Stellaria media attenuates the hyperglycemia and hyperlipidemia in alloxan-induced diabetic rat
Abstract
The aim of this research work was to assess the hyperglycemic and hyperlipidemic effects of Stellaria media in alloxan-induced diabetic rat using different experimental models. Standard documented protocols were used to concede the in vitro and in vivo activities. The results showed pancreatic α-amylase and β-glucosidase inhibition in vitro at varying concentrations of the extract which further validated the in vivo anti-diabetic action of the plant. The administration of various concentrations of the extract showed decrease in fasting blood level when compared to diabetic control. Similarly, decreased HbA1c (−48.4%) were observed when compared to diabetic control rat. The extract also caused reduced serum transaminase and produced a succeeding recovery toward their normal values. In conclusion, the in vitro and in vivo hypoglycemic and hypolipidemic activity offer the methodical justification for the use of S. media in herb based anti-diabetic treatment.
Introduction
Diabetes mellitus is an endocrine abnormalities having numerous etiologies, linking to lipids, protein and carbohydrate metabolic disorders that usually affect the quality of life. Insulin deficiency and insulin resistance are considered to be responsible for all types of diabetes including type 1 and 2 (Narendhirakannan et al., 2006).
The basic aim of all diabetes management strategies is to control and sustain satisfactory blood glucose levels. Oral hypoglycemic drugs and recombinant insulin are being used for the treatment of diabetes. The inhibition of carbohydrate hydrolyzing enzymes is helpful is reducing postprandial blood glucose levels (Tsujita et al., 2008). The two main carbohydrate hydrolyzing enzymes responsible for the breakdown of dietary polysaccharides are α-amylase and α-glucosidase (Daisy et al., 2009). The first step in digestion of dietary starch is catalyzed by the pancreatic α-amylase, which converts the starch into a mixture of small oligosaccharides. After this step, α-glucosidase further degrades the oligosaccharides into glucose. This glucose then diffuses through the intestine wall into the blood stream, increasing postprandial blood glucose levels.
The orally available medicines use is generally associated with the adverse effects together with hematological, cutaneous and gastrointestinal reactions, interruption in kidney and liver functioning and hypoglycemic coma (Gandhi et al., 2011). Therefore, it is crucial to continue the quest for newer and effective drugs.
A number of researchers have discovered the anti-diabetic potential of flavonoids, cumarins, terpenoids, and other secondary plant metabolites, together with arginine and glutamic acid (Tsujita et al., 2008). Various medicinal plants including Allium cepa (Mathew and Augusti, 1975), Allium sativum (Eidi et al., 2006), Artimisia pallens (Subramoniam et al., 1996), Bidens pilosa (Hsu et al., 2009), Bixa Orellana (Russell et al., 2008), Carum carvi (Haidari et al., 2011), Cassia auriculata (Pari and Latha, 2002), Cassia elata (Palanichamy et al., 1988), Cinnamomum zeylanicum (Verspohl et al., 2005), Coiandrum sativum (Gray and Flatt, 1999), Croton cajucara (Farias et al., 1997), Eclipta alba (Ananthi et al., 2003), Embellica officinalis (D'souza et al., 2014), Eruca sativa (Kishore et al., 2017), Gaultheria trichophylla (Alam and Saqib, 2017), Musa sapientum (Pari and Maheswari, 1999) and Teramus labialus (Fort et al., 2000) have shown anti-diabetic activity.
Stellaria media belongs to family Caryophyllaceous which is a major dicot family of angiosperms powered by 85 genera and 2630 species (KhareIndian, 2007). S. media or chick weed is known as a noxious weed of the agricultural land. The whole plant is used as an anti-rheumatic, anti-inflammatory, astringent, refrigerant, demulcent, emollient, vulnerary, antipruritic, infusion used to relieve in itching and to cure psoriasis. The whole plant is applied as a plaster for broken bones and swellings (Shinwari and Khan, 2000). The current work was conceded to study the possible hypoglycemic and hypolipidemic effects of the plant extract in alloxan-induced diabetic rats both in vitro and in vivo experiments.
