A new class of potential anti-diabetic acetohydrazides: Synthesis, in vivo anti-diabetic activity and molecular docking studies
Abstract
Two new pharmacologically active series of tetrazolopyridine-acetohydrazide conjugates [9 (a-n), 10 (a-n)] were synthesized by reacting a variety of suitably substituted benzaldehydes and isomeric 2-(5-(pyridin-3/4-yl)-2H-tetrazol-2-yl)acetohydrazides (7, 8). The synthesized compounds were analyzed through FTIR, 1H NMR, 13C NMR and elemental techniques. These acetohydrazides were screened for their in vivo antidiabetic activity and molecular docking studies. An excellent agreement was obtained as the best docked poses showed important binding features mostly based on interactions due to an oxygen atom and aromatic moieties of the series. The compounds 9a, 9c and 10l were found to be the most active in lowering blood glucose, having the potential of being good antidiabetic agents.
Introduction
Cardiovascular diseases account for the 65-75% deaths of the people with diabetes. Predominant form type 2 diabetes mellitus (T2DM) causes coronary diseases in the people by 2-fold more than in nondiabetic individuals. Sustained efforts are required to discover new antidiabetic drugs with reference to the pharmaceutical industry (Muir et al., 2013).
3- and 4-substituted pyridines have an effective role in respect of their biological activities, for example, derivatives of aminothiadiazole derived from the nicotinic and isonicotinic acids are antibacterial (Tomi et al., 2014) and pyridyl functionalized bis (pyrazol-1-yl)methanes are cytotoxic agents (Li et al., 2010). Compounds derived from the 3-substituted pyridine are good lyase inhibitors (Hart et al., 2004), metabotropic glutamate subtype 5 (mGluR5) antagonists (Iso et al., 2006) and anti-inflammatory agents (Rabea et al., 2006) while 4-pyridyl derivatives have pronounced antiviral (Cheng et al., 2005), aromatase inhibiting (Bayer et al., 1991), p38 MAP kinase inhibiting (Miwatashi et al., 2005) and anticonvulsant activities (Mohammad and Mohammad, 2009). Sulfanilamide derivatives of the 2-amino-4-ethoxycarbonylpyridine have pronounced antibacterial activity (Abd El-Salam et al., 2005). Thiazolo derivatives of 2,6-disubstituted isonicotinic acid hydrazides possess anti-inflammatory, analgesic, antiparkinsonian and anticonvulsant activities (Al-Omar et al., 2010).
It is known that compounds containing tetrazole ring have been established as being of use as anti-inflammatory, (Bepary et al., 2008) antimicrobial (El-Sayed et al., 2011) and antinociceptive (Maione et al., 2008) agents. A series of tetrazole-chromone derivatives have been declared as anti-allergic substances (Nohara et al., 1977) while 5-(pyridyl) tetrazole derivatives are efficient lipid regulators (Holland, 1969). We know some more derivatives having tetrazole moiety are good nonpeptide angiotensin receptor antagonists (Bradbury et al., 1993), SGLT2 inhibitors (Gao et al., 2010) and superoxide scavengers (Maxwell et al., 1984). Orally active, highly potent compounds derived from the picolinaldehyde bearing tetrazole ring are mGlu5 receptor antagonist (Huang et al., 2004).
Acetohydrazide derivatives are potent biologically active compounds as they are antimicrobial (Manna and Agrawal, 2009), analgesic (Kumar et al., 2014), antioxidant (Ahmad et al., 2010) and antiproliferating (Arafa et al., 2013). N'-substituted benzylidene-2-(2,4-dimethylphenoxy) acetohydrazides are known to possess antibacterial and enzyme inhibition activities (Nadeem et al., 2014) whereas acetohydrazide derivatives of 2-aminopyridine are intended as inhibitors of tumor necrosis factor alpha (TNF-a) production (Lacerda et al., 2014).
Taking into consideration of the aforesaid biological activities of the pyridine, tetrazole and acetohydrazide derivatives, we aimed to synthesize biologically active compounds having all three units with their valuable effects in a single structural entity.
Materials and Methods
For the synthesis of tetrazolopyridine-acetohydrazides, all chemicals were obtained from the commercial sources and used without additional purification. These compounds were confirmed by elemental analysis, Fourier Transform-IR (FT-IR) spectroscopy and NMR spectra.
General
Rf -values were determined using aluminum pre-coated silica gel plates Kieselgel 60 F254 from the Merck (Germany) using the solvent system (ethyl acetate: n-hexane 2:1). Melting points were determined using a stuart melting point apparatus (SMP3) and are uncorrected. Infrared spectra were recorded using an FTS 3000 MS, Bio-Rad Marlin (Excalibur Model) spectrophotometer. 1H NMR spectra were obtained using a Bruker 300 NMR MHz spectrometer in DMSO-d6. Chemical shifts were given in δ-scale (ppm). Abbreviations s, d, dd and m are used for singlet, doublet, double doublet, and multiplets, respectively. 13C NMR spectra (75 MHz) were measured in DMSO-d6. LCMS data were recorded on Agilent Technologies 6890N using an EI source of (70 eV). Elemental analyses were performed on CHNS 932 LECO instrument.
Synthesis of 3/4-(2H-tetrazole-5-yl)pyridine (3,4)
Compounds (3, 4) were prepared according to the literature procedure (Demko and Sharpless, 2001). A mixture of sodium azide (0.3 g, 3 mmol/L), 3/4-cyanopyridine (0.20 g, 2 mmol/L) (1, 2), and zinc (II) chloride (0.40 g, 3 mmol/L) was suspended in H2O (20 mL) and the reaction mixture was refluxed for 4-12 hours. On completion (TLC control), the mixture was cooled to room temperature and the solid obtained was treated with 3 N HCl (5 mL). In continuation of workup, solid residue was filtered and then washed with water to afford the product as a white solid and confirmed through melting point which are 240ºC (decomp.), Lit (Maione et al., 2008) 238ºC (decomp.) and 229-232ºC (decomp.), Lit (Maione et al., 2008) 227-228ºC (decomp.) for 3-(2H-tetrazole-5-yl) pyridine (3) and 4-(2H-tetrazole-5-yl) pyridine (4) respectively.
Synthesis of ethyl 2-(5-(pyridin-3/4-yl)-2H-tetrazol-2-yl)acetate (5, 6) (Saeed et al., 2014)
Ethyl chloroacetate (0.32 mL, 3 mmol/L) was added drop wise to a stirred solution of 3/4-(2H-tetrazole-5-yl) pyridine (0.44 g, 3 mmol/L) in chloroform in the presence of triethylamine (1.674 mL, 1 mmol/L). The reaction mixture was refluxed for 4-8 hours. The progress of the reaction was monitored by TLC. Excess of solvent was removed to afford off white crystals.
Ethyl 2-(5-(pyridin-3-yl)-2H-tetrazol-2-yl)acetate (5)
Yield: 78%; off white solid; m.p.: 162-164°C; Rf: 0.49 (ethyl acetate : n-hexane 2:1); FTIR (ATR, υ/cm-1): 3116 (Ar C-H), 2864 (CH3), 1759 (C=O), 1687 (C=N), 1547 (Ar C=C), 1282 (N=N-N); 1H NMR (300 MHz, DMSO-d6): δ 9.24 (d, J = 1.5 Hz, 1H, Ar-H), 8.76 (dd, J = 4.8, 1.5 Hz, 1H, Ar-H), 8.45-8.41 (m, 1H, Ar-H), 7.62 (dd, J = 7.8, 4.8 Hz, 1H, Ar-H), 5.95 (s, 2H, CH2), 4.22 (q, J = 7.2 2H, CH2), 1.32 (t, J = 7.2, 3H, CH3); 13C NMR: (75 MHz, DMSO-d6): 166.5, 162.7, 152.1, 147.6, 134.5, 124.9, 123.2, 62.53, 54.36, 14.38; LC-MS (m/z): (M)+, 234; Elemental analysis calculation for C10H11N5O2 (%): C, 51.50; H, 4.75; N, 30.03; Found: C, 51.48; H, 4.73; N, 30.08.
Ethyl 2-(5-(pyridin-4-yl)-2H-tetrazol-2-yl)acetate (6)
Yield: 75%; off white solid; m.p.: 171-173°C; Rf: 0.49(ethyl acetate : n-hexane 2:1); FTIR (ATR, υ/cm-1): 3113 (Ar C-H), 2869 (CH3), 1761 (C=O), 1685 (C=N), 1552 (Ar C=C), 1284 (N=N-N); 1H NMR (300 MHz, DMSO-d6): δ 8.78 (dd, J = 4.5,1.5Hz, 2H, Ar-H), 7.95 (d, J = 4.5, Hz, 2H, Ar-H), 5.99 (s, 2H, CH2),4.25 (q, J = 7.1 2H, CH2), 1.38 (t, J = 7.1, 3H, CH3); 13C NMR: (75 MHz, DMSO-d6): 166.7, 162.1, 151.3, 134.6, 125.0, 62.34, 54.40, 14.26; LC-MS (m/z): (M)+, 234; Elemental analysis calculation for C10H11N5O2 (%):C, 51.50; H, 4.75; N, 30.03; Found: C, 51.49; H, 4.77; N, 30.01.
