NF-κB signaling contributes to the inhibitory effects of Bombyx batryticatus on neuroinflammation caused by MPTP toxicity
Abstract
Bombyx batryticatus, the dried larvae of Bombyx mori infected by Beauveria bassiana, is a renowned traditional medicine. Previous report shows that B. batryticatus improved behavioral impairments, protected dopaminergic neurons, and maintained dopamine levels by inhibiting oxidative signaling in murine Parkinson’s disease model. In this study, the inhibitory effects of B. batryticatus on 1-methyl 4-phenyl 1,2,3,6-tetrahydropyridine (MPTP)-induced Parkinson’s disease in mice was investigated and explored the corresponding molecular mechanisms, while focusing on NF-κB signaling. Consequently, it was found that B. batryticatus inhibited glial and microglial activation and the levels of neuroinflammatory mediators, such as Cox-2, iNOS, and NF-kB, in the substantia nigra pars compacta. Moreover, pre-inhibition of NF-κB by BAY 11-7082, a κB kinase inhibitor, could neutralize the inhibitory effects of B. batryticatus against the activation of glia and microglia formerly induced by MPTP. It can be considered that B. batryticatus holds implications in providing anti-inflammatory neuroprotection by regulating NF-κB signaling.
Introduction
Parkinson’s disease is a progressive neurodegenerative disorder characterized by motor deficits including bradykinesia, resting tremor, rigidity, and postural instability (Seppi et al., 2019). Pathologically, Parkinson’s disease is characterized by the occurrence of dys-function and degenerative loss of dopaminergic neurons projecting from the substantia nigra pars compacta (SNpc) and the striatum, leading to a decreased content of dopamine in the basal ganglia (Sardi et al. 2018; Seppi et al., 2019). Although the mechanism of Parkinson’s disease is unknown, neuronal oxidation, neuro-inflammation, and consequent neuronal death have been implicated in its pathogenesis (Seppi et al., 2019).
Neuroinflammation is a physiological, biological, and host defense response, which is elicited to provide assistance in the repair of damaged tissue (Schain and Kreisl, 2017). It plays an important role in the development of innate immunity, confers protection to the organism, and initiates the healing process (Kohno et al., 2019; Schain and Kreisl, 2017). Microglia are found in the central nervous system (CNS) where they play a pivotal role in eliciting the immune response and in maintaining homeostasis in the brain, thereby protecting the CNS against diverse types of pathogens (Kohno et al., 2019; Subhramanyam et al., 2019). However, activated microglia can produce considerable amounts of harmful neurotoxic factors, such as nitric oxide (NO), prostaglandin E2 (PGE2), and pro-inflammatory cyto-kines, such as tumor necrosis factor-α (TNF-α), interleukin (IL)-1β, and IL-6, via activating nuclear factor (NF)-κB signaling (Kim et al., 2019; Singh et al., 2020). Thus, activated microglia contribute to the development of pathological processes, and reduction of the levels of inflammatory factors by inhibiting abnormal microglial activation may be an important therapeutic approach for the treatment and/or prevention of neurodegenerative diseases.
B. batryticatus, also known as Back-Gang-Jam in Korea, is the dried larva of Bombyx mori L. infected by Beauveria bassiana (Bals.) Vuill. B. batryticatus was originally described in the Chung-bu, a category of Dongui-Bogam, an ancient Korean medical book (Hu et al., 2017; Lim et al., 2019). According to a recent study, B. batryticatus is believed to possess anticonvulsant, antiepileptic, neurotrophic, anticoagulant, and antitumor properties (Park et al., 2019). B. batryticatus reportedly also exerts anti-apoptotic and antioxidant pharmacological effects (Hu et al., 2019; Lim et al., 2019). Recently, we have reported that B. batryticatus exerts neuroprotective effects on 1-methyl 4-phenyl 1,2,3,6-tetrahydropyridine (MPTP)-induced behavioral impairments and dopaminergic neuron damage via inhibition of oxidative stress by enhancing the response of oxidative defense systems including increasing heme oxygenase-1, NAD(P)H quinone oxidoreductase 1, and glutathione expression (Lim et al., 2019).