Materials and Methods
Reagents and chemicals
The chemicals and standards used in the study were of analytical grade and were obtained from the Sigma. The anti-diabetic drug acarbose (Bayer, Pakistan) was obtained from the local market. HbA1c and hemoglobin tests were executed at the KGN teaching hospital laboratory. The fasting blood glucose sugar was measured by Abbott Glucometer.
Experimental animals
Male Wister rats having age 8-10 weeks and bearing weight 180-260 g were obtained from the NIH Islamabad, Pakistan. The rats were retained in an well-ventilated room (controlled temperature and 12 hours light-dark cycle) in cages with free access to food and water.
Box 1: α-Amylase inhibitory activity
Principle
The method is based on quantifying the reducing sugar (maltose equivalent) liberated. The enzyme inhibitory activity is expressed as a decrease in units of maltose liberated. One unit will liberate 1 mg of maltose from starch in 3 min at pH 6.9 at 20°C.
α-amylase
Starch + water Reducing sugar (maltose)
Requirements
Porcine pancreatic α-amylase (Sigma-Aldrich); 3,5-dinitrosalicylic acid; Electronic balance; Stellaria media extract; Micropipette with tips; Mortar and pestle; Potassium sodium tartrate; Sodium phosphate; Starch; Test tube
Preparation of solutions
Dilution of plant extract: Five different concentrations (100, 200, 300, 400 and 500 µg/mL) of Stellaria media extract as well as acarbose (standard; different concentrations) were prepared
Starch solution (1%): Weigh 1 g of starch and dissolve in 100 mL of buffer solution
α-Amylase solution (0.5 mg/mL): Weigh 500 mg of α-amylase and dissolve in 0.2M sodium phosphate buffer at pH 6.9
3,5-Dinitrosalicylic acid reagent (96 mM): Weigh 219 mg in 10 mL of distilled water by dissolving the powder by heating on a heating/stir plate with mixing. The solution should not heat to boiling point.
Color reagent solution (40 mL): Warm (50-70°C) ultrapure water (12.0 mL) to a 50 mL amber bottle. With mixing, slowly add a) warm potassium sodium tartrate, tetrahydrate solution (5.3 M; 8 mL) and b) warm 3,5-dinitrosalicylic acid solution (96 mM; 20 mL)
Procedure
Step 1: α-Amylase solution (200 μL) was mixed with different concentrations of plant extract (200 μL). A control test tube was also prepared which was devoid of both plant as well as standard sample
Step 2: The mixed solution was pre-incubated at room temperature for 10 min
Step 3: Following pre-incubation, starch solution (200 μL) was added to each tube
Step 4: Incubated for another 3 min
Step 5: The reaction was stopped by the addition of 3,5-dinitrosalicylic acid color reagent (200 μL)
Step 6: The contents were heated in a water bath at 85-90°C for 10 min
Step 7: The mixture was allowed to cool to ambient temperature
Step 8: The test tubes were diluted with 5 mL of distilled water
Step 9: By using UV-Visible spectrophotometer, the absorbance was taken at 540 nm
Calculation
The % inhibition of α-amylase was calculated by the following formula:
[(OD control− OD sample)/ OD control] × 100
The same procedure was repeated for standard acarbose as well
Notes
Color reagent solution is stable for 6 months at ambient temperature if protected from light
Reference
Miller, 1959
Plant collection and extraction
The S. media plant was collected locally from the District Bannu-KPK and was identified and authenticated by a taxonomist at the Department of Botany, UST Bannu-KPK. The leaves of the plant were taken, washed and shade dried. After 25 days, the dried leaves were powered using a blender. 200 g of powder was macerated in 1L ethanol for 2 weeks following constant shaking and stirring using a shaker machine. After 2 weeks, the extract was filtrated. The filtrate was concentrated using a rotary evaporator until the removal of extraction solvent. The dark green product was subjected to lyophilizer for getting a fine powder which was stored at 4°C for future use.
