Antimicrobial activity of secondary metabolites produced by Aspergillus neobridgeri isolated from Pistacia lentiscus against multi-drug resistant bacteria
Abstract
The in vitro antibacterial activity of the endophytic fungus Aspergillus neobridgeri PLR9 (isolated from the plant Pistacia lentiscus) against a wide range of tested bacteria were studied. The maximum inhibitory activity was found on malt extract agar medium with a mean inhibitory zone of 11.3 mm (p<0.05). The ethyl acetate extract showed a significant inhibitory zone of 13.4 mm (p<0.05), followed by dichloromethane 9.1 mm. Whereas, n-hexane extract showed no activity. The largest inhibition zones were observed against Staphylococcus aureus 3, S. aureus 5 and Escherichia coli 1 with 21.0 mm of diameter. The MIC's were ranged between 312.5 and 1,250 μg/mL. The MBC's were ≤2500 μg/mL for most Gram positive bacteria and ≥2500 μg/mL for most Gram negative bacteria. Time kill assay study against the Enterococcus faecium showed a bactericidal effect at 4x MIC and bacteriostatic against Acinetobacter baumannii at different concentrations. Moreover, it is the first report, PLR9 isolated as endophyte and studied for their antimicrobial activity.
Introduction
Endophytic fungi are present in all plant species studied so far and they can be found in the internal tissues of roots, stems, leaves, flowers, fruits or seeds (Tanapichatsakul et al., 2018). They received habitat and nutrients from their hosts and protect their hosts chemically from browsers, insects, biotic and abiotic stresses and improve plant growth of their host (Ibrahim et al., 2018; Malhadas et al., 2017).
Endophytes produce bioactive compounds similar to those in the host plant (Yu et al., 2014). In addition, novel chemical skeletons of secondary metabolites have antimicrobial, antiparasitic, cytotoxic, anti-inflammatory, antitumor, antioxidant and neuroprotective activities (Malhadas et al., 2017).
Due to their ability to produce of a wide range biologically active compounds and their ability to inhibit several pathogens in the plant, animal and human; endophytes from medicinal plants are becoming more interesting to be explored and are considered a promising resource for new bioactive compounds (Atiphasaworn et al., 2017).
Several studies have shown the ability of different species of endophytic fungi to inhibit pathogenic microorganisms, for example; Aspergillus terreus and Aspergillus tubingensis (Yadav et al., 2016), Diaporthe terebinthifolii LGMF907 (de Medeiros et al., 2018), Phomopsis prunorum (Qu et al., 2020), P. griseofulvum TPL25 (Luo et al., 2015), Alternaria GFAV15 (Yadav et al., 2020), Alternariatenuissima OE7 (Chatterjee et al., 2019) and Trichoderma koningiopsis QA-3 (Shi et al., 2020).
Among 16 fungal strains isolated from the Erigeron canadensis, the ethyl acetate extract of strains NPR003 and NPR005 showed the strongest antifungal inhibitory effects (Bai et al., 2017). Bai et al. (2019) isolated six endophytic fungal strains from Rumexmadaio, strains L1 and R1 belonged to the Colletotrichum and Fusarium genera respectively had stronger inhibitory effects on Staphylococcus aureus, and the R1 isolate was the source of two known antimicrobial agents dibutylphthalate and beauvericin. Another known metabolite named resorcinol type of lipid (compound 1) was purified from extract of the endophytic fungus Chaetomium cupreum isolated from the ornamental plant Mussaenda luteola. This compound showed significant antibacterial against Mycobacterium with MIC of 6.3 μg/mL, and also exhibited a strong inhibition against both E. coli (ATCC 25922) as well as S. aureus (ATCC 25923) (Shylaja et al., 2018). The endophytic fungi Aspergillus terreus and Trichoderma virens associated with Sri Lankan plants have the capacity to produce the compounds butyrolactone I and 9-epiviridol respectively. Both compounds exhibited MIC values in the range 128-256 μg/mL against Gram positive Bacillus subtilits, S. aureus, methicillin resistant S. aureus (MRSA) and Escherichia coli (Ratnaweera et al., 2018).
The species of Pistacia lentiscus belong to the family Anacardiaceae composed of more than eleven species. Fruits and leaves parts of P. lentiscus tree are commonly used in traditional Algerian medicine for the treatment of eczema, paralysis, diarrhea throat infection, jaundice and asthma. The plant oil is used for the treatment of small wounds, burns, coughs and erythema, respiratory problems of allergic origin and ulcers.
The leaf extracts of P. lentiscus, and P. atlantica have good antifungal activity and low antibacterial activity (Benhammou et al., 2008).
Endophytic fungi of the plant P. lentiscus are not yet studied for their antimicrobial activity. For these reasons, this paper is focused on the screening of antimicrobial activity of bioactive secondary metabolites produced by endophytic fungus PLR9 isolated from P. lentiscus.
Materials and Methods
Sampling of P. lentiscus
The fresh leaf, stem and root of P. lentiscus were collected from the Mansoura mountain located in the east of Bordj Bou Arreridj (36.052538 N, 4.491563 E), Algeria in March 2014. The identification of the plant was carried out in the Botanic Laboratory, Farhat Abbas University. The plant samples were kept in a sealed plastic bag and returned to the laboratory on the same day and the isolation of the endophytic fungi commenced within 24 hours of the collection of plant samples (Zerroug et al., 2018).
Isolation and preservation of endophytic fungus PLR9
The fungus PLR9 was isolated as a symptomless endophyte from the P. lentiscus. The plant samples were washed with running tap water, allowed to dry at room temperature. Before surface sterilization, the plant material was cut into small pieces (approximately 3 cm). The sample fragments were successively surface sterilized by immersion into 70% ethanol (1 min) and 2% sodium hypochlorite (3 min), 70% ethanol for 30 sec and then finally rinsed with sterilized distilled water three times for 2 min. The roots were aseptically placed on the petri dishes containing solid culture medium potato dextrose agar supplemented with penicillin (30 mg/L) and gentamicin (30 mg/L) to suppress the bacterial growth and incubated at 28°C until the outgrowth of endophytic fungi was discerned. Emergent fungi were isolated and inoculated into the fresh potato dextrose agar antibiotic-free medium and incubated at 28°C for 7 days. This step was repeated several times until the pure endophytic fungal stain with uniform colony was obtained. The water washing from the surface-sterilized samples showed no microbial growth on the potato dextrose agar after 10 days of incubation under the same condition. The isolate was maintained by a periodic transfer onto the potato dextrose agar at 4°C for short-term preservation and in sterile distilled water containing 30% v/v of glycerol for long-term preservation (Campos et al., 2015).
Morphological characteristics
The fungus PLR9 was identified initially based on the colony morphology inoculated onto the malt extract agar and potato dextrose agar media and incubated at 28°C for 10 days, after that all characteristics macroscopic and microscopic were noted. The microscopic identification of fungus was carried out by the lactophenol staining technique (Arevalo et al., 2009).