Synthesis of 2-(5-(pyridin-3/4-yl)-2H-tetrazol-2-yl)acetohydrazide (7, 8) (Bhaskar and Mohite, 2010)
To a solution of ethyl 2-(5-(pyridin-3/4-yl)-2H-tetrazol-2-yl) acetate (0.233 g, 1.0 mmol/L) in 10 mL of dry distilled methanol, was added hydrazine hydrate (0.098 mL, 2 mmol/L). The reaction mixture was stirred at room temperature for half an hour to get acetohydrazides (7, 8). The precipitates were filtered and washed with cold methanol and then recrystallized with methanol chloroform mixture (1:1) to get pure white crystalline solid.
2-(5-(Pyridin-3-yl)-2H-tetrazol-2-yl)acetohydrazide (7)
Yield: 81%; white solid; m.p: 172-174°C Rf: 0.28 (ethyl acetate : n-hexane 2:1); FTIR (ATR, υ/cm-1): 3309 (NH2), 3134 (N-H), 3112 (Ar C-H), 1691 (C=N), 1669 (C=O),1546 (Ar C=C), 1281 (N=N-N);1H NMR (300 MHz, DMSO-d6): δ 12.02 (br, 1H, NH), 9.27 (d, J = 1.5 Hz, 1H, Ar-H), 8.61 (dd, J = 4.7, 1.5 Hz, 1H, Ar-H), 8.46 (d, J = 7.8, 1H, Ar-H), 7.71 (dd, J = 7.8, 4.8 Hz, 1H, Ar-H), 6.01 (s, 2H, CH2); 4.97 (br, 2H, NH2); 13C NMR: (75 MHz, DMSO-d6): 166.1, 162.5, 152.4, 146.9, 134.7, 124.5, 123.8, 54.41; LC-MS (m/z): (M)+1, 220; Elemental analysis calculation. for C8H9N7O (%): C, 43.83; H, 4.14; N, 44.73; Found: C, 43.81; H, 4.18; N, 44.76.
2-(5-(Pyridin-4-yl)-2H-tetrazol-2-yl)acetohydrazide (8)
Yield: 83%; white solid; m.p: 169-171°C Rf: 0.29 (ethyl acetate : n-hexane 2:1); FTIR (ATR, υ/cm-1): 3310 (NH2), 3137 (N-H), 3067 (Ar C-H), 2863 (CH3), 1689 (C=N), 1671 (C=O),1542 (Ar C=C), 1278 (N=N-N); 1H NMR (300 MHz, DMSO-d6): δ 12.04 (br, 1H, NH), 8.80 (dd, J = 4.5,1.5Hz, 2H, Ar-H), 7.91 (d, J = 4.5, Hz, 2H, Ar-H), 6.02 (s, 2H, CH2), 5.03 (br, 2H, NH2); 13C NMR: (75 MHz, DMSO-d6): 166.2, 162.4, 151.7, 134.5, 124.6, 55.40; LC-MS (m/z): (M)+, 220; Elemental analysis calculation for C8H9N7O (%): C, 43.83; H, 4.14; N, 44.73; Found: C, 43.85; H, 4.12; N, 44.75.
Synthesis of N΄-(substituted-benzylidene)-2-(5-(pyridin-3/4-yl)-2H-tetrazol-2-yl)aceto hydrazides 9(a-n),10(a-n)
2-(5-(pyridin-3/4-yl)-2H-tetrazol-2-yl) acetatohydrazide (0.21 g, 1.0 mmol/L) was added to the stirred solution of substituted benzaldehyde (1.0 mmol/L) in dry DMF in the presence of 0.5 mL of glacial acetic acid at 25-50°C for about 30 min to 5 hours. The progress of reaction was monitored by TLC. On completion, the reaction mixture was poured into an ice-cold water, solid separated was filtered and washed with hot water to get pure product.
N΄-(4-chlorobenzylidene-2-(5-(pyridin-3-yl)-2H-tetrazol-2-yl)acetohydrazide (9a)
Yield: 85%; off white solid; m.p.: 248°C (decomp.); Rf: 0.47 (ethyl acetate : n-hexane 2:1); FTIR (ATR, υ/cm-1): 3382 (N-H), 3119 (Ar C-H), 1692 (C=N), 1677 (amide C=O), 1571 (Ar C=C), 1275 (N=N-N); 1H NMR (300 MHz, DMSO-d6): δ 11.87 (br, 1H, NH), ), 9.23 (s, 1H, Ar-H), 8.95 (d, J = 4.8 Hz, 1H, Ar-H), 8.67-8.45 (m, 2H, Ar-H), 8.13 (s, 1H, CH=N), 7.69-7.41 (m, 4H, Ar-H), 6.15 (s, 2H, CH2); 13C NMR: (75 MHz, DMSO-d6): 166.5 (C=O), 162.1 (C=N, tetrazole), 156.9, 151.4, 147.2, 146.7, 135.2, 133.5, 132.0, 129.1, 127.8, 125.4, 54.70; LC-MS (m/z): (M)+, 342; Elemental analysis calculation for C15H12ClN7O (%): C, 52.72; H, 3.54; N, 28.69; Found: C, 52.83; H, 3.51; N, 28.57.
N΄-(3-chlorobenzylidene-2-(5-(pyridin-3-yl)-2H-tetrazol-2-yl)acetohydrazide (9b)
Yield: 88%; off white solid; m.p.: 235°C (decomp.); Rf: 0.44 (ethyl acetate : n-hexane 2:1); FTIR (ATR, υ/cm-1): 3384 (N-H), 3112 (Ar C-H), 1691 (C=N), 1679 (amide C=O), 1576 (Ar C=C), 1276 (N=N-N); 1H NMR (300 MHz, DMSO-d6): δ 12.11 (br, 1H, NH), 9.23 (d, J = 1.5 Hz, 1H, Ar-H), 8.74-8.68 (m, 3H, Ar-H), 8.04 (s, 1H, CH=N), 7.91-7.76 (m, 2H, Ar-H), 7.50-7.46 (m, 2H, Ar-H), 6.19 (s, 2H, CH2); 13C NMR: (75 MHz, DMSO-d6): 166.8 (C=O), 162.5 (C=N, tetrazole), 161.0, 151.9, 147.4, 144.0, 136.2, 134.3, 131.4, 130.3, 128.2, 127.4, 126.6, 124.9; LC-MS (m/z): (M)+, 342; Elemental analysis calculation for C15H12ClN7O (%): C, 52.72; H, 3.54; N, 28.69. Found: C, 52.83; H, 3.51; N, 28.57.
N΄-3-hydroxybenzylidene-2-(5-(pyridin-3-yl)-2H-tetrazol-2-yl)acetohydrazide (9c)
Yield: 78%; off white solid; m.p.: 221°C (decomp.); Rf: 0.46 (ethyl acetate : n-hexane 2:1); FTIR (ATR, υ/cm-1): 3374 (N-H), 3114 (Ar C-H), 1683 (C=N), 1669 (amide C=O), 1581 (Ar C=C), 1280 (N=N-N). 1H NMR (300 MHz, DMSO-d6): δ 11.89 (br, 1H, NH), 9.72 (br, 1H, OH), 9.26 (s, 1H, Ar-H), 8.75 (d, J = 4.5 Hz, 1H, Ar-H), 8.49 (d, J = 7.8 Hz, 1H, Ar-H), 8.17 (s, 1H, CH=N), 7.99 (s, 1H, Ar-H), 7.62 (dd, J = 7.8, 4.5 Hz, 1H, Ar-H), 7.27-7.09 (m, 3H, Ar-H), 6.16 (s, 2H, CH2); 13C NMR: (75 MHz, DMSO-d6): 166.4, 162.6 (C=N, tetrazole), 158.1, 151.9, 149.0, 147.6, 135.4, 134.4, 130.3, 124.8, 123.4, 119.5, 118.9, 118.2, 54.79; LC-MS (m/z): (M)+, 324; Elemental analysis calculation. for C15H13N7O2 (%): C, 55.72; H, 4.05; N, 30.33; Found: C, 55.78; H, 4.01; N, 30.41.
N΄-(4-(dimethylamino)benzylidene)-2-(5-(pyridin-3-yl)-2H-tetrazol-2-yl)acetohydrazide (9d)
Yield: 82%; yellow solid; m.p.: 210°C (decomp.); Rf : 0.39 (ethyl acetate : n-hexane 2:1); IR (KBr, vmax/cm-1): 3386 (N-H), 3115 (Ar C-H), 1682 (C=N), 1673 (amide C=O), 1592 (Ar C=C), 1275 (N=N-N); 1H NMR (300 MHz, DMSO-d6): δ 11.74 (br, 1H, NH), 9.26 (s, 1H, Ar-H), 8.75 (s, 1H, Ar-H), 8.50-8.43 (m, 2H, Ar-H), 8.13 (s, 1H, CH=N), 7.54 (d, J = 6.9 Hz, 2H, Ar-H), 6.73 (d, J = 6.9 Hz, 2H, Ar-H), 6.11 (s, 2H, CH2), 2.97 (s, 6H, CH3); 13C NMR: (75 MHz, DMSOd6): δ 166.9, 161.8, 158.4, 156.1, 152.3, 150.1, 148.9, 137.4, 135.1, 124.1, 122.9, 117.9, 54.11, 41.8; Elemental analysis calculation for C17H18N8O (%): C, 58.27; H, 5.18; N, 31.98; O, 4.57; Found: C, 58.21; H, 5.24; N, 31.86; O, 4.52.