In vitro anti-diabetic assay
β-Glucosidase inhibitory activity
The β-glucosidase inhibitory activity of the plant extract was determined by using a well-established protocol(Krishnaveni et al., 1984) with some alterations. 290 mM β-D glucopyranoside (pNPGlc) solution was prepared in 20 mM citrate buffer having a pH 5.6. Five concentrations of the plant extract and acarbose were prepared. 200 µL of plant extract or standard was taken from each concentration and mixed with 980 µL of pNPG and incubated at 37°C for 5 min. About 20 µL of enzyme β-glucosidase (IU/mL) was added to the above mentioned each mixture and further incubated at 35°C for 40 min in order to stop the reaction, 200 µL 6N-HCl was added to the reaction mixture and the absorbance was taken at 405 nm. The experiment was performed in duplicate. Water was taken as a reference. The %inhibition was calculated using the equation:
%Inhibition = (Ac-As)/Ac × 100
Where, Ac is enzyme activity of standard and As is enzyme activity of AgNPs
In vivo anti-diabetic assay
Diabetes induction in rats
After an overnight starvation, diabetes was impelled by a single intraperitoneal injection of alloxan at a dose of 150 mg/kg body weight. The control animals received normal saline instead of alloxan in an equivalent amount. Since alloxan is capable to cause lethal hypoglycemia because of huge pancreatic insulin release, the animals were provided with 10% glucose solution just after 6 hours of alloxan administration for the next 24 hours in order to overcome drug-induced hypoglycemia. After 48 hours, the fasting blood glucose was measured to assess the induction of diabetes. The animals with blood glucose level >200 mg/dL were considered as diabetic and were further selected for other experimental procedures.
Experimental design
In the test group, 42 rats were used (30 alloxan-induced diabetic rats; 6 normal control rats and 6 normal extract rats). The groups were arranged as follow: a) Control rats; b) alloxan-induced diabetic rats; c) diabetic rats treated with S. media leaf extract (100, 250 or 400 mg/kg/day) in saline intraperitoneally for 21 days; d) diabetic rats treated with glibenclamide (5 mg/kg/day) in saline intraperitoneally for 21 days; e) normal, non-diabetic rats treated with S. media leaf extract (250 mg/kg/day) in saline intraperitoneally for 21 days.
The body weight of the rats was measured at day 0 and 21 whereas, the blood glucose level of all rats was measured at fixed interims (0, 3, 7, 14 and 21 days). At day 21, the food was withhold and the rats were starved overnight. Following starvation, the animals were anesthetized and decapitated. The blood was congregated with and without anticoagulant for plasma and serum portion respectively. The liver of animals of various groups (control, diabetic and treated) was preserved for TBARS assay.
Acute toxicity study
Wister rats were clustered into vehicle-treated ‘control’ and drug-treated ‘test’ groups, making up of seven sets of six rats each. The plant extract (100, 150, 250, 500, 1000, 1500 or 2000 mg/kg) was alone injected through intraperitoneal membrane to the rats in each of the test groups. Every rat in the control group was treated with normal saline only. The animals were studied continuously for 2 hours under the following profiles: a) Behavioral profile including mood alteration, agitation, and irritability; b) neurological profile including touch reaction, pain response, and posture; c) autonomic profile including excretion and urination.
After 24 hours, they were observed for any death or lethality.â€
Blood biochemistry
Using a glucometer, blood glucose measurement was taken at different time intervals i.e. 0, 3, 7, 14 and 21 days just after administration of extract on daily basis.
After blood glucose valuation on day 21st, the whole blood was taken by cardiac rupture under slight anesthetic conditions and further glycated hemoglobin and hemoglobin levels were assessed in normal and alloxan induced diabetic rats in laboratory on an automated hematology analyzer (Sysmex, USA).
Isolation of liver
Soon after collecting blood, the rats were killed by cervical decapitation. The liver was isolated and washed. The liver was sliced and homogenized in 10 mM Tris-HCl buffer (1/10, w/v) having pH 7.5.
