Bufalin inhibits the growth and epithelial to mesenchymal transition of human gastric cancer cells via modulation of MEK/ERK pathway
Abstract
This study was designed to evaluate the anti-cancer effects of bufalin against the human gastric cancer cells and unveil the underlying mechanism. The results showed that bufalin inhibited the proliferation and colony formation of the MGC-803 gastric cancer cells and exhibited an IC50 of 10 μM. These antiproliferative effects were found to be due to the induction of G2/M cell cycle arrest. The G2/M cell cycle arrest was also concomitant with inhibition of cdc2, cdc25 and cyclin B1. Furthermore, bufalin suppressed the epithelial-to-mesenchymal transition, migration, and invasion of the MGC-803 gastric cancer cells. The Western blot analysis revealed that bufalin exerted its effects via deactivation of EK/ERK signaling pathway. Taken together, these results suggest the potential of bufalin as the lead molecule for the development of chemotherapy for gastric cancer.
Introduction
Gastric cancer is a serious global disease and has been reported to be the 5th most diagnosed human cancer in 2018 (Thrift and El-Serag, 2020). The current mortality rate of gastric cancer is still around 75% which makes gastric cancer one of the major contributors to the global disease burden (Rawla and Barsouk, 2019).
Despite the application of advanced diagnostic regimes and effective therapeutic modalities, gastric cancer remains generally undetected till advanced stages and the overall 5-year survival rates are still not so encouraging.
During the past two decades, rigorous research explorations have led to the identification of many natural compounds with substantial biological effects and anti-cancer potential against several types of human cancer (Prakash et al., 2013). For instance, bufalin; a steroidal cardiotonic chemical molecule isolated from the Chinese toad venom prepared from white dried venom of Asiatic toad (Bufo gargarizans) has been reported to exert beneficial effects on the human body in terms of its pain-relieving activity, stimulation of blood pressure and myocardial contraction, anti-inflammatory and anti-cancer potential (Wang et al., 2018, Lan et al., 2019).
Bufalin has attained tremendous scientific attention during recent past years because of its anti-cancer effects against a wide range of cancer cell types like lung cancer, liver cancer, gastric cancer (Jiang et al., 2010; Qiu et al., 2013; Wamg et al., 2018). Gastric cancer cells are induced for apoptosis when treated with bufalin through several cell signaling pathways like PI3K/AKT and endoplasmic stress pathways (Li et al., 2019; Zhao et al., 2017). Bufalin was also shown to modulate mTOR and ERK-driven signaling cascades in gastric cancer cells (Qi et al., 2019).
However, the effects of bufalin have not been investigated on the cell cycle distribution, epithelial to mesenchymal transition, migration, invasion and MEK/ERK signaling pathway of gastric cancer cells.
Materials and Methods
Cell culture
GES-1 human gastric epithelial cells and MGC-803 gastric cancer cells were procured from the Pasture Institute of Cell Culture Collection (Iran). RPMI-1640 medium (Gibco BRL Life Technologies) supplemented with 10% fetal bovine serum (FBS, Sigma-Aldrich) and 1% penicillin/streptomycin (Gibco BRL Life Technologies) was used for in vitro propagation of both GES-1 and MGC-803 cells with 5% CO2/95% O2 environment in a CO2 incubator at 37ºC.
CCK-8 viability assay
The untreated GES-1 and MGC-803 cells and those treated with 2.5, 5, 10, 20, 40, 80 or 160 µM bufalin in a 96-well plate at 37ºC for 24 hours were mixed with diluted CCK-8 solution (Cell counting kit-8, Sigma-Aldrich). The 37ºC incubation was prolonged for 2 hours again after which the absorbance of each sample was read at 450 nm using the microplate reader (Thermo Fisher Scientific, USA).
Colony formation assay
For the analysis of relative colony formation, 5000 MGC-803 cancer cells were incubated without or with 5, 10 or 20 µM bufalin in 10 cm petri dishes at 37ºC in a humidified atmosphere of 5% CO2 for 14 days. The colonies formed were fixed with 70% ethanol and then stained with 0.1% crystal violet (Sigma-Aldrich). Dissecting microscope was used for manually counting the colonies.