N΄-(4-nitrobenzylidene)-2-(5-(pyridin-3-yl)-2H-tetrazol-2-yl)acetohydrazide (9e)
Yield: 82%; yellow solid; m.p.: 210°C (decomp.); Rf: 0.39 (ethyl acetate : n-hexane 2:1); FTIR (ATR, υ/cm-1): 3386 (N-H), 3115 (Ar C-H), 1682 (C=N), 1673 (amide C=O), 1592 (Ar C=C), 1275 (N=N-N); 1H NMR (300 MHz, DMSO-d6): δ 11.74 (br, 1H, NH), 9.26 (s, 1H, Ar-H), 8.75 (s, 1H, Ar-H), 8.50-8.43 (m, 2H, Ar-H), 8.13 (s, 1H, CH=N), 7.54 (d, J = 6.9 Hz, 2H, Ar-H), 6.73 (d, J = 6.9 Hz, 2H, Ar-H), 6.11 (s, 2H, CH2), 2.97 (s, 6H, CH3); 13C NMR: (75 MHz, DMSO-d6): δ 166.9 (C=O), 161.8 (C=N, tetrazole), 158.4, 156.1, 152.3, 150.1, 148.9, 137.4, 135.1, 124.1, 122.9, 117.9, 54.11, 41.8; LC-MS (m/z): (M)+, 351; Elemental analysis calculation for C17H18N8O (%): C, 58.27; H, 5.18; N, 31.98; Found: C, 58.21; H, 5.24; N, 31.86.
N΄-(2,4-dichlorobenzylidene)-2-(5-(pyridin-3-yl)-2H-tetrazol-2-yl)acetohydrazide (9f)
Yield: 81 %; yellow solid; m.p.: 246°C (decomp.). Rf: 0.41 (ethyl acetate : n-hexane 2:1); FTIR (ATR, υ/cm-1): 3385 (N-H), 3123 (Ar C-H), 1681 (C=N), 1667 (amide C=O), 1586 (Ar C=C), 1276 (N=N-N); 1H NMR (300 MHz, DMSO-d6): δ 12.09 (br, 1H, NH), 9.09 (s, 1H, Ar-H), 8.59-8.37 (m, 4H, Ar-H), 8.15 (s, 1H, CH=N), 7.87-7.61 (m, 3H, Ar-H), 6.11 (s, 2H, CH2); 13C NMR: (75 MHz, DMSO-d6): δ 166.9 (C=O), 163.6 (C=N, tetrazole), 157.4, 156.5, 152,1, 146.0, 142.4, 136.1, 133.8, 132.6, 125.9, 122.2, 55.14; LC-MS (m/z): (M)+, 353; Elemental analysis calculation for C15H12N8O4 (%): C, 48.92; H, 3.28; N, 30.42; Found: C, 48.75; H, 3.34; N, 30.51.
N΄-(2,4-dihydroxybenzylidene)-2-(5-(pyridin-3-yl)-2H-tetrazol-2-yl)acetohydrazide (9g)
Yield: 70%; light yellow solid; m.p.: 256°C (decomp.); Rf: 0.41 (ethyl acetate : n-hexane 2:1); FTIR (ATR, υ/cm-1): 3396 (N-H), 3113 (Ar C-H), 1687 (C=N), 1678 (amide C=O), 1561 (Ar C=C), 1272 (N=N-N); 1H NMR (300 MHz, DMSO-d6): δ 12.02 (br, 1H, NH), 9.18 (s, 1H, Ar-H), 8.69 9.26 (d, J = 4.6 Hz, 1H, Ar-H), 8.69 (d, J = 7.8 Hz, 1H, Ar-H), 8.20 (s, 1H, CH=N), 7.82 (dd, J = 4.6,7.8 Hz, 1H, Ar-H), 7.54 (d, J = 4.5 Hz, 1H, Ar-H), 6.72-6.66 (d, 2H, Ar-H), 6.04 (s, 2H, CH2); 5.61 (br, 2OH) 13C NMR: (75 MHz, DMSO-d6): 167.2 (C=O), 163.8 (C=N, tetrazole), 163.0, 162.8, 156.9, 154.8, 147.3, 135.7, 133.8, 133.1, 129.4, 125.7, 118.5, 112.7, 55.1; LC-MS (m/z): (M)+, 340; Elemental analysis calculation for C15H13N7O3 (%): C, 53.10; H, 3.86; N, 28.90; Found: C, 53.13; H, 3.89; N, 28.87.
N΄-(2-bromobenzylidene)-2-(5-(pyridin-3-yl)-2H-tetrazol-2-yl)acetohydrazide (9h)
Yield: 82%; pale yellow solid; m.p.: 288°C (decomp.); Rf: 0.36 (ethyl acetate : n-hexane 2:1); FTIR (ATR, υ/cm-1): 3381 (N-H), 3114 (Ar C-H), 1680 (C=N), 1672 (amide C=O), 1577 (Ar C=C), 1278 (N=N-N); 1H NMR (300 MHz, DMSO-d6): δ 12.03 (br, 1H, NH), 9.09 (s, 1H, Ar-H), 8.62 (d, J = 4.6 Hz, 1H, Ar-H), 8.54 (d, J = 7.8 Hz, 1H, Ar-H), 8.17 (s, 1H, CH=N), 7.69-7.59 (m, 3H, Ar-H), 7.47-7.32 (m, 3H, Ar-H), 6.02 (s, 2H, CH2); 13C NMR: (75 MHz, DMSO-d6): 167.5 (C=O), 163.2 (C=N, tetrazole), 157.9, 153.2, 146.9, 143.2, 137.4, 135.2, 134.6, 133.4, 132.8, 129.7, 126.8, 124.5, 55.04; LC-MS (m/z): (M)+, 387; Elemental analysis calculation for C15H12BrN7O (%): C, 46.6; H, 3.13; N, 25.39; Found: C, 46.52; H, 3.27; N, 25.34.
N΄-(3-bromobenzylidene)-2-(5-(pyridin-3-yl)-2H-tetrazol-2-yl)acetohydrazide (9i)
Yield: 75%; pale yellow solid; m.p.: 238°C (decomp.); Rf: 0.37 (ethyl acetate : n-hexane 2:1); FTIR (ATR, υ/cm-1): 3379 (N-H), 3111 (Ar C-H), 1681 (C=N), 1669 (amide C=O), 1579 (Ar C=C), 1281 (N=N-N); 1H NMR (300 MHz, DMSO-d6): δ 12.04 (br, 1H, NH), 9.19 (s, 1H, Ar-H), 8.87 (d, J = 4.8 Hz, 1H, Ar-H), 8.62 (d, J = 7.6 Hz, 1H, Ar-H), 8.17 (s, 1H, CH=N), 7.76-7.63 (m, 3H, Ar-H), 7.49-7.37 (m, 1H, Ar-H), 6.09 (s, 2H, CH2); 13C NMR: (75 MHz, DMSO-d6): 166.8 (C=O), 164.9 (C=N, tetrazole) , 157.2, 152.9, 146.7, 138.4, 136.2, 135.7, 133.9, 133.0, 132.8, 129.5, 126.1, 124.9, 54.59; LC-MS (m/z): (M)+, 387; Elemental analysis calculation for C15H12BrN7O (%): C, 46.6; H, 3.13; N, 25.39; Found: C, 46.52; H, 3.27; N, 25.34.
N΄-(2-hydroxy-5-nitrobenzylidene)-2-(5-(pyridin-3-yl)-2H-tetrazol-2-yl)acetohydrazide (9j)
Yield: 75.7%; yellow solid; m.p.: 259°C (decomp.); Rf: 0.42 (ethyl acetate : n-hexane 2:1); FTIR (ATR, υ/cm-1): 3386 (N-H), 3111 (Ar C-H), 1682 (C=N), 1671 (amide C=O), 1581 (Ar C=C), 1277 (N=N-N); 1H NMR (300 MHz, DMSO-d6): δ 12.14 (br, 1H, NH), 9.07 (s, 1H, Ar-H), 8.74 (d, J = 4.5 Hz, 1H, Ar-H), 8.54-8.42 (m, 3H, Ar-H), 8.08 (s, 1H, CH=N), 7.51-7.41 (m, 2H, Ar-H), 6.14 (s, 2H, CH2); 5.20 (br, 1H, OH); 13C NMR: (75 MHz, DMSO-d6): 166.3 (C=O), 166.1, 162.8 (C=N, tetrazole), 159.2, 152.4, 146.2, 143.5, 135.4, 134.1, 126.9, 125.8, 125.3, 121.8, 119.4, 56.02; LC-MS (m/z): (M)+, 369; Elemental analysis calculation for C15H12N8O4 (%): C, 48.92; H, 3.28; N, 30.42; Found: C, 48.86; H, 3.17; N, 30.61.
N΄-(2-chloro-6-flourobenzylidene)-2-(5-(pyridin-3-yl)-2H-tetrazol-2-yl)acetohydrazide (9k)
Yield: 78.4%; off white solid; m.p.: 261°C (decomp.); Rf: 0.38 (ethyl acetate : n-hexane 2:1); FTIR (ATR, υ/cm-1): 3386 (N-H), 3114 (Ar C-H), 1687 (C=N), 1651 (amide C=O), 1588 (Ar C=C), 1275 (N=N-N); 1H NMR (300 MHz, DMSO-d6): δ 12.09 (br, 1H, NH), 9.13 (s, 1H, Ar-H), 8.58 (d, J = 4.5 Hz, 1H, Ar-H), 8.49 (d, J = 4.5 Hz, 1H, Ar-H), 8.14 (s, 1H, CH=N), 7.84-7.62 (m, 4H, Ar-H), 5.94 (s, 2H, CH2); 13C NMR: (75 MHz, DMSO-d6): 166.5 (C=O), 163.4 (C=N, tetrazole), 162.6, 158.2, 154.9, 147.0, 140.5, 138.3, 136.7, 134.2, 125.6, 125.0122.3, 121.5, 56.08; LC-MS (m/z): (M)+, 360; Elemental analysis calculation for C15H11ClFN7O (%): C, 50.08; H, 3.08; N, 27.25; Found: C, 50.09; H, 3.13; Cl, 9.79; N, 27.27.