Although the neuroprotective effects of B. batryticatus have been demonstrated, its ability to inhibit neuroinflammation has not been examined. Therefore, in this study, we investigated the inhibitory effects of B. batryticatus on MPTP-induced neuroinflammation and explored the underlying mechanisms of action. We determined the inhibitory effects of B. batryticatus on lipopolysaccharide (LPS)- or MPTP-induced pro-inflammatory modulators. Additionally, we examined the potential mechanisms underlying the pharmacological effects of B. batryticatus by assessing the response of the NF-κB signaling pathway.
Materials and Methods
Chemicals
Sodium chloride, ethanol, dimethyl sulfoxide (DMSO), MPTP, phosphate-buffered saline (PBS), and sodium citrate buffer were purchased from Sigma–Aldrich (USA). Dulbecco’s modified Eagle medium (DMEM), penicillin/streptomycin, and fetal bovine serum (FBS) were purchased from Gibco (USA). Anti-rabbit and mouse-horseradish peroxidase (HRP) secondary, mouse anti-β-actin antibodies, and BAY 11-7082 were purchased from Santa Cruz Biotechnology (USA). Rabbit anti-iNOS and Cox-2 antibodies were purchased from Cell Signaling (USA). TransAM® assay kits for NF-κB p65 activation were purchased from Active Motif (USA). B. batryticatus extract was prepared according to previously published methods (Lim et al., 2019). All other reagents used were of guaranteed or analytical grade.
BV2 cell culture
The mouse microglial BV2 cell line was maintained in the DMEM supplemented with 10% heat-inactivated FBS and 1% penicillin/streptomycin, and incubated in an atmosphere of 95% air and 5% CO2 at 37°C. BV2 cells were seeded in 96-well plates at a density of 2 × 105 cells/mL and treated with B. batryticatus (0–1000 μg/mL) for 24 hours.
Nitric oxide (NO) assay
NO concentrations in culture supernatants were determined by measuring nitrite levels, which is a major stable product of NO, using the Griess reagent. Cells were pretreated with B. batryticatus for 1 hour, and were then treated with LPS (1 µg/mL) for additional 23 hours in 24-well plates. After collection of the culture supernatants, 50 µL of the culture medium was mixed with an equal volume of Griess reagent. Nitrite levels were determined using a microplate reader at 535 nm, with a standard curve generated after analysis of sodium nitrite solutions using the Chemi-Doc Band Analysis system (Bio-Rad Laboratories, USA).
Animals
Male C57BL/6 mice (age: 8 weeks; weight: 23–24 g) were purchased from Doo Yeol Biotech (Seoul, Korea) and maintained under temperature- and light-controlled conditions (20ºC–23ºC, 12-hours light/12-hours dark cycle) with food and water provided ad libitum. All animals were acclimatized for 7 days prior to drug administration.
Drug administration and brain tissue preparation
Mice were assigned to the following 10 different groups: 1) control; 2) MPTP; 3) MPTP + B. batryticatus 5 mg/kg/day; 4) MPTP + B. batryticatus 25 mg/kg/day; 5) control; 6) MPTP; 7) MPTP + B. batryticatus 5 mg/kg/day; 8) MPTP + B. batryticatus 25 mg/kg/day; 9) MPTP + B. batryticatus 25 mg/kg/day + BAY 11-7082 10 mg/kg/day; and 10) MPTP + B. batryticatus 25 mg/kg/day + BAY 11-7082 20 mg/kg/day.
