Glucosidase inhibitor from Kothala-himbutu (Salacia reticulata WIGHT). J Nat Prod. 2008; 71: 981-84.
Patel MB, Mishra S. Hypoglycemic activity of alkaloidal fraction of Tinospora cordifolia. Phytomedicine 2011; 18: 1045-52.
Phuong M, Ali B, Aziz E, Abdellatif S, Yahia C, Pierre S. The petroleum ether extract of Nigella sativa exerts lipid lowering and insulin-sensitizing action in the rats. J Ethnopharmacol. 2004; 94: 251-59.
Saad Ur Rehman, M, Hafeez Kamran S, Ahmad M, Akhtar U. Antidiabetic activity of crude Pistacia lentiscus in alloxan-induced diabetes in rats. Bangladesh J Pharmacol. 2015; 10: 543-47.
Salimifar M, Fatehi-Hassanabad Z, Fatehi M. A review on natural products for controlling type 2 diabetes with an emphasis on their mechanisms of actions. Curr Diab Rev. 2013; 9: 402-11.
Shruthi A, Latha K, Vagdevi H, Pushpa B, Shwetha C. Anti-diabetic activity of the leaves extracts of Wrightia tinctoria on alloxan induced diabetic rats. J Chem Pharm Res. 2012; 4.
Sikarwar MS, Patil M. Antidiabetic activity of Pongamia pinnata leaf extracts in alloxan-induced diabetic rats. Int J Ayurveda Res. 2010; 1: 199.
Suman MLN. Evaluation of antidiabetic activity of Zanthoxylum alatum Roxb. leaves against streptozotocin-induced diabetic rats. Kolkata, Jadavpur University, 2014.
Thomas L, Whicher JT, Andert SE. Clinical laboratory diagnostics: Use and assessment of clinical laboratory results. Germany, TH-Books, 1998.
Trivedi NA, Mazumdar B, Bhatt JD, Hemavathi KG. Effect of shilajit on blood glucose and lipid profile in alloxan-induced diabetic rats. Indian J Pharmacol. 2004; 36: 373-76.
Weir GC, Bonner-Weir S. Five stages of evolving beta-cell dysfunction during progression to diabetes. Diabetes 2004; 53: S16-S21.
Zhang D, Liu R, Sun L, Huang C, Wang C, Zhang DM, Zhang TT, Du GH. Anti-inflammatory activity of methyl salicylate glycosides isolated from Gaultheria yunnanensis (Franch.) Rehder. Molecules 2011; 16: 3875-84.
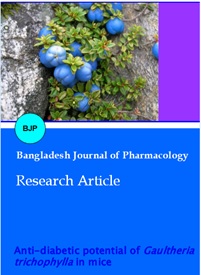