TBARS assay
To assess the lipid peroxidation, TBARS assay was performed according to the protocol published elsewhere (Okhawa et al., 1979; Puntel et al., 2007). The homogenate was centrifuged at 4,000 x g for 10 min to yield a pellet that was discarded and the supernatant was used for determining MDA content. 100 µL low speed supernatant was incubated for 60 min at 37°C in the presence or absence of extract. After incubation, TBA, 8.1% SDS and acetate buffer was added and again incubated for 60 min at 100°C. The production of light pink color showed the reaction of MDA with TBA. By cooling the tubes using ice, absorbance was measured at 532 nm using spectrophotometer.
Statistical analysis
Results were measured as mean ± SD for 6 rats in an individual group. Statistical evaluation of data was done using SPSS, version 20.0. A p value of <0.05 was considered statistically significant.
Results
α-Amylase inhibitory activity
In the Table I, the results of α-amylase inhibitory action by the plant extract are mentioned. Ever since, acarbose is considered as reference, its inhibitory activity has been considered as 100%. Based on that, the relative inhibitory activity has been calculated.â€
Table I: Inhibitory activity of α-amylase and β-glucosidase for extract and standard at different concentrations
Concentration (µg/mL) | Acarbose | α-Amylase %inhibition (extract) | β-Glucosidase % inhibition (extract) |
---|---|---|---|
100 | 68 ± 0.20 | 48.1 ± 0.30 | 50.1 ± 0.20 |
200 | 72.2 ± 0.86 | 55.5 ± 0.62a | 52.8 ± 0.42b |
300 | 77.4 ± 1.05 | 60.2 ± 0.60b | 58.4 ± 0.40a |
400 | 80.2 ± 0.82 | 72.6 ± 0.62a | 76.8 ± 0.52b |
500 | 88.6 ± 0.22 | 74.6 ± 0.44a | 80.4 ± 0.44b |
Values are mean ± SEM, n = 6, when compared with diabetic control by using one multiple comparison test, ap<0.05; bp<0.01 |
β-glucosidase inhibitory activity
Table I shows the β-glucosidase inhibitory action by the plant extract. Ever since, acarbose is considered as reference, its inhibitory activity has been considered as 100%. Based on that, the relative inhibitory activity has been calculated.â€
Acute toxicity study
The results of the acute toxicity study showed that the extract S. media didn’t show any modification in the behavior of the test animals. The extract-treated rat did not show any mortality. There was no lethal reaction or toxicity found till 2,000 mg/kg dosage. This shows the approximate LD50 above 2,000 mg/kg.
Effects of extract on blood glucose levels
Alloxan-induced diabetic rat showed a remarkable upswing in the blood glucose level when compared to the normal rats. Administration of extract (400 mg/kg body weight) caused a momentous decrease in fasting blood level when compared to diabetic control (Table II).
Table II: Fasting blood glucose, hemoglobin and HbA1c readings of control and test groups
Groups | Fasting blood glucose (mg/dL) | Hemoglobin (g/dL) | HbA1c (%) | ||||
---|---|---|---|---|---|---|---|
Day 0 | Day 3 | Day 7 | Day 14 | Day 21 | |||
Normal control | 94.2 ± 4.1a | 96.3 ± 6.0 | 97.2 ± 4.4 | 88.2 ± 6.0 | 87.4 ± 6.9 | 13.4 ± 1.0 | 4.8 ± 0.2 |
Normal control + S. media 250 mg/kg | 92.0 ± 4.2 | 92.6 ± 6.1 | 88.8 ± 5.4 | 87.0 ± 8.8 | 84.2 ± 8.0a | 13.0 ± 1.0a | 5.0 ± 0.4s |
Diabetic control | 286.8 ± 8.8a | 308.6 ± 10a | 348.8 ± 12a | 378.8 ± 15a | 396.6 ± 12a | 9.8 ± 1.4 | 8.2 ± 0.6 |