DNA extraction, PCR amplification and sequencing
The PLR9 isolate was inoculated onto the malt extract agar and incubated at 28°C for 5 days. Genomic DNA was extracted after that using the ultraclean microbial DNA isolation kit (MoBio, USA) as described in the manufacturer’s protocol, and then the extracted DNA was stored at -20°C. Standard gene regions ITS, BenA and calmodulin were used for molecular identification. ITS region was amplified using primers V9G, 5ʹ-TTACGT-CCCTGCCCTTTGTA-3ʹ (forward) and LS266, 5ʹ-GCATTCCCAAACAACTCGACTC-3ʹ (reverse), while BenA region was amplified using the primers Bt2a-F 5ʹ-GGTAACCAAATCGGTGCTGCTTTC-3ʹ (forward) and Bt2b-R 5ʹ-ACCCTCAGTGTAGTGA-CCCTTGGC-3ʹ(reverse), for the calmodulin the primers CMD5-F 5ʹ-CCGAGTACAAGGARGCCTTC-3ʹ and CMD6-R 5ʹ-CCGATRGAGGTCATRACGTGG-3ʹ were used. Polymerase chain reactions (PCR) were performed using Veriti 96-well thermal cycler (Applied Biosystems, USA). The final reaction volume that used was 25 µL, which containing 1 µL of genomic DAN, 2.5 µL of 2.5 µM forward and reverse primers, 2,5 µL of Taq buffer + KCl-MgCl2 (Fermentas), 2.5 µL of 25 mM MgCl2 (Fermentas), 2 µL of 2.5 mM dNP mix, 0,25 of 5 U/µL Taq DNA polymerase (Fermentats), and 11.75 µL of sterile deionized water. The thermal cycler program for ITS was as follows: start step of 5 min at 95°C, followed by 35 cycles of 30 sec at 95°C, 30 sec at 55°C annealing temperature, and 1 min at 72°C, followed by a final extension of 7 min at 72°C. β-Tubulin and calmodulin, the thermocycling conditions for amplifications had an initial denaturing step of 94°C for 10 min, 36 cycles of 94°C for 5 sec, 58°C (β-tubulin) and 56°C (calmodulin) for 45°C, 72°C for 1 min, followed by a final elongation step at 72°C for 7 min (Visagie et al., 2013; Demırel, 2016).
The PCR products were separated by electrophoresis and then purified and sequenced. The ITS locus was sequenced using V9G, LS266, ITS1–5’ TCCGTAGGTG-AACCTGCGG-3’ and ITS4–5’TCCTCCGCTTATTGA-TATGC-3’, while β-tubulin and calmodulin were sequenced using the same primers as those for the PCR amplification. Sequencing reactions were performed in an automated DNA Sequencer CEQ 8000 Genetic Analyses System.
Phylogenetic analysis
The ITS, β-tubulin and calmodulin gene sequences were disposed into GeneBank Data Library with the acession numbers (MK600510), (MK680531) and (MK680532) respectively. Thereafter, consensus sequence data of the three loci were blasted against the NCBI GeneBank database. A sequence dataset was created for each locus using newly generated sequences and those loaded from GeneBank. For phylogenetic analysis, the multiple sequence alignment was carried out using MUSCLE software in the MEGA 7 package, followed by manual adjustments. Maximum likelihood analyses were applied to all individual and combined ITS, β-tubulin and calmodulin alignment using MEGA 7 with the Tamurai-Nei model. Support of the internal branches of the trees was assessed by the bootstrap method with 1000 replications. The phylogram is rooted in the Aspergillus flavus CBS 100927 (Visagie et al. 2013; Houbraken et al., 2014; Siqueira et al., 2017).
Optimization of optimal culture medium for the production of antimicrobial substances
To optimize the production of antibiotics, five culture media recommended were tested: malt extract agar, potato dextrose agar, Sabouraud dextrose agar, yeast extract sucrose agar and yeast extract malt extract agar. The antimicrobial activity test was carried out by the agar cylinder technique described above against E. coli ATCC 25922, P. aeruginosa ATCC 27853, Citrobacter freundii ATCC 8090, Salmonella typhimurium ATCC 13311, S. aureus ATCC 25923, Enterococcus faecalis ATCC 49452, methicillin-resistant S. aureus (MRSA) ATCC 43300, B. cereus ATCC 49452 and one yeast C. albicans ATCC 1024. After collecting the results, a comparison of the inhibition zone averages was made to determine the best production medium that was used in the next fermentation (Devaraju and Satish, 2011).
Fermentation and crude extracts preparation
The crude extracts of endophytic fungus were obtained using the modified method of Sharma et al. (2016). The mycelial agar plugs with 1 cm in diameter were excised from the periphery of 7-days-old PLR9 culture, and the two plugs were inoculated into 250 mL Erlenmeyer flasks containing 100 mL of malt extract broth medium selected in the previous step. The flasks were incubated at 28°C for 21 days with periodical shaking at 150 rpm. After the complete growth, the cultures were taken out and filtered through sterile cheesecloth to separate the mycelial mass from the fermented broth. The resulting filtrates were extracted three times by sequential solvent extraction procedure using three solvents with different polarity: n-hexane, dichloromethane and ethyl acetate. An equal volume of solvents was added to the filtrate, and the flask that contains the mixture was placed on the shaker for 2 hours to allow complete extraction. The filtrate and the solvents were taken in a separation funnel and then kept for one hour till the two immiscible layers formed. Then, the organic layer was recovered and evaporated in a rotavapor ((R-215, BÜCHI Labortechnik AG, Flawil, Switzerland). The fungal extracts were then dissolved in dimethyl sulfoxide, passed through 0.22 µm filtration membrane and kept at 4°C.
Choice of the best solvent for extraction
In order to determine the best solvent for extraction which was used in the following steps: the obtained extracts in the solvents (ethyl acetate, dichloromethane and n-hexane) were tested for their antimicrobial activities. The obtained results were then processed statistically. The antimicrobial activity was performed using wells diffusion method against 8 bacteria, E. coli ATCC 25922, P. aeruginosa ATCC 27853, C. freundii ATCC 8090, S. typhimurium ATCC 13311, S. aureus ATCC 25923, E. faecalis ATCC 49452, methicillin-resistant S. aureus ATCC 43300, B. cereus ATCC 49452 and one yeast C. albicans ATCC 1024. Each bacterial strain was grown in plate count agar at 37°C for 18-24 hours to obtain a freshly grown pure culture. Some colonies from each bacterium were suspended in 0.8% NaCl and were mixed for 15 sec to ensure homogeneity. The turbidity was adjusted to match of a 0.5 MacFarland standard to have optical density of 0.08-0.1 at 625 nm corresponding to 1 x 108 CFU/mL using a spectrophotometer (Jenway 7310, Cole-Parmer). The bacterial suspension was swabbed with a sterile cotton swab evenly in the plate in which 30 μL of sterilized fungal extract was loaded. Dimethyl sulfoxide was used as a negative control. Petri dishes were incubated at 37°C for 24 hours and the diameters of the clear inhibition zones surrounding the holes were measured with a ruler and recorded in mm (Abiala et al., 2016; Mefteh et al., 2018).
Large-scale fermentation and semipurification
After the selection of ethyl acetate as the best solvent for extraction, the preparation of the semipurified ethyl acetate extract was carried out in a similar manner as that was used in the above step. But before concentrating, the ethyl acetate fraction was washed with an equal volume of water to remove the remaining polar constituents and salts. The dried ethyl acetate extract was further partitioned between n-hexane and 90% methanol (1:1) to remove fatty acids and other non-polar constituents. The remaining 90% methanol phase was concentrated using a rotary evaporator and finally dissolved in dimethyl sulfoxide (Kumar and Kaushik, 2013).
Antibacterial activity of ethyl acetate extract against multi-drug resistance
Semi-purified ethyl acetate extract fraction was tested for its ability to inhibit the growth of human pathogenic bacteria using the agar well diffusion assay as described above. A wide range consisting of 25 bacteria were used. Dimethyl sulfoxide was used as a negative control, vancomycin and imipenem as a positive control for Gram positive and Gram negative bacteria respectively (Abiala et al., 2016).