B. batryticatus, dissolved in normal saline, was administered for 5 consecutive days. The control group received an equal volume of normal saline for the same duration. On day 3 of B. batryticatus treatment, MPTP (20 mg/kg; dissolved in saline) was injected intraperitoneally 4 times at 2-hours intervals. Equal volume (0.25 mL) of the vehicle was administered to the control group. On day 2 after MPTP treatment, the SNpc of mouse brain was removed, homogenized, and centrifuged using standard laboratory techniques to perform Western blotting and kit-based analyses. The final supernatant was stored at −80ºC until use.
Western blotting
The SNpc of each brain sample was lysed in the radioimmunoprecipitation assay lysis buffer containing a protease inhibitor cocktail. Protein concentration was determined by analysis of tissue extracts using the Bradford method. Remaining are mentioned in Box 1.
Box 1: Western blotting
Principle
Western blotting is used for detection and characterization of target proteins. It is based on the principle of immunochromatography where proteins are separated into polyacrylamide gel according to their molecular weight.
Requirements
Bovine serum albumin; ChemiDoc band analysis system; Chemiluminescence kit; Electrophoresis; HRP-conjugated secondary antibody; Protein extracts; Polyvinylidene fluoride membrane; Primary antibody; Sodium dodecyl sulfate polyacrylamide gel; Trans-Blot turbo transfer system
Step 1: Sample preparation
Samples were mixtures of 5xSDS-page loading buffer, lysis buffer and protein extracts, heated at 90°C for 10 min and briefly cooled on ice.
Step 2: Electrophoresis
Protein ladder and equal amounts of samples were loaded into each well of 4-20% sodium dodecyl sulfate-poly-acrylamide gel and were run at 100 V for 1 hour 30 min.
Step 3: Transfer
The proteins were transferred in an electrical field from the gel to a polyvinylidene fluoride membrane at 2.5 A 20 V 3 min using trans-blot turbo transfer system (Bio-Rad Laboratories).
Step 4: Blocking
Membranes were blocked with 3% bovine serum albumin in tris-buffered saline with tween-20 (TBST) for 1 hour at room temperature.
Step 5: Primary antibody and washing
Primary antibody was diluted to 3% bovine serum albumin and membranes were incubated in primary antibody solution at 4°C overnight. The membrane was then washed three times in TBST for 15 min each with gentle rocking.
Step 6: Secondary antibody and washing
Secondary antibody was diluted to 3% bovine serum albumin and membranes were incubated with an HRP-conjugated secondary antibody for 1 hour at room temperature. The membrane was again washed with TBST.
Step 7: Detection
Immunoreactivity was visualized using an enhanced chemilu-minescence kit and images were acquired using the ChemiDoc XRS+ system (Bio-Rad Laboratories). Relative expression levels were obtained based on the expression of housekeeping proteins (β-actin) using the ChemiDoc Band Analysis system (Bio-Rad Laboratories).
References
Park et al., 2017, Lim et al., 2019
Reference (video)
Pyo et al., 2019
Trans-AM DNA binding activity for NF-κB p65 activation
Efficiency of NF-κB p65 DNA-binding activity was evaluated using a commercially available TransAM® NF-κB p65 kit. Briefly, 23 μg of nuclear extract was incubated with immobilized wild-type or mutated competitor oligonucleotides. The bound NF-κB p65 was detected using an anti-NF-κB p65 primary antibody (1:1000 dilution) and a HRP-conjugated secondary antibody (1:1000 dilution) prior to chromogenic reaction with the tetramethylbenzidine substrate. Absorbance was measured at 450 nm using a plate reader.
Statistical analyses
All statistical parameters were calculated using the Graphpad Prism 5.0 software (Graphpad Software, USA). Values are expressed as means ± standard error of the mean (SEM). Statistical comparisons between the different treatments were performed using one-way analysis of variance (ANOVA) with Tukey’s multiple comparison post-test. A p-value <0.05 was considered to be statistically significant.