Cell cycle analysis
MGC-803 cancer cells (2 x 105) were plated per well of a 12-well plate and incubated without or with 5, 10 or 20 µM bufalin for 24 hours at 37ºC. The cancer cells were harvested, PBS washed, fixed overnight with chilled 70% ethanol at 4ºC, reharvested and washed with PBS thrice. 0.5 mL of PBS containing 100 µg/mL RNase and 50 µg/mL PI was used for resuspending the cells followed by dark incubation for 1 hour at 37ºC. Finally, the flow cytometer was used for the analysis of the cancer cell cycle.
Box 1: Transwell Migration and Invasion Assays
Principle
The transwell cell migration assay measures the chemotactic capability of cells toward a chemoattractant whereas cell invasion assay measures both cell chemotaxis and invasion of cells through extracellular matrix, a process that is commonly present in cancer metastasis.
Requirements
Crystal violet (0.1%); MGC-803 cell; Optical microscope (Olympus Optical, Japan); Phosphate buffer solution (10%); Transwell; RPMI-1640
Procedure
Cell migration analysis
Step 1: MGC-803 cell suspension with cellular density of (100 µL; 2 × 105 cells/mL) were transferred to the upper chamber of a Transwell insert.
Step 2: Bufalin at concentrations (0, 5, 10 or 20 µM) were transferred to the upper chamber of a Transwell insert
Step 3: The lower chamber was filled with RPMI-1640 containing 10% phosphate buffer solution.
Step 4: The cells were incubated at 37ºC for 48 hours.
Step 5: After incubation, the membranes were collected and swabbed on the upper surface.
Step 6: The lower surface was stained with 0.1% crystal violet for 15 min at room temperature.
Step 7: Migrated cells on the lower surface of the membranes were visualized and counted under an optical microscope.
Cell invasion assay
Step 1: Cell invasion was also determined by using same procedure except for the upper chamber was matrigel coated overnight prior to invasion assay.
Reference
Zheng et al., 2020
Reference (video)
Zheng et al., 2017
Isolation of total RNA and semi-quantitative RT-PCR analysis
Total RNA was extracted using Trizol reagent (Invitrogen, USA) according to the manufacturer’s protocol. The extracted RNA was subjected to treatment with RNase-free DNaseI (TaKaRa, China) to eliminate genomic DNA contamination as per the manufacturer guidelines. The first strand of cDNA was synthesized from 2.0 μg of total RNA using the M-MLV First Strand Kit (Invitrogen) and the cDNA products equivalent to 200 ng of total RNA were used as templates in a 25 μL PCR reaction system. Semi-quantitative RT-PCR analyses for gene expression were performed on a PCR instrument (S1000™ Thermal Cycler, BIO-RAD, USA). PCR primers used in semi-quantitative RT-PCR were designed using Primer Premier 6.0 software to create PCR products actin gene was used as an internal control in semi-quantitative RT-PCR analysis
Agarose gel electrophoresis
Agarose gel electrophoresis was routinely carried out in 1x TAE both in gel as well as running buffer. For determining the expression, 0.8% gel was used while for the PCR products. Ethidium bromide was added to a final concentration of 0.5 μg/mL in the gel and the gel was run at 100 V until the dye reached 2/3rd of the gel. The gel was visualized under UV light and the image was captured using alpha imager- EC (Bio-Rad).
Western blotting
For Western blotting, the protein lysates were prepared from the untreated MGC-803 along with those treated with 5, 10 or 20 µM bufalin for 24 hours at 37ºC using RIPA lysis and extraction buffer (Thermo Fisher Scientific) which were subjected to SDS-PAGE and then transferred electrophoretically onto PVDF membranes. Primary and secondary antibody treatments were given to the PVDF membranes. Finally, the specific protein signals were detected using the ECL by ChemiScope 3000 Exp system. β-actin was used as an internal reference.