Minimum inhibitory concentration (MIC)
The minimum inhibitory concentration of antimicrobial compound, which is identified as the lowest concentration of this compound at which there is no visible growth of bacteria of fungi. MIC values of PLR9 ethyl acetate extract were determined by broth microdilution technique in 96-well microliter plates with minor modification, following the recommendations of the Clinical and Laboratory Standards Institute (Rani et al., 2017). Muller Hinton broth was made and sterilized using an autoclave. 90 μL of sterile Muller Hinton broth was added to each well of the microliter plate followed by the addition of fungal extract in dimethyl sulfoxide (stock solution 5,000 μg/mL dissolved in 1/10th diluted dimethyl sulfoxide in sterile Muller Hinton broth) in the first row of the microliter plate. And then, serial dilutions were performed to get final concentrations ranging from 4.9 to 2,500 μg/mL using the micropipette (A1-A10). The test microorganisms were grown in Muller Hinton broth at 37°C for 18-24 hours and adjusted to 0.5 McFarland standard corresponding to the optical density (OD625 0.08–0.1). The adjusted cultures were then diluted 100 times in Muller Hinton broth and used as inoculum at a final concentration to approximately 105 colony forming units (CFU). Each plate had a negative control contained Muller Hinton broth with test microorganisms in column A11 and blank control to check the sterility consisted only of Muller Hinton broth in the column A12. 1/10th diluted DMSO was used as a negative control. The plates were incubated at 37°C for 24 hours. After incubation, 10 μL of 0.2% 2,3,5-triphenyl tetrazolium chloride solution was added to each well and then plates were incubated at 37°C for 1 hour. A visible change of color purple to pink indicated the growth of microorganisms.
Determination of minimum bactericidal concentration (MBC) and MIC index
The minimum bactericidal concentration was determined using the results of the MIC assay. The concentrations showing no visible growth of bacteria were identified, 10 µL of the sample from each well was taken and streaking on agar plates to judge the viability. All the plates were then incubated at 37°C for 24 hours, the lowest concentrations of the extract that did not produce any bacterial growth (prevention the growth of >99.9% microbial cells) on the solid medium were regarded as MBC values for this extract. To determine the efficacy and the effect bactericidal of bacteriostatic on the bacterial growth of ethyl acetate extract, MIC index (MBC/MIC) was calculated. If MBC/MIC is ≤ 4, then it is bactericidal and if MBC/MIC is >4, then it is bacteriostatic (Rakholiya et al., 2015).
Box 1: Agar plug diffusion assay
Principle
Antimicrobial activity was screened for its inhibitory zone by the agar plug diffusion method.
Requirements
Microorganism [endophytic fungus (PLR9); Escherichia coli (ATCC 25922), Pseudomonas aeruginosa (ATCC 27853), Staphylococcus aureus (ATCC 25923), Bacillus cereus (ATCC 49452) and yeast Candida albicans (ATCC 1024)]; Potato dextrose agar; Mueller-Hinton agar medium; Sabouraud agar medium;
Procedure
Step 1: Fungus was grown on the surface of potato dextrose agar in petri dishes at 28°C for 14 days, 6 mm diameter of actively growing fungal culture discs from potato dextrose agar plates were cut using a sterile cork borer and placed on the surface of the Mueller-Hinton agar medium seeded with test bacteria and Sabouraud agar medium seeded with yeast.
Step 2: These plates were sealed with parafilm and kept in a refrigerator at 4°C for 4 hours to complete diffusion of antimicrobial compounds.
Step 3: The petri dishes were incubated at 37°C four 24 hours for bacteria and 72 hours for yeast.
Step 4: After incubation time, antimicrobial activity was determined by the presence of inhibition zones around the endophytic fungus plug and was measured in mm.
References
Astuti et al., 2014
References (video)
Martà et al., 2018; Merah et al., 2018; Qaralleh, 2018; Semerci et al., 2020
Time kill curve studies
Time kill analysis is a method used to measure the change in the number of bacterial colonies in the culture medium upon the addition of the sample over time. The lethal effect of PLR9 extract was performed using time kill assay as described elsewhere (Rani et al., 2017; Perim et al., 2018) with some modifications. Bacterial density of Enterococcus faecium 1 and Acinetobacter baumannii cultures was adjusted to a 0.5 McFarland standard, after that suspensions were diluted 1/10 time in Muller Hinton broth medium. A volume of 200 µL of bacterial suspension was introduced into 15 mL sterile falcon tubes containing 1800 µL of Muller Hinton broth, with different concentrations of extract fixed at 1× MIC, 2× MIC, and 4× MIC. The final concentration of bacterial suspension yielded was approximately 5 x 105 CFU/mL. Falcon tubes containing 1% dimethyl sulfoxide, bacterial inoculum and Muller Hinton broth without PLR9 extract were used as a positive control. All samples were then incubated at 37°C in a rotary shaker at 150 rpm. A volume of 100 µL of samples was pipetted out from each tube at 0, 1, 2, 4, 6, 12 and 24 hours, diluted 4 serially and 25 µL of diluted samples were spreaded over freshly prepared Muller Hinton agar and incubated at 37°C for 24 hours. After the incubation period, the emergent bacterial colonies were counted and compared with those of control. The reduction percentage in the total viable count of CFU was counted using the following formula:
Initial count – Count at x interval
%Reduction = × 100
Initial count
The logarithm of the viable counts (CFU/mL) was plotted against time to determine the killing rate. Generally, the bactericidal effect is obtained with a lethality percentage of 90% for 6 hours, which is equivalent to 99.9% (≥3 log10) of lethality after 24 hours of incubation, but if the original inoculum is maintained or the reduction less than 99.9% (≥3 log10) of the total number of CFU/mL in the original inoculum, the effect is bacteriostatic.
Statistical analysis
Statistical analysis was done by SAS/STAT® 9.2 software. Results of the antibacterial activities analysis were done by the one-way ANOVA followed by the Student-Newman-Keuls MULTIP-rank test to compare the averages of inhibition zones. The results were expressed as means ± SD. The difference was considered statistically significant when p<0.05.
Results
Isolation and identification of the endophytic fungus
PLR9 colony exhibited a rapid growth on malt extract agar and potato dextrose agar. The colony diameters after 7 days of incubation were 36-38 and 38-40 mm respectively. Mycelium white and no production of spores on both culture media after 7 days of incubation, the colony reversed yellowish to light brownish on potato dextrose agar and brown on malt extract agar. Extensive sporulation with yellowish-brown color was observed after prolonged incubation (since the 8th day of incubation), Conidia were globose and smooth, in short chains and vesicles spathulate. After 7th day, the fungus begins to produce reddish-brownish pigment in both culture media and became brown at the age on malt extract agar. Exudates secretion was observed only on malt extract agar and no sclerotia detected on both culture media (Figure 1).
Figure 1: Morphological characteristics of PLR9 isolate: Colony surface on potato dextrose agar (A), malt extract agar (B) after 7 days, potato dextrose agar (C), malt extract agar (D) after 10 days of incubation at 28 °C. Conidiophore + exudates under zoom stereo binocular microscope (E). Conidia (F), Conidiophore, mycelium (G) under microscope, and reddish/brownish pigments production (H)
The sequenced genes (internal transcribed spacer rDNA: 605 pb), β-tubulin (BenA: 520 pb) and calmodulin (CaM: 600 pb) of PLR9 isolate were disposed in GenBank database under accession numbers MK600510, MK680531 and MK680532 respectively. The BLAST search using GenBank database of the internal transcribed spacer, BenA and calmodulin sequences showed that PLR9 isolate shared 99% similarities with A. neobridgeri NRRL 13078.
The aligned data sets of internal transcribed spacer, BenA and calmodulin sequences for 30 strains including, PLR9 isolate and type or reference strains of related species and an out-group, were used for phylogenetic analysis. Maximum likelihood analyses were applied to all individual and combined genes.