N΄-(3-flourobenzylidene)-2-(5-(pyridin-3-yl)-2H-tetrazol-2-yl)acetohydrazide (9l)
Yield: 82%; off white solid; m.p.: 263°C (decomp.); Rf: 0.42 (ethyl acetate : n-hexane 2:1); FTIR (ATR, υ/cm-1): 3376 (N-H), 3116 (Ar C-H), 1685 (C=N), 1665 (amide C=O), 1571 (Ar C=C), 1271 (N=N-N); 1H NMR (300 MHz, DMSO-d6): δ 12.08 (br, 1H, NH), 9.21 (d, J = 1.5 Hz, 1H, Ar-H), 8.74 (dd, J = 1.5, 4.8 Hz, 1H, Ar-H), 8.60-8.51 (m, 1H, Ar-H), 8.21 (s, 1H, CH=N), 7.81-7.62 (m, 4H, Ar-H), 7.54-7.47 (m, 1H, Ar-H), 6.15 (s, 2H, CH2); 13C NMR: (75 MHz, DMSO-d6): 166.9 (C=O) , 163.0 (C=N, tetrazole), 162.8, 159.9, 152.1, 147.1, 138.2, 135.7, 134.1, 132.8, 129.7, 126.0, 121.4, 119.6, 54.23; LC-MS (m/z): (M)+, 326; Elemental analysis calculation for C15H12FN7O (%): C, 55.38; H, 3.72; N, 30.14; Found: C, 55.27; H, 3.81; N, 30.16.
N΄-(2-flourobenzylidene)-2-(5-(pyridin-3-yl)-2H-tetrazol-2-yl)acetohydrazide (9m)
Yield: 80%; off white solid; m.p.: 252°C (decomp.); Rf: 0.42 (ethyl acetate : n-hexane 2:1); FTIR (ATR, υ/cm-1): 3387 (N-H), 3117 (Ar C-H), 1687 (C=N), 1662 (amide C=O), 1588 (Ar C=C), 1277 (N=N-N); 1H NMR (300 MHz, DMSO-d6): δ 12.10 (br, 1H, NH), 9.25 (s, 1H, Ar-H), 8.82-8.74 (m, 1H, Ar-H), 8.50-8.42 (m, 1H, Ar-H), 8.28 (s, 1H, CH=N), 8.05-7.88 (m, 1H, Ar-H), 7.62 (dd, J = 8.1, 4.8 Hz, 1H, Ar-H), 7.54-7.46 (m, 1H, Ar-H), 7.33-7.29 (m, 2H, Ar-H), 6.20 (s, 2H, CH2); 13C NMR: (75 MHz, DMSO-d6): 166.7 (C=O), 162.8 (C=N, tetrazole), 162.6, 159.5, 151.9, 147.6, 138.3, 138.2, 134.4, 132.7, 127.1, 125.3, 124.8, 123.4; LC-MS (m/z): (M)+, 326; Elemental analysis calculation for C15H12FN7O (%): C, 55.38; H, 3.72; N, 30.14; Found: C, 55.27; H, 3.81; N, 30.16.
N΄-(4-methoxybenzylidene)-2-(5-(pyridin-3-yl)-2H-tetrazol-2-yl)acetohydrazide (9n)
Yield: 79%; very light brown; m.p.: 257°C (decomp.); Rf: 0.41 (ethyl acetate : n-hexane 2:1); FTIR (ATR, υ/cm-1): 3381 (N-H), 3116 (Ar C-H), 1689 (C=N), 1678 (amide C=O), 1585 (Ar C=C), 1275 (N=N-N); 1H NMR (300 MHz, DMSO-d6): δ 12.03 (br, 1H, NH), 9.17 (s, 1H, Ar-H), 8.54 (d, J = 4.8 Hz, 1H, Ar-H), 8.42 (d, J = 8.3 Hz, 1H, Ar-H), 8.09 (s, 1H, CH=N), 7.67-7.51 (m, 3H, Ar-H), 7.39-7.26 (m, 2H, Ar-H), 5.93 (s, 2H, CH2) 4.94 (s, 3H, OCH3); 13C NMR: (75 MHz, DMSO-d6): 166.7 (C=O), 163.2 (C=N, tetrazole), 163.0, 156.2, 152.8, 146.5, 135.9, 134.1, 133.3, 129.4, 126.9, 121.3, 56.3, 55.07; LC-MS (m/z): (M)+, 338; Elemental analysis calculation for C16H12N7O2 (%): C, 56.97; H, 4.48; N, 29.07; Found: C, 56.92; H, 4.54; N, 29.15.
N΄-(4-chlorobenzylidene-2-(5-(pyridin-4-yl)-2H-tetrazol-2-yl)acetohydrazide (10a)
Yield: 81%; off white solid; m.p.: 249°C (decomp.); Rf: 0.46 (ethyl acetate : n-hexane 2:1); FTIR (ATR, υ/cm-1): 3372 (N-H), 3111 (Ar C-H), 1695 (C=N), 1677 (amide C=O), 1576 (Ar C=C), 1274 (N=N-N); 1H NMR (300 MHz, DMSO-d6): δ 12.04 (br, 1H, NH), 8.74 (dd, J = 1.5, 4.6 Hz, 2H, Ar-H), 8.14 (s, 1H, CH=N), 7.91-7.74 (m, 4H, Ar-H), 7.57 (d, J = 8.5Hz, 2H, Ar-H), 6.25 (s, 2H, CH2); 13C NMR: (75 MHz, DMSO-d6): δ 167.2 (C=O), 162.5 (C=N, tetrazole), 151.9, 146.8, 146.2, 134.0, 133.5, 129.7, 128.2, 125.7, 54.21; LC-MS (m/z): (M)+, 342; Elemental analysis calculation for C15H12ClN7O (%): C, 52.72; H, 3.54; N, 28.69; Found: C, 52.78; H, 3.56; N, 28.63.
N΄-(3-chlorobenzylidene-2-(5-(pyridin-4-yl)-2H-tetrazol-2-yl)acetohydrazide (10b)
Yield: 86%; off white solid; m.p.: 237°C (decomp.); Rf: 0.46 (ethyl acetate : n-hexane 2:1); FTIR (υ/cm-1): 3372 (N-H), 3117 (Ar C-H), 1695 (C=N), 1678 (amide C=O), 1568 (Ar C=C), 1278 (N=N-N); 1H NMR (300 MHz, DMSO-d6): δ 12.04 (br, 1H, NH), 8.83 (dd, J = 1.5, 4.5 Hz, 2H, Ar-H), 8.17 (s, 1H, CH=N), 7.97-7.58 , (m, 5H, Ar-H), 5.98 (s, 2H, CH2); 13C NMR: (75 MHz, DMSO-d6): δ 166.1 (C=O), 162.9 (C=N, tetrazole), 151.7, 145.3, 145.2, 136.9, 133.2, 131.4, 128.7, 126.9, 126.5, 125.9, 56.02; LC-MS (m/z): (M)+, 342; Elemental analysis calculation for C15H12ClN7O (%): C, 52.72; H, 3.54; N, 28.69; Found: C, 52.77; H, 3.61; N, 28.55.
N΄-3-hydroxybenzylidene-2-(5-(pyridin-4-yl)-2H-tetrazol-2-yl)acetohydrazide (10c)
Yield: 79%; off white solid; m.p.: 225°C (decomp.). Rf: 0.44 (ethyl acetate : n-hexane 2:1); FTIR (ATR, υ/cm-1): 3384 (N-H), 3119 (Ar C-H), 1691 (C=N), 1674 (amide C=O), 1578 (Ar C=C), 1275 (N=N-N); 1H NMR (300 MHz, DMSO-d6): δ 11.98 (br, 1H, NH), 9.54 (br, 1H, OH), 8.79 (d, J = 4.5 Hz, 2H, Ar-H), 8.16 (s, 1H, CH=N), 8.12-7.99 (m, 3H, Ar-H), 7.31-7.18 (m, 3H, Ar-H), 6.15 (s, 2H, CH2); 13C NMR: (75 MHz, DMSO-d6): δ 166.7 (C=O), 163.5 (C=N, tetrazole), 159.1, 152.0, 146.2, 135.8, 133.9, 130.7, 125.2, 124.1, 120.4, 118.6, 54.91; LC-MS (m/z): (M)+, 324; Elemental analysis calculation for C15H13N7O2 (%): C, 55.72; H, 4.05; N, 30.33; Found: C, 55.69; H, 4.11; N, 30.21.
