Influence of ketamine on amino acid neurotransmitters secretion by nerve cells in vitro
Abstract
In order to study the influence of amino acid neurotransmitters secreted by the nerve cells after ketamine treatment, the nerve cells were cultured in vitro to exclude the interference of other factors in vivo and treated with three different doses of ketamine (1, 3 and 5 µg/mL). Then, the concentration of neuronal amino acid neurotransmitters was examined at 0, 15, 30, 45, 60, 90, 120 min after treatment. The trends of each amino acid concentration after ketamine treatment were nearly the same among the different treatment doses. After 15 min of adapting time, ketamine decreased the excitatory amino acid glutamic acid and aspartic acid concentration, and increased the concentration of the inhibitory amino acid glycine. Their concentrations showed a tendency to return approximately to the original level after 120 min.
Introduction
Ketamine, an n-methyl-D-aspartate antagonist, is commonly used for its sedative and analgesic properties in many species (Green et al., 1981). It could be administrated intramuscularly, intraperitoneally, or intravenously to provide relief of pain and distress (Song et al., 2012). In addition to its analgesic and anesthetic properties, ketamine possesses many advantages including easy administration, wide margin of safety, and its ability to be combined with other drugs (Gaertner et al., 2008). Thus, it is often used in combination with xylazine to anesthetize rodents. As an anesthetic, ketamine modulates neurotransmission at postsynaptic receptors such as n-methyl-D-aspartate (NMDA), glutamate receptors and gamma-aminobutryic acid (GABA) receptors. As a non-competitive antagonist, ketamine blocks NMDA receptor and induces a dissociative anesthesia (Bergman, 1999).
Ketamine is not only an anesthetic, but also a popular abusive drug. As an abusive drug, several studies showed clear toxic effects of ketamine in vitro (Mak et al., 2009; Wang et al., 2005; Lee et al., 2009). In recent years, it has also become a popular recreational drug in several countries of the world, due to its psychosis-like effects and cheap prices (Morgan et al., 2009). Ketamine abuse is mainly affecting the brain, thus inducing mental problems such as anxiety, confusion and memory loss.
In in vivo experimental studies, anesthesia procedures are of critical importance, especially when surgery or particularly invasive experimental approaches are performed on laboratory animals. However, it is important to evaluate the effects that anesthesia may have on the measured experimental parameters, since anesthetics possess powerful and poorly understood biological property. This caution is particularly relevant in studies analyzing brain function or neurochemistry. It is indeed known that anesthetic agents may modulate the neurotransmitter systems. Up to now, little is known about their effects on nerve cells in vitro.
Therefore, the aim of the present work was to analyze the secretion of neuronal amino acid neurotransmitter after adding ketamine to nerve cells, using an in vitro approach, to examine possible modifications in the amino acid secretion. In addition, we analyzed whether the modified secretion of neurotransmitters, when occurring, is due to a specific sensitivity of the nerve cells to this drug.
Materials and Methods
Animals
Sixteen-eighteen (16-18) days’ pregnant Wistar rats, weighing 250 ± 20 g, were provided by Harbin Hanfang mice breeding company of Heilongjiang Province.
Drugs and chemicals
Ketamine was provided by the College of Veterinary Medicine, North Agricultural University. Others were DMEM (Gibco/BRL, USA), FBS (Invitrogen, Cat. No. 16140071), glutamate assay kit (Sigma, Cat. No. MAK004), aspartate assay kit (Sigma, Cat. No. MAK095), glycine assay kit (Nanjing Jiancheng Bioengineering Institute).
Cell culture and ketamine treatment
The extraction of fetal rat brain was conducted as previously described (Beaudoin 3rd et al., 2012). Primary cultures of nerve cells were according to a previously described method (Tanaka et al., 2013). The pregnant rats were killed by cervical dislocation and the fetuses were removed from each rat. Subsequently, the head of the fetus was cut off and a small incision was made on the midline from the base of the skull to divide the skull into two halves to reveal the brain. The intact brain was gently removed from the skull and quickly placed in the dissection medium. During this sequence of events, it is important to be extremely fast and careful to avoid contamination. The brain tissue was immersed in the liquid separation and the cerebellum, the hippocampus, the cerebral cortex and other unnecessary tissues were removed to keep the cerebral cortex, which was used to make the nerve cells culture.