Single and combined genes analyses of sequences revealed similar topologies with minor differences in the support values of the internal nodes. The internal transcribed spacer marker was the least informative, being unable to discriminate between closely related species. Maximum likelihood of tree based on the concatenated genes was used for presenting phylogenies (Figure 2), the tree was rooted in A. flavus CBS 100927, bootstrap support from 1000 replicates is shown at the nodes. The phylogeny based on the single and combined gene sequences shows that PLR9 is closely related to the species A. neobridgeri NRRL 13078 supported by bootstrap values for likelihood (ML> 99%).
Figure 2: Phylogenetic tree generated by Maximum Likelihood analysis based on the combined alignment of ITS, BenA, and CaM sequences data. The tree is rooted with A. flavus CBS 100927. Maximum likelihood bootstrap values MLBS≥70 % are given at the nodes
Morphological identification allowed to identify PLR9 isolate at the genus level as belonging to the Aspergillus genus. The identification at the species level was then confirmed by the molecular identification. Based on the morphological and molecular identification, the PLR9 isolate was identified as A. neobridgeri belongs to the Circumdati section.
Preliminary antimicrobial screening
The fungus showed a wide spectrum, inhibiting both Gram positive and Gram negative bacteria. PLR9 isolate exhibited moderate activity against B. cereus ATCC 49452 with a zone of inhibition of 10 mm, however, against S. aureus ATCC 25923 and E. coli ATCC 25922. The activity was stronger with inhibition zones of 15 and 16 mm respectively. On the other hand, the endophytic isolate showed weak to no ability to inhibit the growth of P. aeruginosa ATCC 27853 and C. albicans ATCC 1024 tested in this study respectively (Table I).
Table I: Zone of inhibition of endophytic fungi PLR9 against test microorganisms
Test microorganism | Inhibition zones (mm) |
---|---|
E. coli | 16 |
P. aeruginosa | 8 |
B. cereus | 10 |
S. aureus | 15 |
C. albicans | 0 |
Choice of optimal culture medium and solvent for the production and extraction of antimicrobial substances
To improve and select the best culture medium that allows the best production of the molecule bioactives, five culture media were tested; malt extract agar, potato dextrose agar, Sabouraud dextrose agar, yeast extract sucrose agar and yeast extract malt extract agar. The antimicrobial activity test was conducted by the agar cylinder technique. The maximum of the average of inhibition zones (11.3 mm) was recorded in malt extract agar medium and it was significantly higher than the other used media (p<0.05). In the second position, the potato dextrose agar medium showed an average of inhibition zones of 5.3 mm followed by yeast extract sucrose agar medium with an average of 1.2 mm. While Sabouraud dextrose agar and yeast extract malt extract agar showed the lowest activity (Figure 3).
Figure 3: Effect of culture media (A) and solvent used (B) on antibacterial activity of PLR9 isolate, means with the same letter are not significantly different at (p<0.05); MEA (malt extract agar), PDA (potato dextrose agar ), YSEA (Yeast extract sucrose agar), YMEA (yeast extract malt extract agar), SDA (Sabouraud dextrose agar); superscript “a, b, c†means with the same letter are not significantly different at p<0.05
Based on the preliminary screening of medium selection, only one medium was selected for metabolites production. PLR9 was fermented in malt extract broth and the produced secondary metabolites were extracted using three solvents, ethyl acetate, dichloromethane and n-hexane. Crude extract from ethyl acetate has shown the highest mean of inhibition zone of 13.4 mm with a significant difference at p<0.05, followed by dichloromethane that had a moderate antibacterial activity of 9.11 mm (Figure 4). The yeast Candida albicans is still resistant to both extracts. Whereas, using n-hexane extract, no activity had been shown against all the tested microorganisms.
Figure 4: Antibacterial activity of extracts of PLR9 isolate, B. cereus (A), Methicillin-resistant Staphylococcus aureus 2 (B), E. coli (C) and C. freundii (D)
Antibacterial activity of ethyl acetate extract against multidrug-resistant bacteria
Ethyl acetate crude extract of PLR9 isolate was found to have significant antibacterial activities against Gram positive bacteria followed by moderate activity against Gram negative. The largest inhibition zones were observed against S. aureus 3, S. aureus 5 and E. coli 1 with 21 mm of diameter. In contrast, 9 mm was observed against K. pneumoniae 1 and K. pneumoniae 2 as the weakest zone. However, the strains P. aeruginosa 2 and P. aeruginosa 3 are the most resistant bacteria (Table II). The comparison of means of inhibition zones shows that the Gram positive have a greater sensitivity to the ethyl acetate extract (17.96 mm) with respect to that of Gram negative bacteria (11 mm).
Table II: Antibacterial evaluation of PLR9 ethyl acetate extract against test bacteria
Zone of Inhibition (mm) |
|||
---|---|---|---|
Test microorganisms | PLR9 extract | Positive control | Negative control |
Gram positive bacteria | Vancomycin 30 | DMSO | |
Bacillus cereus | 16.5 ± 2.1 | 19 | 0 |
Micrococcus luteus | 19.5 ± 0.7 | 27 | 0 |
Microbacterium yannicii | 16.5 ± 0.7 | 46 | 0 |
Staphylococcus aureus 1 | 18.5 ± 0.7 | 20 | 0 |
Staphylococcus aureus 2 | 19 ± 0 | 21 | 0 |
Staphylococcus aureus 3 | 21 ± 0 | 21 | 0 |
Staphylococcus aureus 4 | 19.5 ± 0.7 | 20 | 0 |
Staphylococcus aureus 5 | 21 ± 0 | 19 | 0 |
Enterococcus faecalis 1 | 17 ± 0 | 19 | 0 |
Enterococcus faecalis 2 | 14 ± 0 | 18 | 0 |
Enterococcus faecalis 3 | 12 ±0 | 20 | 0 |
Streptococcus pyogenes | 21 ± 0 | Non-detectable | 0 |
Gram negative bacteria | Imipenem 10 | DMSO | |
Citrobacter freundii | 16.5 ± 2.1 | 30 | 0 |
Acinetobacter baumannii | 12.5 ± 0.7 | 0 | 0 |
Pseudomonas aeruginosa 1 | 9.5 ± 0.7 | 24 | 0 |
Pseudomonas aeruginosa 2 | 0 ± 0 | 0 | 0 |
Pseudomonas aeruginosa 3 | 0 ± 0 | 0 | 0 |
Proteus mirabilis | 18 ± 0 | 0 | 0 |
Salmonella typhimurium | 0 ± 0 | 22 | 0 |
Escherichia coli 1 | 21 ± 1.4 | 30 | 0 |
Escherichia coli 2 | 16 ± 1.4 | 31 | 0 |
Escherichia coli 3 | 16 ± 0 | 29 | 0 |
Escherichia coli 4 | 15.5 ± 0.7 | 21 | 0 |
Klebsiella pneumoniae 1 | 9 ± 0 | 18 | 0 |
Klebsiella pneumoniae 2 | 9 ± 0 | 17 | 0 |
The MIC’s obtained in this study were ranged between 312.5 and 1250 μg/mL. The MBC’s were ≤ 2500 μg/mL for most Gram positive bacteria, but for most Gram negative bacteria were ≥2500 μg/mL. The MBC/MIC ratio was found always ≤4 for all Gram positive bacteria, which showed that the effect of ethyl acetate extract was bactericidal. On other hand, the extract had bacteriostatic effect (MBC/MIC ≥ 4) against most Gram negative bacteria, in particular K. pneumoniae 1, K. pneumoniae 2, P. aeruginosa 2 and P. aeruginosa 3 which confirms the resistance founded in the precedent part (Table III).