Immunofluorescence
MGC-803 cancer cells treated without or with 5, 10 or 20 µM bufalin for 24 hours at 37ºC were plated at a confluence of 50%-80% onto the sterilized glass coverslips. Paraformaldehyde (4%) was used for fixation of the coverslips for 15 min at room temperature while Triton x100 (0.1% solution in PBS) was used for cell permealization. The skimmed milk (5%; Cellconic, Korea) was used for blocking of coverslips for 1 hour at room temperature which were then exposed to primary antibodies for 1 hour. The coverslips were stained with DAPI and then mounted. The fluorescence was detected using Rhodamine or fluorescein isothiocyanate (FITC)-conjugated secondary antibodies. The cells were finally examined through confocal microscopy (Nikon, Japan).
Statistical analysis
The experimental data presented represent mean ± SD values determined by performing at least three replicates for each experiment. Graphpad prism 7.0 software was used for statistical analysis. The statistical difference between the two treatment groups was analyzed by Student’s t-test and a p-value <0.05 was considered to indicate the statistically significant difference.
Results
Growth of gastric cancer cells
Figure 1A presents the chemical structure of bufalin. Gastric cancer cells (MGC-803) were incubated for 24 hours at 37ºC without or with (2.5, 5, 10, 20, 40, 80 or 160 µM) bufalin in a 96-well plate and then the viability of cancer cells was estimated by CCK-8 proliferation assay. MGC-803 cancer cell viability was significantly lower under all treatment concentrations in comparison to that of the untreated cancer cells (Figure 1B). The decline in cancer cell growth followed treatment concentration dependence. The IC50 of bufalin against MGC-803 cancer cells was estimated to be 10 µM. On the other hand, when the same bufalin concentrations were employed against GES-1normal gastric epithelial cells, the cell growth was shown to be affected less prominently with an observed IC50 value of 84 µM (Figure 1C). The results indicated that selective growth inhibitory action of bufalin against the gastric cancer cells. MGC-803 untreated cancer cells and those treated with 5, 10 or 20 µM bufalin were analyzed for the colony formation through clonogenic assay (Figure 1D). There was a considerable decline in colony formation when the cancer cells were incubated with bufalin and also followed the dose-dependence. The relative number of colonies was shown to be reduced by 22, 50 and 80% under 5, 10 and 20 µM bufalin respectively (Figure 1E).
Figure 1: Bufalin exhibits selective antiproliferative activity against gastric cancer cells. (A) Chemical structure of bufalin; (B) CCK-8 viability analysis of MGC-803 gastric cancer cells incubated with different doses (in µM) of bufalin at 37ºC for 24 hours; (C) CCK-8 viability analysis of GES-1 gastric epithelial cells incubated with different doses (in µM) of bufalin at 37ºC for 24 hours (C) evaluation of colony formation from MGC-803 cancer cells incubated without or with 5, 10 or 20 µM bufalin; (D) relative percentage of colonies formed by MGC-803 cancer cells incubated without or with 5, 10 or 20 µM bufalin. The experiments were performed in three biological replicate and the results are shown as mean ± SD (*p<0.05)
G2/M phase cell arrest in gastric cancer cells
To find out the possible mechanism of growth inhibition of gastric cancer cells by bufalin, MGC-803 cells were treated with 5, 10 or 20 µM bufalin for 24 hours in 12-well plates. The cell cycle phase distribution of bufalin-treated cancer cells along with untreated MGC-803 cells was determined using a flow cytometer. The percentage of cancer cells was shown to be accumulating at G2 phase with increasing treatment concentration (Figure 2A). The percentage of the G2/M phase cells increased from 17.2% in control to 61.0% at 20 μM bufalin. The Western blotting of cell cycle regulatory proteins indicated that the expression of cdc2, Cyclin B1 and cdc25c proteins was highly repressed by bufalin in a dose-dependence manner (Figure 2B). The results are suggestive that bufalin induced G2/M cell cycle arrest in gastric cancer cells.