Results
Effects on LPS-induced NO production and IL-6 levels in BV2 cells
To investigate the inhibitory effects of B. batryticatus on LPS-induced NO production, NO and IL-6 levels in the cell supernatants were measured. The exposure to LPS led to significant increase in NO and IL-6 levels in BV2 cells (45.4 ± 0.1 μM and 4160.3 ± 18.7 pg/mL, respectively). Further, B. batryticatus pre-treatment (250 –1000 µg/mL) inhibited NO production and IL-6 level to 37.2 ± 0.4 – 28.1 ± 0.1 μM and 3332.8 ± 68.0 – 2721.9 ± 37.1 pg/mL, respectively (Figure 1).
Figure 1: Effect of B. batryticatus on liposaccharide-induced NO production and IL-6 expression level in microglial BV2 cells. (A) Determination of nitrite levels to estimate NO production. (B) Determination of IL-6 level. Values are presented as mean ± standard error of the mean. ap<0.001 vs. control group; bp<0.05, cp<0.01, and dp<0.001 vs. liposaccharide-alone group
Effects against MPTP-induced microglial and glial activation and levels of neuroinflammation mediators in mice
To investigate the inhibitory effects of B. batryticatus on activation of microglia and glia and neuroinflammation mediators, the expression levels of Iba-1, GFAP, Cox-2, and iNOS were measured. Exposure to MPTP led to significant increase in Iba-1 and GFAP levels in SNpc (290.9 ± 14.1% and 287.9 ± 27.0%, respectively) relative to the control. However, B. batryticatus treatment inhibited the expression levels of Iba-1 and GFAP to 190.9 ± 14.1 – 157.7 ± 3.6% and 228.7 ± 29.7 – 169.7 ± 17.1%, respectively (Figure 2). Moreover, exposure to MPTP led to significant Cox-2 and iNOS elevation in the SNpc (283.9 ± 13.7% and 224.3 ± 8.8%, respectively) relative to the control. B. batryticatus treatment inhibited the expression levels of Cox-2 and iNOS to 141.3 ± 12.3 – 90.9 ± 18.4% and 78.2 ± 3.8 – 86.2 ± 7.5%, respectively, relative to the control (Figure 3). Additionally, NF-κB p65 DNA-binding ability increased by B. batryticatus treatment in the nuclear fractions in SNpc (175.4 ± 7.3%) in contrast to the control group. B. batryticatus treatment inhibited NF-κB p65 activation to 129.7 ± 3.7 – 109.4 ± 6.2% (Figure 4).
Figure 2: Effect of B. batryticatus on MPTP-induced microglial and glial activation in SNpc of mice brain. (A) Western blot analysis of Iba-1 and GFAP (microglial and glial activation makers, respectively). β-actin is the internal control. Determination of (B) Iba-1 and (C) GFAP levels in the SNpc. Values are presented as mean ± standard error of the mean. ap<0.01 and bp<0.001 vs. control group; cp<0.05 and dp<0.001 vs. MPTP-treated group. MPTP, 1-methyl 4-phenyl 1,2,3,6-tetrahydropyridine; SNpc, substantia nigra pars compacta; Iba-1, ionized calcium-binding adapter molecule 1; GFAP, glial fibrillary acidic protein
Figure 3: Effect of B. batryticatus on MPTP-induced expression of Cox-2 and iNOS in SNpc of mice brain. (A) Western blot analysis of Cox-2 and iNOS. β-actin is the internal control. Determination of (B) Cox-2 and (C) iNOS levels in the SNpc. Values are presented as mean ± standard error of the mean. ap<0.001 vs. control group; bp<0.001 vs. MPTP-treated group. MPTP, 1-methyl 4-phenyl 1,2,3,6-tetrahydropyridine; SNpc, substantia nigra pars compacta; Cox-2, Cyclooxygenase-2; iNOS, inducible nitric oxide synthase
Figure 4: Effect of B. batryticatus on MPTP-induced NF-κB p65 activation in SNpc of mice brain. Values are presented as mean ± standard error of the mean. ap<0.001 vs. control group; bp<0.001 vs. MPTP-treated group. MPTP, 1-methyl 4-phenyl 1,2,3,6-tetrahydropyridine; SNpc, substantia nigra pars compacta; NF-κB, nuclear factor-κB