N΄-(4-(dimethylamino)benzylidene)-2-(5-(pyridin-4-yl)-2H-tetrazol-2-yl)acetohydrazide (10d)
Yield: 84%; yellow solid; m.p.: 205°C (decomp.); Rf: 0.41 (ethyl acetate : n-hexane 2:1); FTIR (ATR, υ/cm-1): 3369 (N-H), 3116 (Ar C-H), 1682 (C=N), 1667 (amide C=O), 1568 (Ar C=C), 1277 (N=N-N); 1H NMR (300 MHz, DMSO-d6): δ 12.02 (br, 1H, NH), 8.63 (dd, J = 1.5, 4.7 Hz, 2H, Ar-H), 8.31 (d, J = 4.7 Hz, 2H, Ar-H), 8.21 (s, 1H, CH=N), 7.69 (d, J = 6.79 Hz, 2H, Ar-H), 6.84 (d, J = 6.79 Hz, 2H, Ar-H), 6.09 (s, 2H, CH2); 2.85 (s, 6H, CH3) 13C NMR: (75 MHz, DMSO-d6): δ 166.6 (C=O), 162.2 (C=N, tetrazole), 153.5, 151.8, 145.8, 133.6, 132.1, 125.7, 117.2, 55.04, 41.3; LC-MS (m/z): (M)+, 351; Elemental analysis calculation for C17H18N8O (%): C, 58.27; H, 5.18; N, 31.98; Found: C, 58.31; H, 5.22; N, 31.89.
N΄-(4-nitrobenzylidene)-2-(5-(pyridin-4-yl)-2H-tetrazol-2-yl)acetohydrazide (10e)
Yield: 85%; yellow solid; m.p.: 253°C (decomp.); Rf: 0.37 (ethyl acetate : n-hexane 2:1); FTIR (ATR, υ/cm-1): 3385 (N-H), 3123 (Ar C-H), 1697 (C=N), 1671 (amide C=O), 1582 (Ar C=C), 1280 (N=N-N);1H NMR (300 MHz, DMSO-d6): δ 12.06 (br, 1H, NH), 8.80 (dd, J = 1.5, 4.5 Hz, 2H, Ar-H), 8.28 (d, J = 4.7 Hz, 2H, Ar-H), 8.18 (s, 1H, CH=N), 8.06-7.98 , (m, 4H, Ar-H), 6.23 (s, 2H, CH2); 13C NMR: (75 MHz, DMSO-d6): δ 167.0 (C=O), 162.8 (C=N, tetrazole), 151.4, 148.5, 143.1, 140.4, 134.3, 128.6, 124.4, 120.8; LC-MS (m/z): (M)+, 353; Elemental analysis calculation for C15H12N8O4: C, 48.92; H, 3.28; N, 30.42; Found (%): C, 48.87; H, 3.33; N, 30.52.
N΄-(2,4-dichlorobenzylidene)-2-(5-(pyridin-4-yl)-2H-tetrazol-2-yl)acetohydrazide (10f)
Yield: 83%; off white solid; m.p.: 268°C (decomp.); Rf: 0.39 (ethyl acetate : n-hexane 2:1); FTIR (ATR, υ/cm-1): 3376 (N-H), 3110 (Ar C-H), 1684 (C=N), 1679 (amide C=O), 1577 (Ar C=C), 1278 (N=N-N);1H NMR (300 MHz, DMSO-d6): δ 11.87 (br, 1H, NH), 8.74 (dd, J = 1.5, 4.5 Hz, 2H, Ar-H), 8.20 (s, 1H, CH=N), 7.95-7.51 , (m, 5H, Ar-H), 6.01 (s, 2H, CH2); 13C NMR: (75 MHz, DMSO-d6): δ 166.3 (C=O), 161.8 (C=N, tetrazole), 152.1, 146.8, 141.5, 136.8, 133.8, 132.4, 131.2, 129.4, 128.7, 124.7, 54.9; LC-MS (m/z): (M)+, 377; Elemental analysis calculation for C15H12ClN7O (%): C, 52.72; H, 3.54; N, 28.69; Found: C, 52.77; H, 3.55; N, 28.71.
N΄-(2,4-dihydroxybenzylidene)-2-(5-(pyridin-4-yl)-2H-tetrazol-2-yl)acetohydrazide (10g)
Yield: 72%; dirty brown solid; m.p.: 252°C (decomp.); Rf: 0.42 (ethyl acetate : n-hexane 2:1); FTIR (ATR, υ/cm-1): 3389 (N-H), 3115 (Ar C-H), 1688 (C=N), 1677 (amide C=O), 1574 (Ar C=C), 1275 (N=N-N); 1H NMR (300 MHz, DMSO-d6): δ 12.05 (br, 1H, NH), 8.81 (dd, J = 1.5, 4.6 Hz, 2H, Ar-H), 8.15 (s, 1H, CH=N), 8.02-7.79 , (m, 3H, Ar-H), 6.81-6.69(m, 2H, Ar-H), 6.24 (br, 2H, OH), 5.94 (s, 2H, CH2); 13C NMR: (75 MHz, DMSO-d6): δ 167.2 (C=O), 163.2 (C=N, tetrazole), 162.9, 162.5, 146.5, 133.9, 132.8, 130.5, 125.4, 119.5, 115.9; LC-MS (m/z): (M)+, 340; Elemental analysis calculation for C15H13N7O3 (%): C, 53.10; H, 3.86; N, 28.90; Found: C, 53.14; H, 3.91; N, 28.89.
N΄-(2-bromobenzylidene)-2-(5-(pyridin-4-yl)-2H-tetrazol-2-yl)acetohydrazide (10h)
Yield: 71%; pale yellow solid; m.p.: 233°C (decomp.); Rf: 0.36 (ethyl acetate : n-hexane 2:1); FTIR (ATR, υ/cm-1): 3369 (N-H), 3120 (Ar C-H), 1685 (C=N), 1676 (amide C=O), 1569 (Ar C=C), 1275 (N=N-N); 1H NMR (300 MHz, DMSO-d6): δ 11.93 (br, 1H, NH), 8.91 (d, J = 4.5 Hz, 2H, Ar-H), 8.22 (s, 1H, CH=N), 7.85-7.67 , (m, 4H, Ar-H), 7.51-7.45 (m, 2H, Ar-H), 6.08 (s, 2H, CH2); 13C NMR: (75 MHz, DMSO-d6): δ 166.9 (C=O), 162.5 (C=N, tetrazole), 151.9, 144.7, 144.2, 135.8, 134.5, 134.0, 130.9, 130.2, 125.7, 56.03; LC-MS (m/z): (M)+, 387; Elemental analysis calculation for C15H12BrN7O (%): C, 46.6; H, 3.13; N, 25.39; Found: C, 46.65; H, 3.19; N, 25.36.</p
N΄-(3-bromobenzylidene)-2-(5-(pyridin-4-yl)-2H-tetrazol-2-yl)acetohydrazide (10i)
Yield: 78%; pale yellow solid; m.p.: 239°C (decomp.); Rf: 0.35 (ethyl acetate : n-hexane 2:1); FTIR (ATR, υ/cm-1): 3367 (N-H), 3119 (Ar C-H), 1699 (C=N), 1681 (amide C=O), 1574 (Ar C=C), 1274 (N=N-N); 1H NMR (300 MHz, DMSO-d6): δ 12.07 (br, 1H, NH), 8.75 (dd, J = 1.5, 4.5 Hz, 2H, Ar-H), 8.21, (s, 1H, Ar-H) 8.09 (s, 1H, CH=N), 7.80-7.64 , (m, 4H, Ar-H), 7.50-7.41 (m, 1H, Ar-H), 6.03 (s, 2H, CH2); 13C NMR: (75 MHz, DMSO-d6): δ 166.2 (C=O), 161.9 (C=N, tetrazole), 151.9, 143.9, 138.4, 136.0, 133.9, 133.9, 133.5, 133.2, 128.6, 124.9, 124.6, 56.02; LC-MS (m/z): (M)+, 387; Elemental analysis calculation for C15H12BrN7O (%): C, 46.60; H, 3.13; N, 25.39; Found: C, 46.56; H, 3.26; N, 25.35.
N΄-(2-hydroxy-5-nitrobenzylidene)-2-(5-(pyridin-4-yl)-2H-tetrazol-2-yl)acetohydrazide (10j)
Yield: 76%; yellow solid; m.p.: 261°C (decomp.); Rf: 0.41 (ethyl acetate : n-hexane 2:1); FTIR (ATR, υ/cm-1): 3374 (N-H), 3111 (Ar C-H), 1689 (C=N), 1672 (amide C=O), 1573 (Ar C=C), 1276 (N=N-N); 1H NMR (300 MHz, DMSO-d6): δ 12.11 (br, 1H, NH), 8.69 (dd, J = 1.5, 4.9 Hz, 2H, Ar-H), 8.51-8.38 (m, 2H, Ar-H), 8.20 (s, 1H, CH=N), 7.72-7.58 , (m, 3H, Ar-H), 5.98 (s, 2H, CH2); 5.27 (br, 1H, OH); 13C NMR: (75 MHz, DMSO-d6): δ 167.0 (C=O), 166.4, 163.1 (C=N, tetrazole), 152.1, 145.7, 143.8, 133.8, 127.2, 126.1, 125.4, 122.4, 119.8, 55.94; LC-MS (m/z): (M)+, 369; Elemental analysis calculation for C15H12N8O4 (%): C, 48.92; H, 3.28; N, 30.42; Found: C, 48.89; H, 3.33; N, 30.47.