Table III: MIC and MBC of ethyl acetate fraction against bacteria
Pathogenic bacteria | Ethyl acetate extract of PLR9 isolate | ||
---|---|---|---|
MIC (µg/mL) |
MBC (µg/mL) |
MBC/MIC | |
Gram-positive bacteria | |||
Bacillus cereus | 312.5 | 1250 | 4 |
M. luteus | 625 | 625 | 1 |
Microbacterium yannicii | 156.3 | 312.5 | 2 |
Staphylococcus aureus 1 | 625 | 625 | 1 |
Staphylococcus aureus 2 | 625 | 2500 | 4 |
Staphylococcus aureus 3 | 625 | 1250 | 2 |
Staphylococcus aureus 4 | 625 | 625 | 1 |
Staphylococcus aureus 5 | 625 | 625 | 1 |
Enterococcus faecalis 1 | 625 | 625 | 1 |
Enterococcus faecalis 2 | 625 | 1250 | 2 |
Enterococcus faecalis 3 | 625 | 2500 | 4 |
Enterococcus faecalis 4 | 625 | 625 | 1 |
Enterococcus faecium 1 | 625 | 2500 | 4 |
Enterococcus faecium 2 | 625 | 2500 | 4 |
Streptococcus pyogenes | 312.5 | 312.5 | 1 |
Streptococcus australis | 625 | 625 | 1 |
Gram-negative bacteria | |||
Citrobacter freundii | 312.5 | 1250 | 4 |
Acinetobacter baumannii | 312.5 | 1250 | 4 |
Pseudomonas aeruginosa 1 | 625 | 1250 | 2 |
Pseudomonas aeruginosa 2 | 1250 | >2500 | >2 |
Pseudomonas aeruginosa 3 | 625 | >2500 | >4 |
Pseudomonas mirabilis | 312.5 | 2500 | 8 |
Salmonella typhimurium | 625 | >2500 | >4 |
Escherichia coli 1 | 312.5 | 1250 | 4 |
Escherichia coli 2 | 312.5 | 2500 | 8 |
Escherichia coli 3 | 312.5 | 2500 | 8 |
Escherichia coli 4 | 312.5 | 1250 | 4 |
Klebsiella pneumoniae 1 | 625 | >2500 | >4 |
Klebsiellapneumoniae 2 | 625 | >2500 | >4 |
Time kill curve studies
Time kill assay was performed over a period of 24 hours with the E. faecium 1 and A. baumannii being exposed to MIC, 2x MIC and 4x MIC values of ethyl acetate extract of PLR9 isolate. A graph was plotted between the logarithmic number of CFU/mL and time (Figure 5). The bactericidal effect is obtained with a percentage of 99.9% of lethality for 24 hours, but if the original inoculum is maintained or the reduction of less than 99.9% of the total number of CFU/mL in the original inoculum, the effect is bacteriostatic. For E. faecium 1, and after 24 hours of contact, time kill assay showed >99.9% of reduction at 4x MIC, but no significant reduction observed at MIC and 2x MIC concentrations. The time kill assay for A. baumannii, any reduction was observed at the MIC and 2x MIC concentrations, the maximum reduction noted was 23.7% at 4x MIC value. Therefore, the effect of fungal extract at 4x MIC concentration was bactericidal for E. faecium 1 and bacteriostatic against A. baumannii at all different concentrations, this confirms the resistance of Gram negative bacteria found during the first and second screening and MIC part.
Figure 5: Time kill curve of ethyl acetate crude extract of PLR9 against E. faecium 1 (A) and A. baumannii (B) at MIC, 2x MIC and 4x MIC
Discussion
Based on the morphological identification, the PLR9 isolate was identified as Aspergillus sp. Phylogenetic analysis of sequence parts of ribosomal RNA, BenA and CaM genes indicated that PLR9 is a member of Circumdati section and very close to the A. neobridgeri species supported by bootstrap values for likelihood (ML >99 %).
In all previous studies, A. neobridgeri has never been isolated from plants as endophyte. This is the first time, and in this study, where it was isolated from P. lentiscus. This species was first characterized in 2004 by Frisvad et al. (2004), it was isolated from soil in Nebraska, U.S.A., and has been described and validated as new species. However, various species of the Aspergillus genus have been isolated from different plants. Aspergillus flavipes Y-62 from Suaeda glauca (Akhter et al., 2018), Aspergillus tennesseensis from inner tissue of an unidentified marine alga (Li et al., 2018). Aspergilus flavus QQSG-3 isolated from Kandelia obobata (Wu et al., 2018), Aspergillus sp. from Trpterygium wilfordii (Qi et al., 2018). Aspergillus nidulans from Nyctanthes arbor-tristis (Sana et al., 2018). Cheng et al. (2018), they are found that the genus Aspergillus was among the most dominating endophytic fungi isolated from Paeonia lactiflora.
Endophytic fungi have been isolated from almost every plant species (over 400,000) examined to date (Mmbaga et al., 2018). In this study, we used all parts of P. lentiscus for isolation of endophytic fungi, but PLR9 isolate was isolated only from roots. A. neobridgeri PLR9 isolate may be of origin from the soil of the rhizospheric part and has some degree of specificity to both plant and the organ (James et al., 2012).
For the choice of the optimum culture medium, the malt extract agar was the best medium allowing the highest production of bioactive secondary metabolites. These results support the finding of Singh et al. (2012) and Kumari et al. (2018) who found malt extract agar as the best medium. On the other hand, Bhagat et al. (2016), (Thamilvanan et al. (2018), Deka and Jha, (2018) and Toghueo et al. (2018) were reported the potato dextrose agar as the best medium for the maximum growth and production of metabolites. Kalyani et al. (2016) found that the maximum growth and metabolite production by Aspergillus niger when Sabouraud dextrose agar is used. In the present study, ethyl acetate was found to be the best suitable solvent for maximum extraction of bioactive molecules. Many similar results were reported earlier which correlates with this work. Goutam et al. (2016) reported that among the three organic solvents used, the crude metabolites of Aspergillus terreus (JAS-2) extracted using ethyl acetate showed maximum cell inhibition against pathogenic bacteria. Similarly, results reported by Singh et al. (2015) regarding antibacterial activity of the endophytic fungus Alternaria sp., crude extract from ethyl acetate showed very good results compared to other solvents.
The antibacterial activity obtained by plug agar method for the PLR9 isolate may be due to the secretion of diffusible extracellular metabolites in the agar medium, these compounds having a specific effect against bacteria but no against yeast. This technique is easy and widely used to detect non-volatile compounds produced by microorganisms (Sadrati et al., 2013; Hamzah et al., 2018). It has been clearly reported that the antagonistic properties are largely influenced by the quality of the medium and the type of organisms. The biosynthesis of antibiotic substances was found to be largely influenced, both quantitatively and qualitatively by the composition of the medium (Selvamohan et al., 2016; Abdelghani, 2017). The maximum zone of inhibition that was recorded with malt extract agar medium indicate that their ingredients induced and which may serve as precursors for the biosynthesis of bioactive secondary metabolites by the isolate PLR9. The selection of the suitable solvent system highly depends on the specific nature of the bioactive compound targeted. Different solvents have ability to extract the bioactive compounds from culture broth, where; hexane and diethyl ether to isolate non-polar compounds, while ethyl acetate, dichloromethane, and chloroform were used to extract semi-polar compounds (Djamaan et al., 2018). Ethyl acetate was recognized as the best organic solvent which could be used to extract the fungal bioactive metabolites (Bhagat et al., 2016). These results indicate that the antibacterial bioactive compounds are semi-polar in nature and can be extracted using ethyl acetate and dichloromethane, but none of the antimicrobial compounds present in the non-polar fraction (n-hexane).
