Increased DNA damage in blood cells of rat treated with lead as assessed by comet assay
Abstract
A growing body of evidence suggests that oxidative stress is the key player in the pathogenesis of lead-induced toxicity. The present study investigated lead-induced oxidative DNA damage, if any in rat blood cells by alkaline comet assay. Lead was administered intraperitoneally to rats at doses of 25, 50 and 100 mg/kg body weight for 5 days consecutively. Blood collected on day six from sacrificed lead-treated rats was used to assess the extent of DNA damage by comet assay which entailed measurement of comet length, olive tail moment, tail DNA (%) and tail length. The results showed that treatment with lead significantly increased DNA damage in a dose-dependent manner. Therefore, our data suggests that lead treatment is associated with oxidative stress-induced DNA damage in rat blood cells which could be used as an early biomarker of lead-toxicity.
Introduction
Lead is considered as a common occupational and environmental hazard throughout the world. Lead adversely affects the nervous, hematopoietic, endocrine, renal and reproductive systems of the body. Lead-induced oxidative stress in blood and other soft tissues has been postulated to be one of the possible mechanisms of lead-induced toxic effects (Pande and Flora, 2001). In recent studies, lead has been shown to inhibit the activities of antioxidant enzymes, including glutathione peroxidase, catalase and superoxide dismutase (Bolin et al., 2006; Ercal et al., 2001). Furthermore, generation of reactive oxygen species (ROS), stimulation of lipid peroxidation and depletion of antioxidant reserves have been postulated to be major contributors to lead-exposure related diseases (Patrick, 2006; Silbergeld et al., 2000). Recent evidence indicates that the lead treatment is coupled with enzyme inhibition, fidelity of DNA synthesis, mutation, chromosome aberrations, and cancer (Johnson, 1998).
In several human investigations, the increased levels of DNA damage were observed in the lead exposed workers using micronucleus assay or comet assay (Chen et al., 2006). In animal studies, lead could induce genotoxic damage in all organs of CD-1 mouse except the testicle (Valverde et al., 2002). Additionally, DNA damage was observed after treatment with lead in mouse blood cells (Devi et al., 2000) and in PC12 cells (Xu et al., 2006). Poma et al. (2003) found lead acetate-induced micronuclei and sister chromatid exchange with a dose-dependent manner in human melanoma cells. In fact, the genotoxic effects of lead have been studied in a variety of systems; however the results are not conclusive. So, the purpose of our present study is to investigate the effect of lead on DNA damage of the rat blood cells and explore the correlation between the dose of the lead and the extent DNA damage by using comet assay.
Materials and Methods
Chemicals
All chemicals used for the present study were of analytical grade and purchased from Sigma, USA and Loba Chemie, India.
Animals and lead administration
Male Sprague-Dawley rats (7-8 weeks) were obtained from ICDDR’B (International Center for Diarrheal Disease and Research, Bangladesh). Rats were housed under standard environmental conditions and allowed free access to food and water for 2 weeks prior to the treatment. Rats of five group each were treated with three different lead dose levels of 25, 50, 100 mg/kg body weight (BW). The dose of the lead and the experimental paradigm of the current study were determined on the basis of the previous studies (Xu et al., 2008). Lead acetate was dissolved in saline (0.9% NaCl) with slightly acidic condition (as required) and administered intraperitoneally to animals at the doses of 25, 50 and 100 mg/kg body weight per day for 5 days consecutively. The cumulative doses of lead given to rats were thus 125, 250 and 500 mg/kg BW. Saline was administered to the 5 animals of the control group in the same manner as in the treatment groups. Following five days of lead treatment, animals were decapitated and 1 mL of blood was collected in an eppendorf tube containing 100 µL of 10% EDTA. The blood samples were stored in a dark box at 4ºC until use.
Comet assay
The comet assay was carried out according to the protocol described by Tice et al. (2000) with slight modification. 10 µL of whole blood was suspended in 75 µL 0.7% low melting point agarose in Phosphate Buffer Saline (PBS) at 37ºC and was pipetted immediately into a glass microscopic slide having a layer of 1% agarose. The slides were covered with another layer of agarose. Then, the slides were immersed in lysis solution at 4ºC for 1 hour to remove cellular membranes, proteins and so forth. Slides were then placed in a single row in a horizontal electrophoresis tank containing alkaline buffer at 4ºC for 20 min for DNA unwinding. After 20 min, the current was switched on and electrophoresis was carried out at 25 V, 300 mA for 40 min at the same temperature. Following removal of slides from lysis solution, the slides were washed for three times 5 min each with neutralization buffer. Subsequently the slides were visualized by silver staining as described by Nadin et al. (2001). All steps beginning with the isolation of lymphocytes were conducted under yellow light to minimize the possibility of cellular DNA damage. Slides were analyzed microscopically by using software called Computer Assay Software Project (CASP, version 1.2.2). Threshold values of CASP parameters were adjusted to obtain the optimal values for our staining protocol. The selected parameters were: Head center threshold (HCT) = 0.999, Comet thresholds (CT) = 0.005, Head threshold (HT) = 0.05, Tail threshold (TT) = 0.05 and profile 1. The comet parameters analyzed by CASP were: Length of head and length of tail of comet (in pixels), % of DNA in the comet’s head and in the tail, the tail moment (arbitrary units) and olive tail moment (arbitrary units).