N΄-(2-chloro-6-flourobenzylidene)-2-(5-(pyridin-4-yl)-2H-tetrazol-2-yl)acetohydrazide (10k)
Yield: 79%; off white solid; m.p.: 255°C (decomp.); Rf: 0.39 (ethyl acetate : n-hexane 2:1); FTIR (ATR, υ/cm-1): 3377 (N-H), 3122 (Ar C-H), 1698 (C=N), 1669 (amide C=O), 1576 (Ar C=C), 1279 (N=N-N); 1H NMR (300 MHz, DMSO-d6): δ 12.04 (br, 1H, NH), 8.89 (d, J = 4.9 Hz, 2H, Ar-H), 8.20 (s, 1H, CH=N), 7.79-7.57 (m, 5H, Ar-H), 6.04 (s, 2H, CH2); 13C NMR: (75 MHz, DMSO-d6): δ 166.9 (C=O), 163.0 (C=N, tetrazole), 162.4, 151.5, 145.4, 141.3, 137.2, 133.9, 125.6, 124.8, 122.8, 122.3, 55.79; LC-MS (m/z): (M)+, 360; Elemental analysis calculation for C15H11ClFN7O (%): C, 50.08; H, 3.08; N, 27.25; Found: C, 50.12; H, 3.15; N, 27.29.
N΄-(3-flourobenzylidene)-2-(5-(pyridin-4-yl)-2H-tetrazol-2-yl)acetohydrazide (10l)
Yield: 79%; light brown solid; m.p.: 259°C (decomp.); Rf: 0.42 (ethyl acetate : n-hexane 2:1); FTIR (ATR, υ/cm-1): 3373 (N-H), 3110 (Ar C-H), 1695 (C=N), 1673 (amide C=O), 1566 (Ar C=C), 1279 (N=N-N); 1H NMR (300 MHz, DMSO-d6): δ 11.89 (br, 1H, NH), 8.92 (dd, J = 1.5, 4.8 Hz, 2H, Ar-H), 8.09 (s, 1H, CH=N), 7.85-7.69 (m, 5H, Ar-H), 7.59-7.37(m, 1H, Ar-H), 5.97 (s, 2H, CH2); 13C NMR: (75 MHz, DMSO-d6): δ 166.8 (C=O), 163.2 (C=N, tetrazole), 162.7 (C=N, 152.2, 146.2, 139.4, 133.9, 132.4, 130.2, 124.8, 122.5, 120.5, 55.35; LC-MS (m/z): (M)+, 326; Elemental analysis calculation for C15H12FN7O (%): C, 55.38; H, 3.72; N, 30.14; Found: C, 55.21; H, 3.78; N, 30.18.
N΄-(2-flourobenzylidene)-2-(5-(pyridin-4-yl)-2H-tetrazol-2-yl)acetohydrazide (10m)
Yield: 79%; off white solid; m.p.: 261°C (decomp.); Rf: 0.43 (ethyl acetate : n-hexane 2:1); FTIR (ATR, υ/cm-1): 3376 (N-H), 3111 (Ar C-H), 1687 (C=N), 1676 (amide C=O), 1571 (Ar C=C), 1279 (N=N-N); 1H NMR (300 MHz, DMSO-d6): δ 12.17 (br, 1H, NH), 8.85 (dd, J = 1.5, 4.5 Hz, 2H, Ar-H), 8.23 (s, 1H, CH=N), 8.01-7.79(m, 1H, Ar-H), 7.87-7.61 (m, 3H, Ar-H), 7.54-7.42 (m, 2H, Ar-H), 6.05 (s, 2H, CH2), 4.87 (s, 2H, CH3); 13C NMR: (75 MHz, DMSO-d6): δ 166.2 (C=O), 162.5 (C=N, tetrazole), 162.3, 151.4, 145.4, 135.1, 133.7, 132.5, 127.8, 125.2, 124.8, 123.9, 55.04; LC-MS (m/z): (M)+, 326; Elemental analysis calculation for C15H12FN7O (%): C, 55.38; H, 3.72; N, 30.14; Found: C, 55.35; H, 3.76; N, 30.17.
Results
Antidiabetic essay
Synthesized analogues were checked for their in vivo antidiabetic activity. Plasma glucose at 7th hours for all the synthesized compounds is in the Table I. The compounds which lowered the blood glucose level, their results were compared with glibenclamide, while compounds that led to an increase in blood glucose concentration, their results were compared with diabetic non-treated mice. Graphical representation of some of the representative compounds which decreased the plasma glucose concentration near to glibenclamide is shown in Figure 1.
Figure 1: Plasma glucose concentration in mice treated with synthetic compounds (9a, 9b, 9c, 9i; 10i, 10l) positive control was treated with glibenclamide while the negative control was alloxan only treated
Molecular docking studies
Drug likeness
Several descriptors such as number of rotatable bonds, hydrogen bond donor, hydrogen bond acceptor, Lipinski’s acceptors, Lipinski’s donors, Lipinski’s violation, Lipinski’s drug likeness, logP, molecular weight and total polar surface area (TPSA) were computed to access the drug like properties of the synthesized library acetohydrazides. Computation results revealed that all of them were found to obey the Lipinski’s Ro5 and Veber’s Ro3, cut-off limits, (Lipinski et al., 1997, Veber et al., 2002) revealing the fact that these could be potent antidiabetic compounds.
According to the Lipinski’s Ro5, most drug like molecules should have molecular weight ≤500, logarithm of the octanol/water partition coefficient (log P) ≤ 5, total polar surface area (TPSA) <140 Ǻ, number of hydrogen bond donors (HBD) ≤5 and hydrogen bond acceptor (HBA) ≤10 (Lipinski et al., 1997). Further modifications in the Ro5 were made by another group(Veber et al., 2002) who suggested the number of rotatable bond (NOR) of drug like molecule must be fewer or equal to 10 (Veber et al., 2002). Molecules violating more than one of these criteria could have problem with bioavailability. Figure 2 shows graphical presentation of the descriptors of the 28 synthesized acetohydrazides.
Figure 2: Drug likeness of the synthesized library of acetohydrazides
Docking studies
To develop a deeper insight into the molecular mechanism of inhibition of the synthesized libraries comprising the compounds 9a-9n and 10a-10n were simlated computationally to the active sites of human insulin protein (PDB code: 3Q6E). Human insulin protein consisted of five active sites as shown in Figure 3A. The active sites shown in the figure are marked with alpha centers created by the software.
Figure 3: A) Active site of 3Q6E. Five active sites represented by alpha centers created by site finder module (MOE). B) Active site of human insulin protein (3Q6E). Docked poses of all the most favorable docked conformations of the ligands are shown inside the pocket. Ligands are presented in ball n stick mode while key amino acid residues are shown in elemental color, stick mode. Backbone receptor is presented in cartoon and ribbon form. Active sites are marked with grey and red colored spheres
These sites were found to be very small bowl shaped cavities. In silico molecular docking results, produced the different docking conformations based on binding energy. The variants with the minimal energy of the enzyme–inhibitor complex were selected for studies of binding mode. Preferred docked conformations of most of the ligands formed one cluster inside the active.
All the docked conformations for each compound were analyzed and it was found that the most favorable docking poses with maximum number of interactions were those which were ranked the highest based on the minimal binding energy, which was computed as a negative value by the software. The most favorable docking poses of the 30 docked conformations for each compound were analyzed to further investigate the interactions of the docked conformations within the active sites. The detailed docking results are tabulated in Table I.
Phe1, Gln4, Ser9, His10, Glu13 and Leu17 are found to be key residues which are also reported in the literature (Batool et al., 2015). The active site consisted of mainly hydrophilic amino acids. The hydrophobic portion was constructed of Phe1 and Leu17 while the hydrophilic portion was lined with amino acids, including Gln4, Ser9, His10 and Glu13 which were also the prominent interacting residues.
Oxygen atom present in the template formulated strong interaction with Ser9 and His10, while phenyl and other cyclic moieties containing π electrons established π-H interactions with His10 in ligands 9a, 9c-9f, 9h, 9i and 9k-9m. Almost all the ligands showed strong polar interactions with Ser9, His10 and Glu13. However, ligand 10f-10h showed π-π interaction. Ligand 10f and 10g showed hydrophobic interaction with Phe1 and Leu17 while ligands 10c, 9b, 9f, 9j and 9m made contact with Leu17 and ligand 9d and 9e showed hydrophobic interaction with Phe1. Ligand 10a and 10l found to be the most potent antidiabetic agents of the series. These both ligands showed a similar binding pattern and anchored tightly inside the active site gorge (Site V) of the protein by establishing strong hydrogen bonding with Ser9 (2.73 Å), π-H interaction with His10, in addition to polar interactions with His10, Ser9 and Glu13. Ligand 10l bind itself strongly inside the active site by establishing two deterministic hydrogen bonding with Ser9 and His10 (2.77 Å and 2.30 Å rec.) Moreover, this ligand also showed interaction with His10, Ser9 and Glu13. Figure 4A-D represent the docking pose of the ligand 9a and 10l. It is quite interesting to note that the binding free energy in “S†field is in the quite narrow range from ‒1.60 to ‒3.67 kcal/mol (Table I), similarly binding energy in Escore-1 field (London dG ranging from –7.52 to ‒8.69 kcal/mol (Table I) is also found in very narrow range.