Figure 2: G2/M phase mitotic arrest is induced in bufalin treated gastric cancer cells. (A) Analysis of cell cycle phase distribution of MGC-803 cancer cells through flow cytometry incubated without or with 5, 10 or 20 µM bufalin at 37ºC for 24 hours; (B) relative expression analysis of cdc2, cyclin B1 and cdc25c cell cycle regulatory proteins form MGC-803 cancer cells through flow cytometry incubated without or with 5, 10 or 20 µM bufalin at 37ºC for 24 hours. Each experiment was performed with three replicates
Gastric cancer cell migration, invasion and EMT
Transwell assays were performed to understand the effect of bufalin on gastric cancer cell migration and invasion. Bufalin concentrations used were 5, 10 and 20 µM. The migration and invasion of bufalin administered MGC-803 cancer cells as well as the untreated cancer cells were analyzed after 48 hours of treatment. The cancer cells showed significantly lower migration and invasion when treated with bufalin and the higher treatment concentrations were shown to be more effective (Figure 3). The cell migration was decreased by 12, 41 and 71% while the invasion was reduced by 42, 81 and 89% respectively under 5, 10 and 20 µM bufalin treatment. In addition, the immunostaining of E-cadherin and vimentin marker proteins of EMT showed that bufalin treatment enhanced E-cadherin and repressed vimentin protein expression in MGC-803 cancer cells in a dose-dependent manner (Figure 4A). The Western blotting results of E-cadherin and vimentin marker proteins were also in agreement with their immunostaining (Figure 4B). The results indicate that bufalin inhibits the gastric cancer cell migration, invasion and EMT further suggesting its possible antimetastatic potential against gastric cancer.
Figure 3: Bufalin treated gastric cancer cells display significantly lower migration and invasion. Analysis of migration and invasion of MGC-803 cancer cells through transwell assays incubated without or with 5, 10 or 20 µM bufalin at 37ºC for 48 hours. The experiments were performed in three biological replicate and the results are shown as mean ± SD (*p< 0.05)
Figure 4: Bufalin inhibits the epithelial-to-mesenchymal transition of gastric cancer cells, in vitro. (A) Immunostaining of E-cadherin and vimentin marker proteins from MGC-803 cancer cells incubated without or with 5, 10 or 20 µM bufalin at 37ºC for 24 hours; (B) western blotting of E-cadherin and vimentin marker proteins from MGC-803 cancer cells incubated without or with 5µM, 10 µM or 20 µM bufalin at 37ºC for 24 hours. Each experiment was performed with three replicates
MEK/ERK signaling pathway in gastric cancer cells
To investigate the effect of bufalin in vitro treatment on MEK/ERK signaling pathway of gastric cancer cells, the Western blotting was used for analyzing the expression levels of p-ERK (phosphorylated), ERK, p-MEK (phosphorylated) and MEK signaling proteins from bufalin treated (5, 10 or 20 µM) and untreated MGC-803 cancer cells. The expression of both p-ERK and p-MEK proteins decreased with increased bufalin concentration while MEK protein expression increased (Figure 5). The expression of ERK remained by and large unaltered. The results suggest that bufalin induces blockage of MEK/ERK signaling pathway in gastric cancer cells.
Figure 5: Bufalin treatment induces blockage of MEK/ERK signaling pathway in gastric cancer cells. Relative protein expression analysis of p-ERK, ERK, p-MEK, MEK pathway proteins from MGC-803 cancer cells incubated without or with 5, 10 or 20 µM bufalin at 37ºC for 24 hours. Experiments were performed with three replicates
Discussion
The studies have suggested that bufalin has sizeable anti-cancer effects against human cancer cells in terms of its pro-apoptotic potential (Takai et al., 2014). Bufalin has been shown to display antiproliferative effects against gastric cancer cells through apoptosis induction (Zhao et al., 2015). In the present study, the gastric cancer cell growth was selectively inhibited by bufalin while the effects on proliferation of normal stomach cells were comparatively less prominent. This signifies the therapeutic applicability of bufalin to aid in chemotherapy treatment against gastric cancer. The administration of gastric cancer cells with bufalin in vitro resulted in G2/M phase cell cycle arrest which was evident from flow cytometry and expression analysis of cell cycle regulatory proteins cdc2, cyclin B1 and cdc25c. The latter proteins act as positive regulators of mitotic entry of eukaryotic cells (Liu et al., 2020). Bufalin induced cell cycle arrest in gastric cancer cells by down-regulating the expression of cdc2, Cyclin B1 and cdc25c proteins. Pancreatic cancer cells were also shown to be induced for G2/M cell cycle arrest by bufalin (Li et al., 2014).