Ethyl acetate extract of A. neobridgeri PLR9 showed significant activity against Gram positive and Gram negative bacteria. No study has been done on the antibacterial activity of A. neobridgeri species. However, several works have been reported on the isolation and antibacterial activity of different species of endophytic fungi belonging to the genus Aspergillus. The extracts of these fungi were exhibited broad spectrum activity against Gram positive and Gram negative bacteria including multi-drug-resistant strains similarly with the results obtained in the present study. Bezerra et al. (2015) found that the endophytic Aspergillus ochraceus exhibited a high range of antibacterial activity, and inhibited the growth of four out of 10 pathogenic bacteria. Six different species of Aspergillus were isolated from Calotropis procera exhibited a good antibacterial activity against all tested bacteria but it was lower against Gram negative bacteria (Rani et al., 2017; Murali et al., 2017). Yadav et al. (2016) reported that extract of two endophytic fungi A. terreus and A. tubingensis are able to inhibit the growth of both K. pneumoniae and P. aeruginosa multi-drug resistant strains. Results of MIC values of the present study are in agreement with those obtained from the previous study of Aspergillus sp. extract, where MIC values ranged from 500 to 4000 µg/mL (Leong et al., 2018). The resistance phenomenon of Gram negative bacteria has generally been observed and which results in a bacteriostatic effect of the extracts during the time kill kinetics study, this has been shown by previous studies made on extracts of A. nomius (Rani et al., 2017) and those of A. terreus MP15 (Yin et al., 2015)
The reason for the different sensitivity between Gram positive and Gram negative bacteria could be ascribed to the morphological and chemical structure differences of their cell wall (Sadrati et al., 2013). Cell wall of Gram negative bacteria have an outer phospholipid membrane, lipopolysaccharide components and periplasmic space. This constitutes makes the cell wall less permeable to lipophilic solutes and provide an addition degree of protection against antibiotics targeting the cell wall (Yin et al., 2015). Whereas, the cell wall of Gram positive bacteria is less complex and can be more susceptible, this due to the presence of only outer peptidoglycan layer which is not an effective permeability barrier, and may facilitate the infiltration of hydrophobic compounds (Zainuddin et al., 2010). Aspergillus species are able to synthesize several molecules active against pathogenic bacteria (Ma et al., 2016 ; Gombodorj et al., 2017 ; Monggoot et al. 2018 ; Yang et al. 2018). A. neobridgeri and according to the results obtained by Frisvad et al. (2004), Visagie et al. (2014) and Varga et al. (2015) regarding the mycotoxins production using standards, this species has been found able to synthetizing penicillic acids, xanthomegnins, insulicolides, aspochracin and sclerotides. The significant antibacterial activity obtained in the present study may be due to the presence one or more these molecules in the crude extracts especially penicillic acid that which has been found active against bacteria (Ezzat et al., 2007; Varga et al., 2015; Nguyen et al., 2016), or due to other molecules that not yet characterized.
Conclusion
A. neobridgeri PLR9, an endophytic fungus isolated from the plant P. lentiscus was able to inhibit the growth of broad number of human pathogenic bacteria.
References
Abdelghani T. Production of antibacterial and antifungal metabolites by (S. albovinaceus) Strain no.10/2 and media optimization. Am Int J Biol. 2017; 5: 1-24.
Abiala M, Olayiwola J, Babatunde O, Aiyelaagbe O, Akinyemi S. Evaluation of therapeutic potentials of plant extracts against poultry bacteria threatening public health. BMC Complement Altern Med. 2016; 16: 417.
Akhter N, Pan C, Liu Y, Shi Y, Wu B. Isolation and structure determination of a new indene derivative from endophytic fungus Aspergillus flavipes Y-62. Nat Prod Res. 2018; 23: 1-6.
Arevalo J, Hidalgo-Diaz L, Martins I, Souza JF, Castro JMC, Carneiro RMDG, Tigano MS. Cultural and morphological characterization of Pochonia chlamydosporia and Lecanicillium psalliotae isolated from Meloidogyne mayaguensis eggs in Brazil. Trop Plant Pathol. 2009; 34: 158-63.
Astuti P, Sudarsono S, Nisak K, Nugroho GW. Endophytic fungi isolated from Coleus amboinicus Lour exhibited antimicrobial activity. Adv Pharm Bull. 2014; 4: 599-605.
Atiphasaworn P, Monggoot S, Gentekaki E, Brooks S, Pripdeevech P. Antibacterial and antioxidant constituents of extracts of endophytic fungi isolated from Ocimum basilicum var. thyrsiflora leaves. Curr Microbiol. 2017; 74: 1185-93.
Bai X, Yu R, Li M, Zhang H. Antimicrobial assay of endophytic fungi from Rumexmadaioand chemical study of strain R1. Bangladesh J Pharmacol. 2019; 14: 129-35.
Bai X, Zhou T, Lai T, Li Y, Chai J, Ni J, Zhang H. Isolation and antifungal screening of endophytic fungi from Erigeron canadensis. Bangladesh J Pharmacol. 2017; 12: 256-59.
Benhammou N, Bekkara FA, Panovska TK. Antioxidant and antimicrobial activities of the Pistacia lentiscus and Pistacia atlantica extracts. Afr J Pharm Pharmacol. 2008; 2: 22-28.
Bezerra JDP, Nascimento CCF, Barbosa RN, Silva DCV, Svedese VM, Silva-Nogueira EB, Gomes BS, Paiva LM, Souza-Motta CM. Endophytic fungi from medicinal plant Bauhinia forficata: Diversity and biotechnological potential. Braz J Microbiol. 2015; 46: 49-57.
Bhagat J, Kaur A, Kaur R, Yadav AK, Sharma V, Chadha BS. Cholinesterase inhibitor (Altenuene) from an endophytic fungus Alternaria alternata: Optimization, purification and characterization. J Appl Microbiol. 2016; 121: 1015-25.
Campos FF, Junior PAS, Romanha AJ, Araújo MSS, Siqueira EP, Resende JM, Alves TMA, Martins-Filho OA, Dos Santos VL, Rosa CA, Zani CL, Cota BB. Bioactive endophytic fungi isolated from Caesalpinia echinata Lam. (Brazilwood) and identification of beauvericin as a trypanocidal metabolite from fusarium sp. Mem Inst Oswaldo Cruz. 2015; 110: 65-74.
Chatterjee S, Ghosh R, Mandal NC. Inhibition of biofilm- and hyphal- development, two virulent features of Candida albicans by secondary metabolites of an endophytic fungus Alternaria tenuissima having broad spectrum antifungal potential. Microbiol Res. 2019; 2019.
Cheng K, Wei Z, Pu S, Xiang M, Yan A, Zhang Y, Wang X. Diversity of endophytic fungi of Paeonia lactiflora Pallas and screening for fungal paeoniflorin producers. FEMS Microbiol Lett. 2018; 365.
Deka D, Jha DK. Optimization of culture parameters for improved production of bioactive metabolite by endophytic Geosmithia pallida (KU693285) isolated from Brucea mollis Wall ex. Kurz, an endangered medicinal plant. J Pure Appl Microbiol. 2018; 12: 1205-13.
de Medeiros AG, Savi DC, Mitra P, Shaaban KA, Jha AK, Thorson JS, Rohr J, Glienke C. Bioprospecting of Diaporthe terebinthifolii LGMF907 for antimicrobial compounds. Folia Microbiol (Praha). 2018; 63:499-505.
Demirel R. Comparison of rDNA regions (ITS, LSU, and SSU) of some Aspergillus, Penicillium, and Talaromyces spp. Turk J Bot. 2016; 40: 576-83.
Devaraju R, Satish S. Endophytic mycoflora of Mirabilis jalapa L. and studies on antimicrobial activity of its endophytic Fusarium sp. Asian J Exp Biol Sci. 2011; 2: 75-79.
Djamaan A, Agustien A, Zam SI, Jannah M, Lalfari RS, Aldi Y, Dewi AP, Suci RP. Characterization of biopesticide compounds from Bacillus subtilis Aaf2 Uaac 20701 fermentation products. Int Res J Pharm. 2018; 9: 47-52.