The cerebral cortex tissue underwent cutting, digesting, pipetting and filtering. After cell counting, cells were seeded into the culture plates and stored in a cell culture incubator at 5% CO2 and 37°C. The lipophilic 1-B-D arabin of uranosylcytosine (ara-C) 1-5 μm was added to the cell culture two days after inoculation, when and if it was necessary to inhibit the proliferation of non-neuronal cells. Half amount of the medium was replaced twice a week and the cell morphology was routinely observed.
After eight days of culture, ketamine was added to the medium containing the nerve cells at the concentration of 1, 3 and 5 μg/mL. The experiment was performed in triplicate per each concentration. Next, 40 μL supernatant was harvested in a EP tube at 0, 15, 30, 45, 60, 90, 120 min after treatment and the excitatory amino acid glutamic acid and aspartic acid and the inhibitory amino acid glycine and GABA were detected according to the kit manufactured instructions.
Statistical analysis
Data were analyzed using SPSS 17.0 statistical software and showed as the mean ± standard error. Statistical analyses were performed using one-way ANOVA and the Student’s t-test. p<0.01 was considered statistically significant.
Results
Figure 1 shows the concentration of glutamate in the nerve cells culture after ketamine administration. Ketamine at 1 µg/mL induced a 44% significant increase in glutamate concentration at 15 min after treatment (p<0.01, as compared with the 0 min group), and a decrease from 30 min till 120 min to a level close to the initial concentration, although remaining at a concentration slightly higher to be still significant respect time 0. Ketamine at 3 µg/mL induced a significant decrease in glutamate concentration at 60 min (p<0.01), with an increase at 90 and 120 min that was still significant respect time 0. Ketamine at 5 µg/mL induced a decrease in glutamate concentration, reaching the lowest level at 45 min (p<0.01, 33% decrease as compared with the 0 min group), and increasing from 60 till 120 min, although still significant respect time 0.
Figure 1: FIGURE_DESCRIPTION_HERE
Figure 2 shows the concentration of aspartate in the nerve cells culture after ketamine administration. Ketamine at 1, 3 and 5 µg/mL induced an increase of the aspartate concentration at 15 min, followed by a decrease, and finally an increase to reach the original concentration at 120 min. Ketamine at 1 µg/mL induced a 39% significant increase in aspartate concentration at 10 min (p<0.01 as compared with the 0 min group), while the increase induced by ketamine at 3 µg/mL was of the 59% at 15 min (p<0.01, as compared with the 0 min group), and the increase induced by ketamine at 5 µg/mL was of the 29% at 15 min (p<0.01, as compared with the 0 min group).
Figure 2: FIGURE_DESCRIPTION_HERE
Figure 3 shows the concentration of glycine in the nerve cells culture after ketamine administration. Ketamine at 1 µg/mL induced a 35% significant increase in glycine concentration at 45 min (p<0.01 as compared with the 0 min group), while ketamine at 3 µg/mL and 5 µg/mL induced a 23% and 18% significant decrease, respectively, at 15 min (p<0.01 as compared with the 0 min group). These decreased concentrations were back to the initial level at 120 min.
Figure 3: FIGURE_DESCRIPTION_HERE
In our experiment, for the excitatory amino acid, ketamine at 3 and 5 µg/mL, the concentration of glutamate both hold the trend of getting down first and up later. The lowest points happened at 60 min (3 µg/mL), 45 min (5 µg/mL). Ketamine at 1 µg/mL, 3 µg/mL, 5 µg/mL, the concentration of aspartate increased first, then decreased and finally increased to the normal level. Coincidentally, the top points also happened at the 15 min and the lowest points are all after 45 min. For the inhibitory amino acids, the glycine concentration, Ketamine at 1, 3 and 5 µg/mL decreased 15, 23 and 18% at 15 min separately compared with each 0 min group, at the same time, in these groups, 15 min are all the lowest points. Then they all began to get down first, then gradually increased, and finally returned to the normal level.
Discussion
In our experiments, after adding ketamine 15 min, the content of glutamate and aspartate were decreased significantly, and the content of glycine increased. A previous report showed that anesthesia induced by the dissociative anesthetic ketamine was quick and short, and inducing spontaneous alterations in movements, in particular during the induction of anesthesia. Ketamine reduced the levels of glutamate, aspartate and glycine, of approximately 27–72% according to the time of sampling and to the anesthetic used, compared to the values before anesthesia (Rozza et al., 2000).