Figure 5: Effect of NF-κB inhibition on the inhibitory action of B. batryticatus in MPTP-intoxicated mouse model. (A) Western blot analysis of Iba-1 and GFAP (microglial and glial activation makers, respectively). β-actin is the internal control. Determination of (B) Iba-1 and (C) GFAP levels. Values are presented as mean ± standard error of the mean. ap<0.001 vs. control group; bp<0.05 and cp<0.001 vs. MPTP-treated group; dp<0.001 vs. MPTP and B. batryticatus (25 mg/kg)-treated group. NF-κB, nu-clear factor-κB; MPTP, 1-methyl 4-phenyl 1,2,3,6-tetrahydro-pyridine; BAY, BAY 11-7082; Iba-1, ionized calcium-binding adapter molecule 1; GFAP, glial fibrillary acidic protein
Effect of an NF-κB inhibitor against the effect of B. batryticatus on microglial and glial activation follow-ing MPTP treatment in mice
B. batryticatus pretreatment significantly inhibited NF-κB p65 associated with neuroinflammation in the SNpc after MPTP-intoxication. These results suggest that pre-inhibition of the NF-κB p65 pathway might neutralize the inhibitory effect of B. batryticatus against MPTP-induced glial activation. Therefore, we measured Iba-1 and GFAP levels in SNpc. Exposure to MPTP increased Iba-1 and GFAP levels (388.6 ± 5.6% and 213.1 ± 8.9%, respectively) as compared to the control group. As expected, the positive effect of B. batryticatus for microglial and glial activation by MPTP-intoxication (150.5 ± 6.8 – 140.8 ± 12.4% and 141.1 ± 9.0 – 97.1 ± 8.1%, respectively) was significantly neutralized by pre-inhibiting Nrf2 signaling using 10 – 20 mg/kg of the NF-κB inhibitor (244.4 ± 17.4 – 349.0 ± 6.2% and 155.7 ± 5.2 – 299.1 ± 24.2%, respectively; Figure 5). These results indicate that the neuroinflammatory effect of B. batryticatus does not appear after MPTP-intoxication and treatment with the NF-κB inhibitor. Notably, the effect of B. batryticatus is associated with the activation of NF-κB pathway.
Discussion
Generally, Parkinson’s disease is the result of increased susceptibility to neuroinflammation via oxidative stress (Block et al., 2007). In a previous study performed using an MPTP mouse model of Parkinson’s disease, we reported that B. batryticatus exhibited neuroprotective effects by inhibiting oxidative signaling through an enhancement of expression of antioxidant defense systems such as heme oxygenase-1, NAD(P)H quinone oxidoreductase 1, superoxide dismutase, catalase, and glutathione (Lim et al., 2019). Under pathological conditions, activated microglia can exert detrimental effects involving the overproduction of neurotoxic factors, such as NO, PGE2, and inflammatory cytokines (Block et al., 2007). Therefore, inhibition of the aberrant activation of microglia may demonstrate therapeutic potential in the treatment of neuroinflammation-related neurodegenerative diseases.
In the present study, we investigated the mechanisms underlying the anti-inflammatory properties of B. batryticatus in an LPS-stimulated inflammation model using BV2 microglial cells and in a Parkinson’s disease mouse model of MPTP-induced neuroinflammation. In response to LPS, BV2 microglial cells were activated and production of the inflammatory mediators, NO and IL-6, was promoted. These characteristic neuroinflammation changes can induce neurological dysfunction and lead to the development of neurodegenerative diseases such as PD (Amor et al., 2010).