Diabetic + S. media 100 mg/kg | 286.3 ± 8.1 | 274.2 ± 4.0 | 250.2 ± 6.2 | 230.4 ± 6.0a | 218.0 ± 6.2a | 12.2 ± 1.2a | 7.5 ± 0.2 |
Diabetic + S. media 250 mg/kg | 289.8 ± 7.2 | 260.4 ±5.0 | 230.4 ± 4.0a | 171.5 ± 6.5a | 150.2 ± 3.0a | 11.8 ± 1.8 | 6.2 ± 0.4 |
Diabetic + S. media 400 mg/kg | 288.2 ± 6.8 | 264.8 ± 5.4 | 222.2 ± 7.5a | 155.5 ± 10a | 120.8 ± 8.2a | 13.0 ± 1.2a | 5.5 ± 0.6a |
Diabetic + Glibenclamide 5 mg/kg | 288.4 ± 6.6 | 248.1 ± 8.4 | 210.4 ± 8.0 | 144.4 ± 8.8 | 114.28 ± 7.3a | 13.2 ± 1.4a | 5.4 ± 0.2a |
Values are mean ± SEM, n = 6, when compared with diabetic control by using one multiple comparisonâ€test. ap<0.05 |
Effects on hemoglobin and HbA1c
Table II also shows the effect of S. media on hemoglobin and glycosylated hemoglobin levels in normal and alloxan-induced diabetic rats. A significant reduction in hemoglobin (-30.3%) and an upsurge in HbA1c (+82.5%) was observed in diabetic animals when compared to normal control group. Administration of S. media remarkably improved hemoglobin (+20.1%), and decreased HbA1c (−48.4%) when compared to diabetic control rats.
Effect of extract on Liver function tests
Alloxan induced damage in liver cells of the diabetic rats and resulted in an increased serum level of ALT, ALP and bilirubin, in comparison to control group (Table III). The levels were normalized significantly with an administration of extract at a concentration 200 and 400 mg/kg body weight through oral route. Treatment with glibenclamide 5 mg/kg body weight following 48 hours of diabetes induction also displayed parallel effect to that of extract (200 mg/kg body weight) in diminishing alloxan intoxication in rats.
Table III: Effects of extract on liver function tests
Groups | ALT (U/L) | ALP (U/L) | Bilirubin (mg/dL) |
---|---|---|---|
Normal control | 30 ± 6.40a | 130.8 ± 1.0 | 0.70 ± 6.0 |
Normal Control + S. media 250 mg/kg | 26 ± 6.40a | 128.4 ± 1.0a | 0.50 ± 6.0a |
Diabetic Control | 56 ± 6.40b | 265.8 ± 1.0b | 2.18 ± 6.0 |
Diabetic + S. media 100 mg/kg | 42 ± 6.40 | 188.2 ± 1.0b | 0.9 ± 6.0b |
Diabetic + S. media 200 mg/kg | 38 ± 6.40a | 148.6 ± 1.0a | 0.8 ± 6.0a |
Diabetic + S. media 400 mg/kg | 26 ± 6.40a | 130.5 ± 1.0a | 0.8 ± 6.0a |
Diabetic + Glibenclamide 5 mg/kg | 28 ± 6.40a | 118.8 ± 1.0a | 0.62 ± 6.0b |
Values are mean ± SEM, n = 6, when compared with diabetic control by using one multiple comparison, ap<0.05; bp<0.01 |
TBARS assay
The alloxan-induced diabetes caused an increased level of TBARS both in plasma and liver in comparison to extract treated group (Figure 1).
Figure 1: (B) TBARS levels in liver tissues of various experimental groups. ap<0.001 compared to control group; bp<0.001 compared to diabetic group
Discussion
The results of our study showed high blood glucose level in alloxan induced diabetic rats and its probable reason is the destructive nature of alloxan on β-cells. Upon intraperitoneal administration of S. media extract (250, 400 mg/kg) and glibenclamide to the diabetic rats markedly decreased blood glucose level. These results were observed from first to fourth week of the study period in comparison to diabetic control rats. Therefore, it might be presumed that antihyperglycemic nature of S. media is due to its protective nature against alloxan induced β-cell devastation and imaginable renaissance of impaired β-cells or an upsurge in insulin secretion or its actions.