The results of the current study also suggested antimetastatic potential of bufalin against gastric cancer cells. In agreement with its previous established activity, bufalin restrained the migration and invasion of gastric cancer cells in vitro (Wang et al., 2018). Cancer cell migration and invasion are key features of metastasis and are largely triggered through epithelial-to-mesenchymal transition (EMT) in cancer cells (Hua et al., 2020). EMT enhances the malignant behavior and aggressiveness of human cancer cells by enabling the cancer cells to invade the secondary sites through the process of metastasis (Ribatti et al., 2020). E-cadherin is an epithelial marker while vimentin is a mesenchymal molecular marker (Kallergi et al., 2011). The enhancement in E-cadherin and repression of vimentin protein in gastric cancer cells by bufalin indicated that bufalin restricts EMT of gastric cancer cells. Recently, it was shown that bufalin inhibited gastric cancer cell metastasis and EMT by modulating Wnt/ASCL2 expression (Wang et al., 2018). Bufalin was shown to block MEK/ERK signaling pathway in gastric cancer cells in the present study to exert its anti-cancer effects. The MEK/ERK pathway has a dominant regulatory role in controlling the cell cycle, differentiation and apoptosis (McCubrey et al., 2013). The signaling pathway is generally highly expressed in cancer cells and is considered a key therapeutic target in human cancer (Montagut and Settleman, 2009; Marampon et al., 2019).
Conclusion
Bufalin induces G2/M phase cell cycle arrest and selectively inhibits the growth of gastric cancer cells. Moreover, the migration, invasion and EMT of gastric cancer cells are significantly reduced by bufalin in vitro. The anti-cancer effects are exerted through MEK/ERK signaling pathway.
Acknowledgement
All the author of this manuscript is thankful to Shidong Hospital, Yangpu District, Shanghai, China, 200438 for providing the laboratory faciality for the conduction of experiments.
References
Dalton S. Cell cycle regulation of the human cdc2 gene. EMBO J. 1992; 11: 1797-804.
Hua W, Ten Dijke P, Kostidis S, Giera M, Hornsveld M. TGFβ-induced metabolic reprogramming during epithelial-to-mesenchymal transition in cancer. Cell Mol Life Sci. 2020; 77: 2103-23.
Jiang Y, Zhang Y, Luan J, Duan H, Zhang F, Yagasaki K, Zhang G. Effects of bufalin on the proliferation of human lung cancer cells and its molecular mechanisms of action. Cytotechnology 2010; 62: 573-83.
Kallergi G, Papadaki MA, Politaki E, Mavroudis D, Georgoulias V, Agelaki S. Epithelial to mesenchymal transition markers expressed in circulating tumour cells of early and metastatic breast cancer patients. Breast Cancer Res. 2011; 13: R59.
Lan YL, Lou JC, Jiang XW, Wang X, Xing JS, Li S, Zhang B. A research update on the anticancer effects of bufalin and its derivatives. Oncol Lett. 2019; 17: 3635-40.
Li D, Qu X, Hou K, Zhang Y, Dong Q, Teng Y, Zhang J, Liu Y. PI3K/Akt is involved in bufalin-induced apoptosis in gastric cancer cells. Anti-cancer Drugs. 2019; 20: 59-64.
Li M, Yu X, Guo H, Sun L, Wang A, Liu Q, Wang X, Li J. Bufalin exerts antitumor effects by inducing cell cycle arrest and triggering apoptosis in pancreatic cancer cells. Tumor Biol. 2014; 35: 2461-71.
Liu K, Zheng M, Lu R, Du J, Zhao Q, Li Z, Li Y, Zhang S. The role of CDC25C in cell cycle regulation and clinical cancer therapy: A systematic review. Cancer Cell Int. 2020; 20: 1-6.
Marampon F, Ciccarelli C, Zani BM. Biological rationale for targeting MEK/ERK pathways in anti-cancer therapy and to potentiate tumour responses to radiation. Int J Mol Sci. 2019; 20: 2530.