Several studies have reported that the expression of Coxâ€2 and iNOS is induced in various CNS diseases. NO, derived primarily from iNOS, is a major neuroinflammatory modulator, and excessive NO production occurs in both acute and chronic neuroinflammation (Amor et al., 2010; Stephenson et al. , 2018). High levels of NO induce Coxâ€2 expression. Subsequently, the wellâ€known neuroinflammatory modulator, PGE2, which is produced by the action of Coxâ€2 from arachidonic acid, contributes to the development of many chronic neuroinflammatory diseases (Fakhoury, 2015; Stephenson et al., 2018). Therefore, blocking the production of these modulators has been an aim for the application of therapeutic antiâ€inflammatory drugs. Our findings demonstrated that B. batryticatus significantly decreased the levels of NO and IL-6, as well as inhibited iNOS and Cox-2 expression. These effects support the application of B. batryticatus as an antiâ€inflammatory agent.
NF-κB plays an important role in the regulation of microglia-mediated neuroinflammation (Singh et al., 2020; Subhramanyam et al. 2019). Dysregulation of NF-κB has been linked to aberrant neuroinflammation by the upregulated expression of proinflammatory media-tors (Lee and Suk, 2017). Indeed, specific NF-κB-binding regions have been identified in proinflammatory genes such as those for iNOS, Cox-2, and TNF-α (Baby et al., 2014; Lee and Suk, 2017). Therefore, inhibition of NF-κB transcriptional activity may prevent the progression of neurodegenerative diseases caused by neuroinflammation (Singh et al., 2020). As such, NF-κB has garnered interest as a molecular target for the development of therapeutic anti-inflammatory agents (Singh et al., 2020).
The results of the current study showed that treatment of mice with MPTP activated NF-κB expression, whereas B. batryticatus inhibited MPTP-stimulated NF-κB activity in the SNpc of mouse brain. Moreover, MPTP stimulation resulted in glial cell activation using GFAP and Iba-1 as markers, whereas B. batryticatus inhibited this activation. Inhibition of NF-κB by the κB kinase inhibitor, BAY 11-7082, neutralized the effects of B. batryticatus against the activation of glia induced by MPTP (Kim et al., 2010; Kumar et al., 2012). This suggests that B. batryticatus treatment inhibits neuroinflammation by affecting the NF-κB signaling activity.
Conclusion
The inactivation of NF-κB, at least partly, underlies the anti-inflammatory effect of B. batryticatus in LPS- or MPTP-stimulated inflammation via exertion of effects on glia and microglia. B. batryticatus elicits an anti-neuroinflammatory response via the NF-κB signaling pathway.
Ethical Issue
The experimental protocol was approved by the institutional animal care committee of the Korea Institute of Oriental Medicine (19-053, 20-003, and 20-078) and analysis was performed according to the guidelines of the Animal Care and Use Committee.
References
Amor S, Puentes F, Baker D, Van Der Valk P. Inflammation in neurodegenerative diseases. Immunology 2010; 129: 154-69.
Baby N, Patnala R, Ling E-A, Dheen S. Nanomedicine and its application in treatment of microglia-mediated neuroinflammation. Curr Med Chem. 2014; 21: 4215-26.
Block ML, Zecca L, Hong J-S. Microglia-mediated neurotoxicity: Uncovering the molecular mechanisms. Nat Rev Neurosci. 2017; 8: 57-69.
Fakhoury M. Role of immunity and inflammation in the pathophysiology of neurodegenerative diseases. Neurodegener Dis. 2015; 15: 63-69.
Hu M, Liu Y, He L, Yuan X, Peng W, Wu C. Antiepileptic effects of protein-rich extract from Bombyx batryticatus on mice and its protective effects against H2O2-induced oxidative damage in PC12 cells via regulating PI3K/Akt signaling pathways. Oxidative medicine and cellular longevity. 2019, 2019.
Hu M, Yu Z, Wang J. Traditional uses, origins, chemistry and pharmacology of Bombyx batryticatus: A review. Molecules 2017; 22: 1779.