Another important clinical marker in diabetes is the glycosylated hemoglobin. It helps to regulate the degree of protein glycation during diabetes mellitus (Aboonabi et al., 2014). In insistent hyperglycemia, formation of HbA1c takes place by non-enzymatic reaction between free amino groups of hemoglobin and glucose. In diabetes mellitus, HbA1c level are a helping hand to assess long-term glycemic control, and to assess the danger of the progression or development of complications associated with diabetes. In alloxan induced diabetic animals, marked decrease in hemoglobin levels and an increase in HbA1c levels were noticed in comparison to control group. S. media treatment demonstrated a reduction of HbA1c and improvement in hemoglobin levels, and it might be due to blood glucose dropping effects of S. media conceivably through setback of insulin resistance or increasing insulin secretion by rejuvenation of pancreatic β-cells.
α-Amylase and β-glucosidase are the two principal enzymes for carbohydrate metabolism present in the small intestine which acts to convert utilized polysaccharides to monosaccharides. The enzymes action results in an increase in postprandial blood glucose level because of the absorption of synthesized glucose from polysaccharides in the small intestine(Mohammadi and Naik, 2008). Drugs possessing an inhibitory act on both α-amylase and β-glucosidase, keep a capability to control postprandial blood glucose levels. Acarbose and miglitol are currently available drugs in this category that inhibit above enzymes competitively. But the drugs possess common side effects including abdominal bloating and flatulence (Atmani et al., 2009; Djeridane et al., 2006; Maksimovic et al., 2005; Mburu et al., 2007). The current investigation showed that S. media possesses strong pancreatic α-amylase and β-glucosidase inhibition which further validated the in vivo anti-diabetic action of the plant because of the inhibition of the above enzymes.
Diabetes mellitus is characterized principally by two features, decreased insulin secretion from pancreatic β-cells and peripheral insulin resistance. Commonly observed sites for insulin resistance are liver, peripheral tissues, skeletal muscle, and adipose tissues. Drug which reduces insulin resistance can efficiently regulate the hyperglycemic state; can normalize lipid metabolism and diabetes-mediated cardiovascular complications (Diouf et al., 2006).
The induction of diabetes with alloxan caused liver damage which is reflected by a momentous upsurge in ALT, ALP, and bilirubin, in comparison with the normal control. The high content of bilirubin is typically a sign of biliary impediment, hemolysis, and renal failure in selected cases (Eidi et al., 2012; Arsad et al., 2014). However, administration of extract reduced serum enzyme levels and produced a succeeding recovery toward normal values. This result indicates an improved hepatoprotective potential of extract.
Lipid peroxidation is a characteristic feature of diabetes as increased lipid peroxidation causes oxidative stress by increasing hydroxyl and peroxy radicals (Motilla et al., 1998). Increased MDA and TBARS levels in diabetic animal’s liver and plasma is due to an enhanced lipid peroxidation. The administration of the extract ameliorated the harmful effects of diabetes in treated animal groups.
Conclusion
S. media in vitro and in vivo hypoglycemic activity provides the methodical justification for its use in herbal anti-diabetic therapy.
Ethical Issue
The protocol of the study was in accordance with the guidelines of the Brazilian association for laboratory animal science (COBEA).
References
Aboonabi A, Rahmat A, Othman F. Antioxidant effect of pomegranate against streptozotocin-nicotinamide generated oxidative stress induced diabetic rats. Toxicol Rep. 2014; 1: 915-22.
Alam F, Saqib Q. Antidiabetic potential of Gaultheria trichophylla in mice. Bangladesh J Pharmacol. 2017; 12: 292-98.
Ananthi J, Prakasam A, Pugalendi KV. Antihyperglycemic activity of Eclipta alba leaf on alloxan-induced diabetic rats. Yale J Biol Med. 2003; 76: 97.
Arsad SS, Esa NM, Hamzah H. Histopathologic changes in liver and kidney tissues from male Sprague Dawley rats treated with Rhaphidophora decursiva (Roxb.) Schott extract. J Cytol Histol. 2014; S4: 001
Atmani D, Chaher N, Berboucha M, Ayouni K, Lounis H, Boudaoud H, Debbache N, Atmani D. Antioxidant capacity and phenol content of selected Algerian medicinal plants. Food Chem. 2009; 112: 303-09.