Table I: In vivo antidiabetic activities and binding energies of synthesized compounds
Entry | GC [mg/DL (glucose concentration)] | Binding energy (KJ/mol) | ||||
---|---|---|---|---|---|---|
S Field | London dG | |||||
9 | 10 | 9 | 10 | 9 | 10 | |
a | 60 | 201 | -3.05 | -2.11 | -7.81 | -8.70 |
b | 86 | 135 | -2.80 | -3.37 | -8.64 | -7.65 |
c | 63 | 115 | -3.18 | -2.30 | 8.73 | -8.63 |
d | 92 | 404 | -2.33 | -2.68 | -7.86 | -7.60 |
e | 98 | 132 | -2.64 | -1.95 | -7.77 | -7.94 |
f | 92 | 247 | -1.45 | -2.00 | -7.80 | -7.51 |
g | 203 | 507 | -1.88 | -2.52 | -8.65 | -8.70 |
h | 94 | 255 | -2.66 | -2.21 | -7.92 | -7.48 |
i | 81 | 94 | -2.54 | -1.90 | -8.35 | -8.23 |
j | 487 | 433 | -2.82 | -3.24 | -7.52 | -8.58 |
k | 207 | 101 | -2.98 | -2.38 | -8.69 | -8.08 |
l | 465 | 89 | -3.38 | -2.11 | -7.60 | -7.99 |
m | 279 | 108 | -1.76 | -3.03 | -7.94 | -7.66 |
n | 102 | 209 | -1.96 | -1.58 | -7.52 | -8.88 |
Alloxan | 411 | -1.66 | -5.79 | |||
Glibenclamide | 40 | -1.60 | -6.73 |
Figure 4: Molecular docking analysis of (3QE6). A, C) Docking pose of 9a and 10l in 3D space, molecule is shown in cyan color (ball n stick model) key residues are shown in elemental color (stick mode). The receptor is shown in red ribbons and cartoons. Hydrogen bonding interactions are shown in dashed purple lines. B, D) Docking pose of 9a and 10l in 2D space, hydrogen bonding interactions are shown in green colored dashed lines
Discussion
Synthesis of acetohydrazide derivatives [9(a-n), 10(a-n)] is shown in Scheme 1. Cycloaddition of sodium azide and 3/4-cyanopyridine (1,2) in the presence of zinc chloride in water led to the formation of 3-(2H-tetrazole-5-yl) pyridine (3) and 4-(2H-tetrazole-5-yl) pyridine (4) which are capable of existing in solution as equilibrium mixtures of N1 and N2 tautomers (Nadeem et al., 2014). The position of equilibrium depends significantly on the solvent polarity and the substituents in the tetrazole ring. Less polar solvent and bulky substituent in the tetrazole ring favors N2 tautomer (Katritzky et al., 2010). Next step is the reaction of (3) and (4) with ethyl chloroacetate to give ethyl 2-(5-(pyridin-3-yl)-2H-tetrazol-2-yl)acetate (5) and ethyl 2-(5-(pyridin-4-yl)-2H-tetrazol-2-yl) acetate (6). Normally these reactions are carried out in acetone using K2CO3 which leads to the formation of isomeric esters requiring separation. That is why we used triethyl amine and chloroform which led to the formation of pure N2 tautomer. In FTIR the presence of carbonyl stretches at 1759 and 1761 cm-1 of (5) and (6) respectively, indicates the attachment of ester moiety. This was further confirmed through carbonyl carbon with δ value 166.5, 166.7 in 13C NMR spectrum as well as triplets at 1.32, 1.35 and quartets at 4.22, 4.19 for CH3 and CH2 respectively in 1H NMR spectrum 2-(5-(pyridin-3-yl)-2H-tetrazol-2-yl)aceto-hydrazide (7) and 2-(5-(pyridin-4-yl)-2H-tetrazol-2-yl)acetohydrazide (8) formation from ester (5), (6) was confirmed by the stretching vibrations at 3319, 3320 (NH2), 3134, 3137 (N-H) in FTIR spectrum and broad peaks at 12.02, 12.04 and 4.97, 5.03 in 1H NMR spectra. Finally N΄-(substitutedbenzylidene-2-(5-(pyridin-3-yl)-2H-tetrazol-2-yl)acetohydrazide (9a-n) and N΄-(substituted benzylidene-2-(5-(pyridin-4-yl)-2H-tetrazol-2-yl)acetohydrazide (10a-n) were synthesized by stirring hydrazides (7), (8) and substituted benzaldehydes in dry DMF. In proton NMR spectra, sharp singlets in the range between 8.04-8.28 led to the formation of CH=N bond of acetohydrazides (9a-n) and (10a-n).
The series (9a-n) contain an acetohydrazide moiety linked with 3-pyridine through a tetrazole ring. The compounds (9a) and (9b). With chloro substituent at 4 and 3 position showed decrease in blood glucose and this decrease is more significant in case of (9a) as compared to (9b). While by introducing two chloro groups in case of (9f), we have nearly same results as of (9b). The compound (9c) with m-OH group resulted in a decrease in glucose level as of (9a) and by adding two –OH groups at 2,4-positions (9g), we have an increase in blood glucose level. For the derivatives (9d) and (9e) with respective electron donating and electron withdrawing substituents, there is a decrease in plasma glucose while (9n) with 4-OMe group showed intermediate behavior as compared to the positive control glibenclamide and negative control alloxan. Introduction of bromo group also reduces the glucose concentration and this decrease is independent of the position of substituent. Compounds (9j-m) increased the blood glucose level and this increase is more significant in case of (9j) and (9l) as compared to (9k) and (9m).
The compounds of the series (10a-n) have similar structures as of (9a-n) with the only difference of having 4-pyridinyl instead of 3-pyridinyl moiety. The derivatives (10a,f,n) showed intermediate behavior as compared to both positive and negative control and the compounds (10b,c,e,k) have decreased the glucose level, although not significantly. Introduction of bromo group has interesting effects on blood glucose level. Its presence at ortho position (10h) increases the blood glucose while at meta position (10i), there is significant lowering of blood glucose level. Derivatives (10d,g,j) showed a drastic increase in the glucose concentration. By introducing –F group, we have lowering in blood glucose level and this lowering is more significant in case of (10l) with 3-F as compared to (10m) with 2-F substituent.
All compounds obeyed drug likeness criteria and docking studies showed that almost all the compounds exhibited a similar binding pattern against 3Q6E. Binding mode analyses revealed that the ligands showed two types of docking orientations. Most of the ligands attained a twisted shape like a semi square and fit inside the small cavity (Figure 3B) while few attained extended position and their docking poses found to be anchored inside the two adjacent active sites.
The ligands 9a, 9b, 9f-10b, 10e, 23, 10i-10n showed similar orientation inside the cavities and found to fit completely inside the Site V. Due to the flexible linker between the two terminals of the template, ligands somehow managed to attain semi squared shape which paved the way for them, to enter inside the small cavity of the Site V of the protein. Ligands 9c, 9e and 10f showed similar binding pattern inside the active site, however different from the above said ligands. These ligands were docked in extended form. One end of the molecule managed to bind inside the site V while the rest of the molecule extended to site II of the protein. These ligands are shown in blue color in Figure 3B, ligand 10j found to attain little different binding mode from that of ligand 9c, 9e, 10f. However, it also docked inside the site V in addition to site II. It is shown in red color in Figure 3B. Finally, it could be concluded from the current computations, that good activities of these inhibitors, which are noticed to be more potent than standard drug alloxan can be accredited to quite low binding energy (Table I) and multiple interactions with the key residues inside the active site of the target. This may provide a rationale for more effective binding through various interactions with the enzyme’s active site. The binding energies derived from docking simulations indicated that almost all the members of the synthesized series exhibited stronger binding affinity for 3Q6E. The synthesized ligands showed better binding free energy and binding energy in Escore-1 field as compared to both the standard drugs, alloxan (-1.60 and -5.79 kcal/mol) and glibenclamide (-1.60 and -6.73 kcal/mol).
In case of compounds 9a-9f, 9h, 9i, 9n, 10b, 10i and 10k-10m experimental screening results approve that of the docking computation and this rationale could be used as a strong evidence for further development of these compounds as useful antidiabetic agents. Compound 9a and 10l can serve as potential surrogates for further studies. These results complement biological studies providing additional information about possibilities for the development of the novel antidiabetic drugs.
Conclusion
Among the two new series of tetrazolopyridine-acetohydrazide conjugates synthesized, compounds 9a, 9c and 10l lowered the plasma glucose near to that of glibenclamide. Molecular docking studies and results of antidiabetic activities were in agreement with each other.
Ethical Issue
Animal handling and experimental procedures were carried out according to the guidelines provided by the Ethics Committee of the Department of Animal Sciences on care and use of animals for scientific research.
Acknowledgements
The authors are thankful for the financial support provided by higher education commission Pakistan. One of the authors (Farukh Jabeen) is thankful to Dr. Charles Dennis Hall, University of Florida, USA for the MOE software.
References
Abd El-Salam OI, Mohamed SF. Synthesis and preliminary antiâ€bacterial activity of some 4â€substitutedâ€N1â€2â€pyridylsul-fanilamide derivatives. J Chin Chem Soc. 2005; 52: 1157-64.
Ahmad M, Siddiqui HL, Zia-ur-Rehman M, Parvez M. Antioxidant and antibacterial activities of novel N′-aryl-methylidene-2-(3, 4-dimethyl-5, 5-dioxidopyrazolo [4, 3-c][1, 2] benzothiazin-2 (4H)-yl) acetohydrazides. Eur J Medi Chem. 2010; 45: 698–704.
Ali MK, Narayan KV, Tandon N. Diabetes and coronary heart disease: Current perspectives. Ind J Med Res. 2010; 132: 584.
Al-Omar MA, Amr AE, Al-Salahi RA. Antiâ€inflammatory, analgesic, anticonvulsant and antiparkinsonian activities of some pyridine derivatives using 2,6â€disubstituted isonicotinic acid hydrazides. Arch Pharm Chem Life Sci. 2010; 10: 648–56.
Arafa RK, Hegazy GH, Piazza GA, Abadi SH. Synthesis and in vitro antiproliferative effect of novel quinoline-based potential anti-cancer agents. Eur J Med Chem. 2013; 63: 826-32.