Kim ME, Park PR, Na JY, Jung I, Cho JH, Lee JS. Anti-neuroinflammatory effects of galangin in LPS-stimulated BV-2 microglia through regulation of IL-1β production and the NF-κB signaling pathways. Mol Cell Biochem. 2019; 451: 145-53.
Kim YS, Kim JS, Kwon JS. BAY 11-7082, a nuclear factor-κB inhibitor, reduces inflammation and apoptosis in a rat cardiac ischemia-reperfusion injury model. Int Heart J. 2015; 51: 348-53.
Kohno M, Link J, Dennis LE, McCready H, Huckans M, Hoffman WF, Loftis JM. Neuroinflammation in addiction: A review of neuroimaging studies and potential immunotherapies. Pharma Biochem Behav. 2019; 179: 34-42.
Kumar A, Negi G, Sharma SS. Suppression of NF-κB and NF-κB regulated oxidative stress and neuroinflammation by BAY 11-7082 (IκB phosphorylation inhibitor) in experimental diabetic neuropathy. Biochimie 2012; 94: 1158-65.
Lee S-H, Suk K. Emerging roles of protein kinases in microglia-mediated neuroinflammation. Biochem Pharmacol. 2017; 46: 1-9.
Lim H-S, Kim J-S, Moon BC, Ryu SM, Lee J, Park G. Batryticatus bombyx protects dopaminergic neurons against MPTP-induced neurotoxicity by inhibiting oxidative damage. Antioxidants 2019; 8: 574.
Park SY, Ahn SH, Kim HH. Effects of Fructus amomi Amari, Eucommiae cortex, Bombyx batryticatus extract on improving symptoms of late-onset hypogonadism. J Physiol Pathol Korean Med. 2019; 33: 89-101.
Park G, Lee SH, Oh DS, Kim YU. Melatonin inhibits neuronal dysfunctionâ€associated with neuroinflammation by atopic psychological stress in NC/Nga atopicâ€like mouse models. J Pineal Res. 2017; 63: e12420.
Pyo JS, Kim HH, Kim KM, Kang JS. Amelioration of dry eye syndrome by oral administration of cultivated wild ginseng extract. Bangladesh J Pharmacol. 2019; 14: 61-66.
Sardi SP, Cedarbaum JM, Brundin P. Targeted therapies for Parkinson's disease: From genetics to the clinic. Mov Disord. 2018; 33: 684-96.
Schain M, Kreisl WC. Neuroinflammation in neurodegenerative disorders: A review. Curr Neurol Neurosci Rep. 2017; 17: 25.
Seppi K, Ray Chaudhuri K, Coelho M, Fox SH, Katzenschlager R, Perez Lloret S, Weintraub D, Sampaio C, collaborators of the Parkinson's Disease Update on Nonâ€Motor Symptoms Study Group on behalf of the Movement Disorders Society Evidenceâ€Based Medicine Committee, Chahine L, Hametner EM. Update on treatments for nonmotor symptoms of Parkinson's disease: An evidenceâ€based medicine review. Mov Disord. 2019; 34: 180-98.
Singh SS, Rai SN, Birla H, Zahra W, Rathore AS, Singh SP. NF-κB-mediated neuroinflammation in Parkinson’s disease and potential therapeutic effect of polyphenols. Neurotox Res. 2020; 37: 491-507.
Stephenson J, Nutma E, van der Valk P, Amor S. Inflammation in CNS neurodegenerative diseases. Immunology 2018; 154: 204-19.
Subhramanyam CS, Wang C, Hu Q, Dheen ST Microglia-mediated neuroinflammation in neurodegenerative diseases. In: Seminars in cell & developmental biology. Vol 94. Elsevier, 2019, pp 112-20.
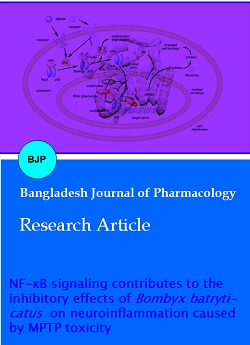