Daisy P, Eliza J, Farook K. A novel dihydroxy gymnemic triacetate isolated from Gymnema sylvestre possessing normoglycemic and hypolipidemic activity on STZ-induced diabetic rats. J Ethnopharmacol. 2009; 126: 339-44.
Diouf PN, Merlin A, Perrin D. Antioxidant properties of wood extracts and colour stability of woods. Ann For Sci. 2006; 63: 525-34.
Djeridane A, Yousfi M, Nadjemi B, Boutassouna D, Stocker P, Vidal N. Antioxidant activity of some Algerian medicinal plants extracts containing phenolic compounds. Food Chem. 2006; 97: 654-60.
D'souza JJ, D'souza PP, Fazal F, Kumar A, Bhat HP, Baliga MS. Anti-diabetic effects of the Indian indigenous fruit Emblica officinalis Gaertn: Active constituents and modes of action. Food Funct. 2014; 5: 635-44.
Eidi A, Eidi M, Esmaeili E. Antidiabetic effect of garlic (Allium sativum L.) in normal and streptozotocin-induced diabetic rats. Phytomedicine 2006; 13: 624-29.
Eidi A, Mortazavi P, Bazargan M, Zaringhalam J. Hepatoprotective activity of cinnamon ethanolic extract against CCl4-induced liver injury in rats. EXCLI J. 2012; 11: 495-507
Farias RA, Rao VS, Viana GS, Silveira ER, Maciel MA, Pino AC. Hypoglycemic effect of trans-dehydrocrotonin, a nor-clerodane diterpene from Croton cajucara. Planta Medica. 1997; 63: 558-60.
Fort DM, Rao K, Jolad SD, Luo J, Carlson TJ, King SR. Antihyperglycemic activity of Teramnus labialis (Fabaceae). Phytomedicine 2000; 6: 465-67.
Gandhi GR, Ignacimuthu S, Paulraj MG. Solanum torvum Swartz. fruit containing phenolic compounds shows anti-diabetic and antioxidant effects in streptozotocin-induced diabetic rats. Food Chem Toxicol. 2011; 49: 2725-33.
Gray AM, Flatt PR. Insulin-releasing and insulin-like activity of the traditional anti-diabetic plant Coriandrum sativum (coriander). Bri J Nutr. 1999; 81: 203-09.
Haidari F, Seyed-Sadjadi N, Taha-Jalali M, Mohammed-Shahi M. The effect of oral administration of Carum carvi on weight, serum glucose, and lipid profile in streptozotocin-induced diabetic rats. Saudi Med J. 2011; 32: 695-700.
Hsu YJ, Lee TH, Chang CL, Huang YT, Yang WC. Antihyperglycemic effects and mechanism of Bidens pilosa water extract. J Ethnopharmacol. 2009; 122: 379-83.
KhareIndian CP. Medicinal plants, An illustrated dictionary. Berlin/Heidelberg, Springer-Verlag, 2007, pp 508-83.
Kishore L, Kaur N, Kajal A, Singh R. Extraction, characterization and evaluation of Eruca sativa against streptozotocin-induced diabetic nephropathy in rat. Bangladesh J Pharmacol. 2017; 12: 216-27.
Krishnaveni S, Balasubramanian T, Sadasivam S. Sugar distribution in sweet stalk sorghum. Food Chem. 1984; 15: 229-32.
Maksimovic Z, Malencié D, Kovacevié N. Polyphenol contents and antioxidant activity of Maydis stigma extracts. Bioresour Technol. 2005; 96: 873-77.
Mathew PT, Augusti KT. Hypoglycaemic effects of onion, Allium cepa Linn. on diabetes mellitus: A preliminary report. Indian J Physiol Pharmacol. 1975; 19: 213-17.