The Olive tail moment (OTM) is [percent of DNA in the tail] x [distance between the center of gravity of DNA in the tail and that of center of gravity of DNA in the head in x-direction].
Statistical analysis
One-way analysis of variance (ANOVA) was used for statistical evaluation in all experiments. Bonferroni posthoc test was performed for multiple group comparisons. Data are shown as mean ± SEM. The value of p<0.05 was considered statistically significant.
Results
The alkaline comet assay showed a significant increase in comet length in all three treatment groups as compared to controls (Figure 1). As shown in Figure 2A, treatment with lead at 25, 50 and 100 mg/kg doses increases the comet length by 1.8, 3 and 4 fold respectively.
Figure 1: Comet images of blood cells from lead-treated rat. Following consecutive 5 days treatment with 0 (A), 25 (B), 50 (C) and 100 (D) mg/kg lead, the extent of DNA damage was assessed by comet assay coupled with silver staining. Treatment with lead increases the DNA damage in a dose-dependent fashion
Olive tail moment is a commonly used marker of DNA damage in comet assay. OTM data shows significant DNA damage in lead treated rat blood cells as compared to the control group. Treatment with lead at 25, 50 and 100 mg/kg doses increases the OTM by 5.4, 11 and 22 fold respectively (Figure 2B).
Figure 2: Bar diagrams showing the effect of lead on comet assay. Quantitative analysis of comet length (A), olive tail moment (B), tail DNA (C) and tail length (D) were carried out in rat blood cells following treatment with 0, 25, 50 and 100 mg/kg doses of lead for 5 consecutive days. Data are shown as mean ± SEM for separate groups of rats (n = 5). ap<0.05; bp<0.01; cp<0.001; (Bonferroni test)
The extent of damage can be expressed by measuring percent of DNA present in the tail in comet assay. Dose-dependent increase by 10, 22 and 40% of tail DNA has been observed following treatment with 25, 50 and 100 mg/kg of lead respectively as compared to controls (Figure 2C). This data indicates that increasing concentration of lead is associated with the migration of damaged head DNA from head to the tail region during electrophoresis.
Comet tail length increased with the increase of lead doses in all three different rat groups. Longest tail was found in 100 mg/kg group. As shown in Figure 2D, the mean value of tail length increased 8.5, 22.5 and 35 times respectively in 25, 50 and 100 mg/kg lead treatment groups when compared with control group.
Discussion
This study was carried out to investigate the dose-dependent effect of lead exposure on DNA damage by comet assay. Our data suggests that administration of lead is associated with DNA damage in dose-dependent fashion. We used whole blood in our study. RBCs being enucleated cells, the comet images we obtained in this study represented only white blood cells, presumably lymphocytes though we did not isolate lymphocytes by traditional histopaque procedure. Therefore, in the present study, we examined effect of lead on DNA damage in white blood cells.
The comet assay is a simple, sensitive, and rapid method that can be used to estimate DNA damage at the individual cell level through strand breaks, open repair sites, cross-links, and alkali labile sites caused by oxidative stress (Tice et al., 2000). The basis of the comet assay involves embedding a suspension of single cells in low melting point agarose on a microscope slide, thus creating a mini DNA agarose gel. The cells are lysed and then the DNA is electrophoresed. After silver-staining, the cells are observed under microscope and compared with control. In this study we found that cells from control rats had tightly compressed DNA and maintained the circular form of the normal nucleus, with little or no evidence of comet formation (Figure 1A). In contrast, cells from rats treated with different doses of lead acetate displayed an altered appearance (Figure 1B-D). The basis of this altered appearance is, during alkaline unwinding (at pH 13.0) double stranded supercoiled DNA was unwound and denatured. As a result, double stranded complex DNA became single stranded and relaxed. So while electrophoresis was carried out, due to electric attraction, fragmentized DNA (negatively charged) migrated towards anode leaving the intact DNA in the head region. If nucleus contains more damaged DNA, large amount of these broken DNA (which are small in size compared to intact DNA molecule) will migrate in the tail region. Thus comet like shape was formed as evident in Figure 1. The intensity of the comet tail relative to the head reflects the number of DNA breaks. Cells containing greater levels of DNA strand breakage generate comets with more intense 'tails' (Figure 1C and D). Densitometric and geometric parameters of the comets as determined using image analysis software suggest that lead administration dose-dependently increased the comet tail, OTM and DNA percentage in the tail.