McCubrey JA, Steelman LS, Chappell WH, Abrams SL, Wong EW, Chang F, Lehmann B, Terrian DM, Milella M, Tafuri A, Stivala F. Roles of the Raf/MEK/ERK pathway in cell growth, malignant transformation and drug resistance. Biochim Biophys Acta Mol Cell Res. 2017; 1773: 1263-84.
Montagut C, Settleman J. Targeting the RAF–MEK–ERK pathway in cancer therapy. Cancer Lett. 2009; 283: 125-34.
Nie Y, Wu K, Yu J, Liang Q, Cai X, Shang Y, Zhou J, Pan K, Sun L, Fang J, Yuan Y. A global burden of gastric cancer: The major impact of China. Exp Rev Gastroenterol Hepatol. 2017; 11: 651-61.
Prakash OM, Kumar A, Kumar P. Anticancer potential of plants and natural products. Am J Pharmacol Sci. 2013; 1: 104-15.
Qi HY, Qu XJ, Liu J, Hou KZ, Fan YB, Che XF, Liu YP. Bufalin induces protective autophagy by Cblâ€b regulating mTOR and ERK signaling pathways in gastric cancer cells. Cell Biol Int. 2019; 43: 33-43.
Qiu DZ, Zhang ZJ, Wu WZ, Yang YK. Bufalin, a component in Chansu, inhibits proliferation and invasion of hepatocellular carcinoma cells. BMC Complement Altern Med. 2013; 13: 185.
Rawla P, Barsouk A. Epidemiology of gastric cancer: Global trends, risk factors and prevention. Prz Gastroenterol 2019; 14: 26.
Ribatti D, Tamma R, Annese T. Epithelial-Mesenchymal Transition in cancer: A historical overview. Transl Oncol. 2020; 13: 100773.
Takai N, Kira N, Ishii T, Yoshida T, Nishida M, Nishida Y, Nasu K, Narahara H. Bufalin, a traditional oriental medicine, induces apoptosis in human cancer cells. Asian Pac J Cancer Prev. 2012; 13: 399-402.
Thrift AP, El-Serag HB. Burden of gastric cancer. Clin Gastroenterol Hepatol. 2020; 18: 534-42.
Wang J, Cai H, Xia Y, Wang S, Xing L, Chen C, Zhang Y, Xu J, Yin P, Jiang Y, Zhao R. Bufalin inhibits gastric cancer invasion and metastasis by down-regulating Wnt/ASCL2 expression. Oncotarget 2018; 9: 23320.
Wang J, Cai H, Xia Y, Wang S, Xing L, Chen C, Zhang Y, Xu J, Yin P, Jiang Y, Zhao R. Bufalin inhibits gastric cancer invasion and metastasis by down-regulating Wnt/ASCL2 expression. Oncotarget 2018; 29: 23320.
Wang J, Xia Y, Zuo Q, Chen T. Molecular mechanisms underlying the antimetastatic activity of bufalin. Mol Clin Oncol. 2018; 8: 631-36.
Zhao H, Li Q, Pang J, Jin H, Li H, Yang X. Blocking autophagy enhances the pro-apoptotic effect of bufalin on human gastric cancer cells through endoplasmic reticulum stress. Biol Open. 2017; 6: 1416-22.
Zhao H, Zhao D, Tan G, Liu Y, Zhuang L, Liu T. Bufalin promotes apoptosis of gastric cancer by down-regulation of miR-298 targeting bax. Int J Clin Exp Med. 2015; 8: 3420.
Zheng L, He H, Shen X, Sun Y. Centipede Scolopendra suppesses cell growth in human epidermoid carcinoma cell A431. Bangladesh J Pharmacol. 2017; 12: 299-307.
Zheng Q, Jane JY, Li C, Li J, Wang J, Wang S. miR-224 targets BTRC and promotes cell migration and invasion in colorectal cancer. 3 Biotech. 2020; 10: 485.
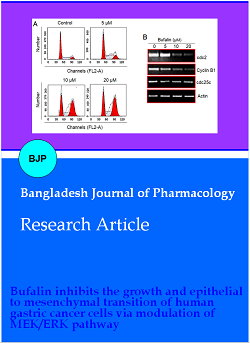