Amino acid neurotransmitters represent the main signal neurotransmitters, and play a key role in the regulation of the spiritual awareness activities. Previous studies show that ketamine inhibits glutamate release from the cerebrocortical area of the brain (Buggy et al., 2000; Lindefors et al., 1997). Ketamine is considered an anesthetic (especially an inhalation anesthetic) that works by inhibiting the release of presynaptic glutamate, enhancing the re-uptake of glutamate and blocking the postsynaptic excitatory amino acid receptors (Boveris and Puntarulo, 1998). Several in vitro and in vivo studies demonstrated that volatile compounds depress the central nervous system through the inhibition of glutamate mediated transmission, both via post-synaptic depressant action and pre-synaptic inhibition (Martin et al. 1995; Dildly-Mayfield et al., 1996; Miyazaki et al., 1997). The level of circulating glutamate is an important factor influencing the synaptic efficacy. Moreover, the small changes of extracellular glutamate can alter the excitatory synaptic transmission by presynaptic and postsynaptic mechanisms (Zorumski et al., 1996). GABA is an important brain neurotransmitter amino acid, and plays an excitatory and inhibitory regulatory role in the activities of neurons. The anesthetics enhance the central inhibitory neurotransmission mediated by the GABAA receptor–channel complex (Tanelian et al., 1993). The anesthesia induced by ketamine was also considered to work by enhancing the GABA inhibitory neurotransmission in the central nervous system (Irifune et al., 2000). Previous studies showed that intravenous administration of anesthetics enhances GABAA receptors and calcium channels involved in the synaptic inhibition, both of which can be provoked by K+ induced reduction of glutamate release and increased GABA release, thereby inhibiting synaptic transmission (Kitayama et al., 2002). Moreover, it has been demonstrated, in an in vitro experiment, that halothane increases the possibility to open the glycine-activated channel, increasing the affinity of this receptor for the agonist. The increased glycine action may contribute to the general anesthesia of volatile agents (Wakamori et al., 1998).
The results of ketamine acted directly on cells in vitro fit well with the in vivo experiment, which means that ketamine had the same effect on in vivo and in vitro nerve cells. The secretion of excitatory amino acids decreased and inhibitory amino acid increased, they both reduced the effect of synaptic excitation, so that the nerve became desensitive to the excitation and made the body to anesthesia. Previously reports showed different results compared to our results, because they were in vivo experiments, where the neurotransmitter amino acids concentration was monitored directly in the part of the brain related to their site of release and the selected time points was less compared with our experiments. Thus, these previous reports are showing a simple trend of effects, and not a complex increase/decrease of the amino acids concentration, as we obtained in our results. However, our experiments performed in vitro using nerve cells from the cerebral cortex tissue and testing continuously the amino acids concentration, showed the influence and action of ketamine on the release of the neurotransmitter amino acids without the interference of in vivo factors due to the complexity of the whole brain.
Conclusion
The first 15 min may represent the time that the nerve cells needed to interact and to adapt to ketamine. The drug began to play its regulatory effect in the nerve cells only after 15 min after treatment, decreasing the concentration of the excitatory amino acid glutamate and aspartate and increasing the concentration of the inhibitory amino acid glycine, to achieve the anesthetic effect.
References
Beaudoin GM 3rd, Lee SH, Singh D, Yuan Y, Ng YG, Reichardt LF, Arikkath J. Culturing pyramidal neurons from the early postnatal mouse hippocampus and cortex. Nat Protoc. 2012; 7: 1741-54.
Bergman SA. Ketamine: Review of its pharmacology and its use in pediatric anesthesia. Anesth Prog. 1999; 46: 10-20.
Boveris AD, Puntarulo S. Free-radical scavenging actions of natural antioxidants. Nutr Res. 1998; 18: 1545-57.
Buggy DJ,Nicol B,Rowbotham DJ, et al., Effects of intravenous anesthetic agents on glutamate release: A role for GABAA receptor-mediated inhibition. Anesthesiology 2000; 92: 1067-73.
Dildly-Mayfield JE, Eger EI, Harris RA. Anesthetics produce subunit-selective actions on glutamate receptors. J Pharmacol Exp Ther. 1996; 276: 1058–65.