Ezzat SM, El-Sayed EA, Abou EL-Hawa MI, Ismaiel AA. Morphological and ultrastructural studies for the biological action of penicillic acid on some bacterial species. Res J Microbiol. 2007, 2: 303-14.
Frisvad JC, Samson RA, Houbraken JAMP, Kuijpers AFA, Frank JM. New ochratoxin A or sclerotium producing species in Aspergillus section Nigri. Stud Mycol. 2004; 50: 45-61.
Gombodorj S, Yang MH, Shang ZC, Liu RH, Li TX, Yin GP, Kong LY. New phenalenone derivatives from Pinellia ternata tubers derived Aspergillus sp. Fitoterapia 2017; 120: 72-78.
Goutam J, Singh S, Kharwar RN, Ramarai V. In vitro potential of endophytic fungus Aspergillus terrus (JAS-2) associated with Achyranthus aspera and study on its culture conditions. Biol Med (Aligarh). 2016; 8: 349.
Hamzah TNT, Lee SY, Hidayat A, Terhem R, Faridah-Hanum I, Mohamed R. Diversity and characterization of endophytic fungi isolated from the tropical mangrove species, Rhizophora mucronata, and identification of potential antagonists against the soil-borne fungus, Fusarium solani. Front Microbiol. 2018, 9: 1707.
Houbraken J, Visagie CM, Meijer M, Frisvad JC, Busby PE, Pitt JI, Seifert KA, Louis-Seize G, Demirel R, Yilmaz N, Jacobs KB, Christensen M, Samson RA. A taxonomic and phylogenetic revision of Penicillium section Aspergilloides. Stud Mycol. 2014; 78: 373-451.
Ibrahim SRM, Mohamed GA, Al Haidari RA, Zayed MF, El-Kholy AA, Elkhayat ES, Ross SA. Fusarithioamide B, a new benzamide derivative from the endophytic fungus Fusarium chlamydosporium with potent cytotoxic and antimicrobial activities. Bioorg Med Chem. 2018; 26: 786-90.
James AW, Brian CS, Neil JM, Alan CG. Species and organ specificity of fungal endophytes in herbaceous grassland plants. J Ecol. 2012; 100: 1085-92.
Kalyani P, Geetha S, Hemalatha KPJ. Optimization of cultural conditions for improved production and bioactive metabolites by Aspergillus niger (Mttc-961). EJPMR. 2016; 3: 255-60.
Kumar S, Kaushik N. Endophytic fungi isolated from oil-seed crop Jatropha curcas produces oil and exhibit antifungal activity. PLoS One. 2013; 2013.
Kumari M, Taritla S, Sharma A, Jayabaskaran C. Antiproliferative and antioxidative bioactive compounds in extracts of marine-derived endophytic fungus Talaromyces purpureogenus. Front Microbiol. 2018; 9: 1777.
Leong CR, Arifah A, Mansur B, Rashid SA, Ang SN, Tan WN, Tong WY, Ibrahim D. Antimicrobial activity of Aspergillus sp. IBRL MP15 CCL, an endophytic fungus isolated from Swietenia macrophylla leaf against human pathogens. Malays J Microbiol. 2018; 14: 49-54.
Li ZX, Wang XF, Ren GW, Yuan XL, Deng N, Ji GX, Li W, Zhang P. Prenylated diphenyl ethers from the marine algal-derived endophytic fungus Aspergillus tennesseensis. Molecules 2018; 23: 2368.
Luo ZP, Lin HY, Ding WB, He HL, Li YZ. Phylogenetic diversity and antifungal activity of endophytic fungi associated with Tephrosia purpurea. Mycobiology 2015; 43: 435-43.
Ma YM, Ma CC, Li T, Wang J. A new furan derivative from an endophytic Aspergillus flavus of Cephalotaxus fortunei. Nat Prod Res. 2016; 30: 79-84.
Malhadas C, Malheiro R, Pereira JA, Guedes de Pinho P, Baptista P. Antimicrobial activity of endophytic fungi from olive tree leaves. World J Microbiol Biotechnol. 2017; 33: 46.
Martà M, FrÃgols B, Serrano-Aroca A. Antimicrobial characterization of advanced materials for bioengineering applications. JoVE. 2018: e57710.
Mefteh F Ben, Daoud A, Bouket AC, Thissera B, Kadri Y, Cherif-Silini H, Eshelli M, Alenezi FN, Vallat A, Oszako T, Kadri A, Ros-GarcÃa JM, Rateb ME, Gharsallah N, Belbahri L. Date palm trees root-derived endophytes as fungal cell factories for diverse bioactive metabolites. Int J Mol Sci. 2018; 19: 1986.
Merah S, Dahmane D, Krimat S, Metidji H, Nouasri A, Lamari L, Dob T. Chemical analysis of phenolic compounds and determination of antioxidant, antimicrobial and cytotoxic activities of organic extracts of Pinus coulteri. Bangladesh J Pharmacol. 2018; 13: 120-29.
Mmbaga MT, Gurung S, Maheshwari A. Screening of plant endophytes as biological control agents against root patho-gens of pepper (Capsicum annum L.). J Plant Pathol Microbiol. 2018; 9: 435.
Monggoot S, Pichaitam T, Tanapichatsakul C, Pripdeevech P. Antibacterial potential of secondary metabolites produced by Aspergillus sp., an endophyte of Mitrephora wangii. Arch Microbiol. 2018; 200: 951-59.
Murali M, Mahendra C, Hema P, Rajashekar N, Nataraju A, Sudarshana MS, Amruthesh KN. Molecular profiling and bioactive potential of an endophytic fungus Aspergillus sulphureus isolated from Sida acuta: A medicinal plant. Pharm Biol. 2017; 55: 1623-30.
Nguyen HT, Yu NH, Jeon SJ, Lee HW, Bae CH, Yeo JH, Lee HB, Kim IS, Park HW, Kim JC. Antibacterial activities of penicillic acid isolated from Aspergillus persii against various plant pathogenic bacteria. Lett Appl Microbiol. 2016; 62: 488-93.
Perim MC, Borges JDC, da Silva EML, Araújo TAS, da Silva ACO, da Silva VC, Carreiro SC, Cunha AF, Pranchevicius MCDS. In vitro antibacterial and time-kill assay of ethanolic extract of Davilla nitida bark on multidrug resistant bacteria isolated from diabetic foot lesions. Nat Prod Res. 2019; 33: 2383-88.
Qaralleh H. Chemical composition and antibacterial activity of Origanum ramonense essential oil on the β-lactamase and extended-spectrum β-lactamase urinary tract isolates. Bangladesh J Pharmacol. 2018; 13: 280-86.
Qi C, Gao W, Wang J, Liu M, Zhang J, Chen C, Hu Z, Xue Y, Li D, Zhang Q, Zhu H, Zhang Y. Terrusnolides A-D, new butenolides with anti-inflammatory activities from an endophytic Aspergillus from Tripterygium wilfordii. Fitoterapia. 2018; 130: 134-39.
Qu HR, Yang WW, Zhang XQ, Lu ZH, Deng ZS, Guo ZY, Cao F, Zou K, Proksch P.Antibacterial bisabolane sesquiterpenoids and isocoumarin derivatives from the endophytic fungus Phomopsis prunorum. Phytochem Lett. 2020; 37: 1-4.
Rakholiya KD, Kaneria MJ, Chanda SV. In vitro assessment of novel antimicrobial from methanol extracts of matured seed karnel and leaf of Mangifera indica L. (Kesar Mango) for inhibition of Pseudomonas spp. and their synergistic potential. Am J Drug Disc Dev. 2015, 5: 13-23.
Rani R, Sharma D, Chaturvedi M, Yadav JP. Antibacterial activity of twenty different endophytic fungi isolated from Calotropis procera and time kill assay. Clin Microbiol. 2017; 6: 280.