Mburu F, Dumarcay S, Huber F, Petrissans M, Gérardin P. Evaluation of thermally modified Grevillea robusta heart-wood as an alternative to shortage of wood resource in Kenya: Characterisation of physicochemical properties and improvement of bio-resistance. Bioresour Technol. 2007; 98: 3478-86.
Miller GL. Use of dinitrosalicylic acid reagent for determination of reducing sugar. Anal Chem. 1959; 31: 426-28.
Mohammadi J, Naik PR. Evaluation of hypoglycemic effect of Morus alba in an animal model. Indian J Pharmacol. 2008; 40: 15-18.
Motilla P, Vargas JF, Munoz De, Agueda MC, Valdelvira ME, Cabrera ES. Oxidative stress in diabetic rats induced by streptozotocin: Preventive effects of melatonin. J Pineal Res. 1998; 25: 94-100.
Narendhirakannan R, Subramanian S, Kandaswamy M. Biochemical evaluation of antidiabetogenic properties of some commonly used Indian plants on streptozotocin-induced diabetes in experimental rats. Clin Exp Pharmacol Physiol. 2006; 33: 1150-57.
Ohkawa H, Ohishi H, Yagi K. Assay for lipid peroxide in animal tissues by thiobarbituric acid reaction. Anal. Biochem. 1979; 95: 351-58.
Palanichamy S, Nagarajan S, Devasagayam M. Effect of Cassia alata leaf extract on hyperglycemic rats. J Ethnopharmacol. 1988; 22: 81-90.
Pari L, Latha M. Effect of Cassia auriculata flowers on blood sugar levels, serum and tissue lipids in streptozotocin diabetic rats. Singapore Med J. 2002; 43: 617-21.
Pari L, Maheswari JU. Hypoglycaemic effect of Musa sapientum L. in alloxan-induced diabetic rats. J Ethnopharmacol. 1999; 68: 321-25.
Puntel RL, Roos DH, Grotto D, Garcia SC, Nogueira CW, Rocha JB. Antioxidant properties of Krebs cycle intermediates against malonate prooxidant activity in vitro: A comparative study using the colorimetric method and HPLC analysis to determine malondialdehyde in rat brain homogenates. Life Sci. 2007; 81: 51-62.
Russell KR, Omoruyi FO, Pascoe KO, Morrison EY. Hypoglycaemic activity of Bixa orellana extract in the dog. Methods Find Exp Clin Pharmacol. 2008; 30: 301-05.
Shinwari MI, Khan MA. Folk use of medicinal herbs of Margalla hills national park, Islamabad. J Ethnopharmacol. 2000; 69: 45-56.
Subramoniam A, Pushpangadan P, Rajasekharan S, Evans DA, Latha PG, Valsaraj R. Effects of Artemisia pallens Wall. on blood glucose levels in normal and alloxan-induced diabetic rats. J Ethnopharmacol. 1996; 50: 13-17.
Tsujita T, Takaku T, Suzuki T. Chestnut astringent skin extract, an alpha-amylase inhibitor, retards carbohydrate absorption in rats and humans. J Nutr Sci Vitaminol (Tokyo). 2008; 54: 82-88.
Verspohl EJ, Bauer K, Neddermann E. Antidiabetic effect of Cinnamomum cassia and Cinnamomum zeylanicum in vivo and in vitro. Phytother Res. 2005; 19: 203-06.
You Q, Chen F, Wang X, Luo PG, Jiang Y. Inhibitory effects of muscadine anthocyanins on alpha-glucosidase and pancreatic lipase activities. J Agric Food Chem. 2011; 59: 9506-11.
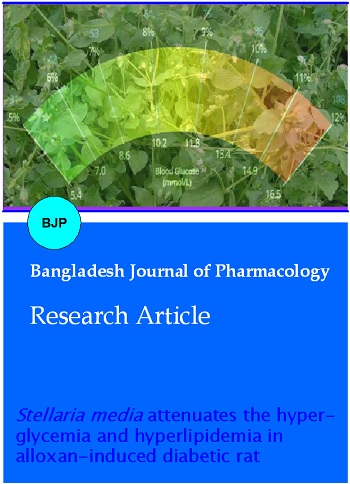