Gaertner DJ, Hallman TM, Hankenson FC, Batchelder MA. Anesthesia and analgesia for laboratory rodents. In: Anesthesia and analgesia in laboratory animals. Fish RE, Brown MJ, Danneman PJ, Karas AZ (eds). San Diego, Academic Press, 2008, pp 240–82.
Green CJ, Knight J, Precious S, Simpkin S. Ketamine alone and combined with diazepam orxylazine in laboratory animals: A 10-year experience. Lab Anim. 1981; 15: 163–70.
Irifune M, Sato T, Kamata Y, Nishikawa T, Dohi T, et al., Evidence for GABA(A) receptor agonistic properties of ketamine: Convulsive and anesthetic behavioral models in mice. Anesth Analg. 2000; 91: 230–36.
Kitayama M, Hirota K, Kudo M, Kudo T, Ishihara H, Matsuki A. Inhibitory effects of intravenous anaesthetic agents on K(+)-evoked glutamate release from rat cerebrocortical slices. Involvement of voltage-sensitive Ca2+ channels and GABA(A) receptors. Naunyn Schmiedebergs Arch Pharmacol. 2002; 366: 246-53.
Lee ST, Wu TT, Yu PY, Chen RM. Apoptotic insults to human HepG2 cells induced by S-(+)-ketamine occurs through activation of a Bax-mitochondria-caspase protease pathway. Br J Anaesth. 2009; 102: 80–89.
Lindefors N, Barati S, Connor WT. Differential effects of single and repeated ketamine administration on dopamine, serotonin and GABA transmission in rat medial prefrontal cortex. Brain Res. 1997; 759: 205-12.
Mak YT, Lam WP, Lü L, Wong YW, Yew DT. The toxic effect of ketamine on SH-SY5Y neuroblastoma cell line and human neuron. Microsc Res Tech. 2009; 73: 195–201.
Martin DC, Plagenhoef M, Abraham J, Dennison RL, Aronstam RS. Volatile anesthetics and glutamate activation of N-methyl-D-aspartatereceptors. Biochem Pharm. 1995; 49: 809–17.
Miyazaki H, Nakamura Y, Arai T, Katoaka K. Increase of glutamate uptake in astrocytes: A possible mechanism of action of volatile anesthetics. Anesthesiology 1997; 86: 1359–66.
Morgan CJ, Muetzelfeldt L, Curran HV. Ketamine use, cognition and psychological wellbeing: A comparison of frequent, infrequent and ex-users with polydrug and non-using controls. Addiction 2009; 104: 77–87.
Rozza A, Masoero E, Favalli L, Lanza E, Govoni S, Rizzo V, Montalbetti L. Influence of different anaesthetics on extracellular aminoacids in rat brain. J Neurosci Methods. 2000; 101: 165-69.
Song G, Wu H, Yoshino K, Zamboni WC. Factors affecting the pharmacokinetics and pharmacodynamics of liposomal drugs. J Liposome Res. 2012; 22: 177–92.
Tanaka T, Kai S, Matsuyama T, Adachi T, Fukuda K, Hirota K. General anesthetics inhibit LPS-induced IL-1β expression in glial cells. PLoS One 2013; 8: e82930.
Tanelian DL, Kosek P, Mody I, Maclver MB. The role of the GABAA receptor/chloride channel complex in anesthesia. Anesthesiology 1993; 78: 757–76.
Wakamori M, Ikemoto Y, Yamashita M. Halhotane increases the open probability of glycine-activated channel current in rat central neurones. Br J Anaesth. 1998; 80: 840–42.
Wang C, Sadovova N, Fu X, Schmued L, Scallet A, et al., The role of the n-methyl-D-aspartate receptor in ketamine-induced apoptosis in rat forebrain culture. Neuroscience 2005; 132: 967–77.
Zorumski C F, Mennerick S, Que J. Modulation of excitatory synaptic transmission by low concentrations of glutamate in cultured rat hippocampal neurons. J Physiol. 1996; 494: 465-77.
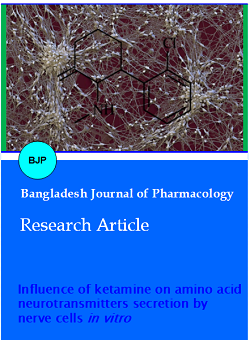
Apply citation style format of Bangladesh Journal of Pharmacology
Copyright (c) 2016 Mingxian Shi, Rui Chen, Cen Guo, Li Gao

This work is licensed under a Creative Commons Attribution 4.0 International License.