Ratnaweera PB, Walgama RC, Jayasundera KU, Herath SD, Abira S, Williams DE, Andersen RJ, de Silva ED. Antibacterial activities of endophytic fungi isolated from six Sri Lankan plants of the family Cyperaceae. Bangladesh J Pharmacol. 2018; 13: 264-72.
Sadrati N, Daoud H, Zerroug A, Dahamna S, Bouharati S. Screening of antimicrobial and antioxidant secondary metabolites from endophytic fungi isolated from wheat (Triticum durum). J Plant Prot Res. 2013; 53: 129-36.
Sana T, Siddiqui BS, Shahzad S, Farooq AD, Siddiqui F, Sattar S, Begum S. Antiproliferative activity and characterization of metabolites of Aspergillus nidulans: An endophytic fungus from Nyctanthes arbor-tristis Linn. against three human cancer cell lines. Med Chem. 2019; 15: 352-59.
Samson RA, Houbraken JAMP, Kuijpers AFA, Frank JM, Frisvad JC. New ochratoxin A or sclerotium producing species in Aspergillus section Nigri. Stud Mycol. 2004; 50: 45-61.
Selvamohan T, Parameswaran NK, Felcy SA. Isolation of Actinomycetes from different soils for analysing the antagonistic activity against pathogens. IJARBS. 2016; 3: 124-31.
Semerci AB, İnceçayır D, Mammadova V, Hoş A, Tunç K. Antimicrobial activities of Allium staticiforme and Allium subhirsutum. Bangladesh J Pharmacol. 2020; 15: 19-23.
Sharma D, Pramanik A, Agrawal PK. Evaluation of bioactive secondary metabolites from endophytic fungus Pestalotiopsis neglecta BAB-5510 isolated from leaves of Cupressus torulosa D. Don. 3 Biotech. 2016; 6: 210.
Shi XS, Li HL, Li XM, Wang DJ, Li X, Meng LH, Zhou XW, Wang BG. Highly oxygenated polyketides produced by Trichoderma koningiopsis QA-3, an endophytic fungus obtained from the fresh roots of the medicinal plant Artemisia argyi. Bioorg Chem. 2020; 94: 103448.
Shylaja G, Sasikumar K, Sathiavelu A. Antimycobacterial potential of resorcinol type lipid isolated from Chaetomium cupreum, an endophytic fungus from Mussaenda luteola. Bangladesh J Pharmacol. 2018; 13: 114-19.
Singh B, Thakur A, Kaur S, Chadha BS, Kaur A. Acetylcholinesterase inhibitory potential and insecticidal activity of an endophytic Alternaria sp. from Ricinus communis. Appl Biochem Biotechnol. 2012; 168: 991-1002.
Singh D, Rathod V, Singh AK, Joshi R, Nagaratna H, Avinash B. Antibacterial activity and phytochemical analysis of the crude extracts of endophytic fungus, Alternaria sp. from the medicinal plant Euphorbia hirta (L). Int J Green Chem Bioproc. 2015; 5: 14-20.
Siqueira JP, Sutton DA, Gené J, GarcÃa D, Wiederhold N, Peterson SW, Guarro J. Multilocus phylogeny and antifungal susceptibility of Aspergillus section Circumdati from clinical samples and description of A. pseudosclerotiorum sp. Nov. J Clin Microbiol. 2017; 55 : 947-58.
Tanapichatsakul C, Monggoot S, Gentekaki E, Pripdeevech P. Antibacterial and antioxidant metabolites of Diaporthe spp. isolated from flowers of Melodorum fruticosum. Curr Microbiol. 2018; 75: 476-83.
Thamilvanan D, Ram Kumar A, Ramesh R, Balakumar BS, Kumaresan S. In vitro antibacterial activity of the soil fungal metabolites. Int Res J Pharm. 2018; 9: 175-81.
Toghueo RMK, Sahal D, Zabalgogeazcoa Ã, Baker B, Boyom FF. Conditioned media and organic elicitors underpin the production of potent antiplasmodial metabolites by endophytic fungi from Cameroonian medicinal plants. Parasitol Res. 2018; 117: 2473-85.
Varga J, Baranyi N, Chandrasekaran M, Vágvölgyi C, Kocsubé S. Mycotoxin producers in the Aspergillus genus: An update. Acta Biol Szeged. 2015; 59: 151-67.
Visagie CM, Houbraken J, Rodriques C, Pereira CS, Dijksterhuis J, Seifert KA, Jacobs K, Samson RA. Five new Penicillium species in section Sclerotiora: A tribute to the Dutch Royal family. Mol Phylogeny Evol Fungi 2013; 31: 42-62.
Visagie CM, Varga J, Houbraken J, Meijer M, Kocsubé S, Yilmaz N, Fotedar R, Seifert KA, Frisvad JC, Samson RA. Ochratoxin production and taxonomy of the yellow aspergilli (Aspergillus section Circumdati). Stud Mycol. 2014; 78: 1-61.
Wu Y, Chen Y, Huang X, Pan Y, Liu Z, Yan T, Cao W, She Z. α-Glucosidase inhibitors: Diphenyl ethers and phenolic bisa-bolane sesquiterpenoids from the mangrove endophytic fungus Aspergillus flavus QQSG-3. Mar Drugs. 2018; 16: 307.
Yadav M, Yadav A, Kumar S, Yadav JP. Spatial and seasonal influences on culturable endophytic mycobiota associated with different tissues of Eugenia jambolana Lam. and their antibacterial activity against MDR strains. BMC Microbiol. 2016; 16: 44.
Yadav V, Singh A, Mathur N, Yadav R. Isolation and characterization of Alternaria GFAV15, an endophytic fungus from green fruit of Tinospora cordifolia (Willd.) Miers from semi-arid region. S AFR J BOT. 2020; 2020.
Yang SQ, Li XM, Xu GM, Li X, An CY, Wang BG. Antibacterial anthraquinone derivatives isolated from a mangrove-derived endophytic fungus Aspergillus nidulans by ethanol stress strategy. J Antibiot (Tokyo). 2018; 71: 778-84.
Yin OCJ, Ibrahim D, Lee CC. Bioactive compounds from Aspergillus terreus MP15, an endophytic fungus isolated from Swietenia macrophylla leaf. Mal J Med Biol Res. 2015; 2: 262-72.
Yu N, He L, Liu N, Wang Y, Xu H, Liu D. Antimicrobial action of an endophytic fungi from Sophor flavescens and structure identification of its active constituent. Biotechnol Biotechnol Equip. 2014; 28: 327-32.
Zainuddin N, Alias SA, Lee CW, Ebel R, Othman NA, Mukhtar MR, Awang K. Antimicrobial activities of marine fungi from Malaysia. Bot Mar. 2010; 53: 507-13.
Zerroug A, Sadrati N, Demirel R, Bakli S, Harzallah D. Antibacterial activity of endophytic fungus, Penicillium griseofulvum MPR1 isolated from medicinal plant, Mentha pulegium L. Afr J Microbiol Res. 2018; 12: 1056-66.
Zohary M. A monographical study of the genus Pistacia. Palestine J Bot Jerusalem Ser. 1952; 5: 187-228.
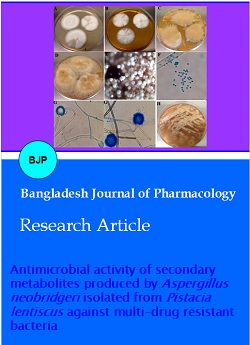
Apply citation style format of Bangladesh Journal of Pharmacology
Copyright (c) 2020 Nouari Sadrati, Amina Zerroug, Rasime Demirel, Sabrina Bakli, Daoud Harzallah

This work is licensed under a Creative Commons Attribution 4.0 International License.