To the best of our knowledge this is the first study showing the extent of oxidative DNA damage following the administration of lead using animal model.
Conclusion
Our results suggest that lead treatment is associated with oxidative stress leading to oxidative DNA damage. Therefore, DNA damage in the peripheral blood could be a suitable early biomarker to monitor the environmental or occupational lead toxicity.
Ethical Issue
All animal experiments were carried out according to the law of Animal Experiments Guidelines approved by National Institute of Health (NIH).
Acknowledgement
We thank Prof. Laila N. Islam, for her support and encouragement in this research.
References
Aykin-Burns N, Laegeler A, Kellogg G, Ercal N. Oxidative effects of lead in young and adult Fisher 344 rats. Arch Environ Contam Toxicol. 2003; 44: 417-20.
Bolin CM, Basha R, Cox D, Zawia NH, Maloney B, Lahiri DK, Cardozo-Pelaez F. Exposure to lead and the developmental origin of oxidative damage in the aging brain. Faseb J. 2006; 20: 788-90.
Chen Z, Lou J, Chen S, Zheng W, Wu W, Jin L, Deng H, He J. Evaluating the genotoxic effects of workers exposed to lead using micronucleus assay, comet assay and TCR gene mutation test. Toxicology 2006; 223: 219-26.
Devi KD, Banu BS, Grover P, Jamil K. Genotoxic effect of lead nitrate on mice using SCGE (comet assay). Toxicology 2000; 145: 195-201.
Ercal N, Gurer-Orhan H, Aykin-Burns N. Toxic metals and oxidative stress part I: Mechanisms involved in metal-induced oxidative damage. Curr Top Med Chem. 2001; 1: 529-39.
Johnson FM. The genetic effects of environmental lead. Mutat Res. 1998; 410: 123-40.
Nadin SB, Vargas-Roig LM, Ciocca DR. A silver staining method for single-cell gel assay. J Histochem Cytochem. 2001; 49: 1183-86.
Pande M, Flora SJS. Lead induced oxidative damage and its response to combined administration of a-lipoic acid and succimers in rats. Toxicology 2002; 177: 187-96.
Patrick L. Lead toxicity part II: The role of free radical damage and the use of antioxidants in the pathology and treatment of lead toxicity. Altern Med Rev. 2006; 11: 114-27.
Poma A, Pittaluga E, Tucci A. Lead acetate genotoxicity on human melanoma cells in vitro. Melanoma Res. 2003; 13: 563-66.
Silbergeld EK, Waalkes M, Rice JM. Lead as a carcinogen: Experimental evidence and mechanisms of action. Am J Ind Med. 2000; 38: 316-23.
Tice RR, Agurell E, Anderson D, Burlinson B, Hartmann A, Kobayashi H, Miyamae Y, Rojas E, Ryu JC, Sasaki YF. Single cell gel/comet assay: Guidelines for in vitro and in vivo genetic toxicology testing. Environ Mol Mutagen. 2000; 35: 206-21.
Valverde M, Fortoul TI, Diaz-Barriga F, Mejia J, del Castillo ER. Genotoxicity induced in CD-1 mice by inhaled lead: Differential organ response. Mutagenesis 2002; 17: 55-61.
Xu J, Ji LD, Xu LH. Lead-induced apoptosis in PC12 cells, involvement of p53, Bcl-2 family and caspase3. Toxicol Lett. 2006; 166: 160-67.
Xu J, Ling-jun L, Chen Wu, Xiao-feng W, Wen-yu Fu, Li-hong X. Lead induces oxidative stress, DNA damage and alteration of p53, Bax and Bcl-2 expressions in mice. Food Chem Toxicol. 2008; 46: 1488-94.
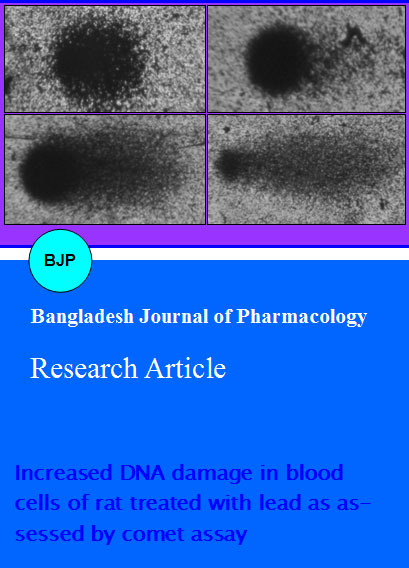