Batool I, Saeed A, Qureshi IZ, Kalsoom S, Razzaq A. Synthesis, molecular docking and biological evaluation of new thiazolopyrimidine carboxylates as potential antidiabetic and antibacterial agents. Res Chem Intermed. 2015; 1164: 2078-82.
Bayer H, Batzl C, Hartmann RW, Mannschreckt A. New aromatase inhibitors: Synthesis and biological activity of pyridyl-substituted tetralone derivatives. J Med Chem. 1991; 34: 2685-91.
Bepary S, Das BK, Bachar SC, Kundu JK, Rouf ASS, Datta BK. Anti-inflammatory activity of indanyltetrazole derivatives. Pakistan J Pharm Sci. 2008; 21: 295-98.
Bhaskar VH, Mohite PB. Synthesis, characterization and qsar study of some substituted n'-[arylidene]-2-(5-phenyl-1h-tetrazol-1-yl) acetohydrazide. Dig J Nanomater Bios. 2010; 5: 177-83.
Bradbury RH, Allott CP, Dennis M, Girdwood JAlan, Kenny PW, Major JS, Oldham AA, Ratcliffe AH, Rivett JE, Roberts DA, Robins PJ. New nonpeptide angiotensin II receptor antagonists. 3. Synthesis, biological properties, and structure-activity relationships of 2-alkyl-4-(biphenylylmethoxy) pyridine derivatives. J Med Chem. 1993; 36: 1245-54.
Cheng J, Xie J, Luo X. Synthesis and antiviral activity against Coxsackie virus B3 of some novel benzimidazole derivatives. Bioorg Med Chem Lett. 2005; 15: 267-69.
Chinai JM, Taylor AB, Hargreaves ND, Ryno LM, Morris CA, Hart PJ. Urbach, Human insulin in complex with cucurbit[7]uril (2010 Released: 2011-04-20).
Demko ZP, Sharpless KB. Preparation of 5-substituted 1 H-tetrazoles from nitriles in water. J Org Chem. 2001; 66: 7945-50.
El-Sayed WA, Abdel Megeid RE, Abbas H-Allah S. Synthesis and antimicrobial activity of new 1-[(tetrazol-5-yl) methyl] indole derivatives, their 1, 2, 4-triazole thioglycosides and acyclic analogs. Arch Pharm Res. 2011; 34: 1085-96.
Gao XL, Zhao Gl, Liu W, Shao H, Wang YL, Xu WR, Tang LD, Wang JW. Design, synthesis and in vivo hypoglycemic activity of tetrazole-bearing N-glycosides as SGLT2 inhibitors. Indian J Chem. 2010; 49B: 1499-508.
Halgren TA, Nachbar RB. Merck molecular force field. IV. Conformational energies and geometries for MMFF94. J Comput Chem. 1996; 17: 587-615.
Hart B, Bierer DE, Zhang C. Substituted 3-pyridyl oxazoles as c17, 20 lyase inhibitors. 2004; US Patent; 0209924:A1.
Holland GF. Lipid regulation with 5-(pyridyl) tetrazole derivatives. 1969; Conn. US. Cl; 424-263.
Huang D, Poon SF, Chapman DF, Chung J, Cramer M, Reger TS, Roppe JR, Tehrani L, Cosford NDP, Smith ND. 2-{2-[3-(Pyridin-3-yloxy) phenyl]-2H-tetrazol-5-yl} pyridine: A highly potent, orally active, metabotropic glutamate subtype 5 (mGlu5) receptor antagonist. Bioorg Med Chem Lett. 2004; 14: 5473–76.
Iso Y, Grajkowska E, Wroblewski JT, Davis J, Goeders NE, Johnson KM, Sanker S, Roth BL, Tueckmantel W, Kozikowski AP. Synthesis and structure-activity relationships of 3-[(2-methyl-1, 3-thiazol-4-yl) ethynyl] pyridine analogues as potent, noncompetitive metabotropic glutamate receptor subtype 5 antagonists: Search for cocaine medications. J Med Chem. 2006; 49: 1080-100.
Katritzky HAR, El-Gendy BEM, Draghici B, Dennis C, Steel PJ. NMR Study of the tautomeric behavior of N-(α-aminoalkyl) tetrazoles. J Org Chem. 2010; 75: 6468-76.
Kumar D, Lal R, Rani S. Synthesis of some new substituted azetidinonyl and thiazolidinonyl quinazolon-4 (3H)-ones as potential non-steroidal anti-inflammatory and analgesic agents. Int J Innov App Stud. 2014; 8: 1798-813.
Lacerda RB, Sales NM, Silva LLda, Tesch R, Miranda ALP, Barreiro EJ, Fernandes PD, Fraga CAM. Novel potent imidazo [1, 2-a] pyridine-N-glycinyl-hydrazone inhibitors of TNF-α production: In vitro and in vivo studies. PLoS One 2014; 9: e 91660.
Li FL, Song HB, Dai B, Tang LF. Synthesis, structure and biological activity of diorganotin derivatives with pyridyl functionalized bis (pyrazolâ€1â€yl)methanes. Appl Organometal Chem. 2010; 24: 669–74.
Lipinski CA, Lombardo F, Dominy BW, Feeney PJ. Experimental and computational approaches to estimate solubility in drug discovery and development. Adv Drug Deliv Rev. 1997; 23: 3-25.
Maione S, E Morera E, Marabese I, Ligresti, A, Luongo L, Ortar G, Marzo VDi. Antinociceptive effects of tetrazole inhibitors of endocannabinoid inactivation: Cannabinoid and nonâ€cannabinoid receptorâ€mediated mechanisms. Brit J Pharmacol. 2008; 155: 775–82.
Manna K, Agrawal YK. Microwave assisted synthesis of new indophenazine 1, 3, 5-trisubstruted pyrazoline derivatives of benzofuran and their antimicrobial activity. Bioorg Med Chem Lett. 2009; 19: 2688–92.
Maxwell JR, Wasdahl DA, Wolfson AC, Stenberg VI. Synthesis of 5-aryl-2H-tetrazoles, 5-aryl-2H-tetrazole-2-acetic acids, and [(4-phenyl-5-aryl-4H-1, 2, 4-triazol-3-yl) thio] acetic acids as possible superoxide scavengers and anti-inflammatory agents. J Med Chem. 1984; 27: 1565-70.
Miwatashi S, Arikawa Y, Naruo K-ichi, Igaki K, Watanabe Y, Kimura H, Kawamoto T, Ohkawa S. Synthesis and biological activities of 4-phenyl-5-pyridyl-1, 3-thiazole derivatives as p38 MAP kinase inhibitors. Cliem Pharm Bull. 2005; 53: 410-18.
Mohammad SY, Mohammad WA. Synthesis and anticonvulsant activity of substituted oxadiazole and thiadiazole derivatives. Acta Pol Pham Drug Res. 2009; 66: 393.
MOE (Molecular operating environment) 2014. 08; chemical computing group inc., 1010 sherbooke St. west, suite 910, montreal, QC, Canada, H3A 2R7, 2014.
Muir CW, Kennedy AR, Redmond JMW, Watson A. Synthesis of functionalised 4H-quinolizin-4-ones via tandem Horner–Wadsworth–Emmons olefination/cyclisation. J Org Biomol Chem. 2013; 11: 3337-40.
Nadeem S, Aziz-ur-Rehman, Abbasi MA, Siddiqui SZ, Rasool S, Ahmad I, Afzal S. Antibacterial and enzyme inhibition studies of N'-substituted benzylidene-2-(2,4-dimethylphenoxy) acetatohydrazides. Pak J Chem. 2014; 4: 96-100.
Nohara A, Kurik H, Saijo T, Sugihara H, Kanno M and Sanno Y. Studies on antianaphylactic agents: 5. Synthesis of 3-(1H-tetrazol-5-yl) chromones, a new series of antiallergic substances. J Med Chem. 1977; 20: 141-45.
Qureshi IZ, Abbas Q. Modulation of testicular and whole blood trace element concentrations in conjunction with testosterone release following kisspeptin administration in male rabbits (Oryctolagus cuniculus). Biol Trace Elem Res. 2013; 154: 210-16.
Rabea SM, El-Koussi NA, Hassan HY, Aboul-Fadl T. Synthesis of 5â€Phenylâ€1â€(3â€pyridyl)â€1Hâ€1, 2, 4â€triazoleâ€3â€carboxylic acid derivatives of potential antiâ€inflammatory activity. Arch Pharm Chem Life Sci. 2006; 339: 32–40.
Saeed A, Hussain M, Qasim M. Novel N-acyl/aroyl-2-(5-phenyl-2H-tetrazol-2-yl) acetohydrazides: Synthesis and characterization. Turk J Chem. 2014; 38: 436-42.
Tomi IHR, Al-Daraji AHR, Al-Qaysi RRT, Hasson MM, Al-Dulaimy KHD. Synthesis, characterization and biological activities of some azo derivatives of aminothiadiazole derived from nicotinic and isonicotinic acids. Arabian J Chem. 2014; 7: 687–94.
Veber DF, Johnson SR, Cheng HY, Smith BR, Ward KW, Kopple KD. Molecular properties that influence the oral bioavailability of drug candidates. J Med Chem. 2002; 45: 2615-23.
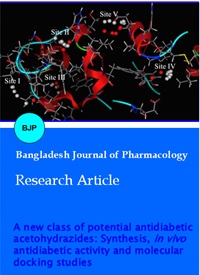