Nrf2 signaling contributes to the neuroprotective effect of Cicadidae Periostracum against 1-methyl-4-phenyl-1,2,3,6-tetrahydropyridine toxicity
Abstract
Cicadidae Periostracum has various pharmacological effects, including neuroprotective potential via nuclear receptor related-1 (NURR1) protein signaling. However, there are no studies on its antioxidative effect. The neuroprotective effect against 1-methyl-4-phenyl-1,2,3,6-tetrahydropyridine (MPTP)-induced damage to dopaminergic neurons was evaluated and explored the mechanisms of its antioxidant action, focusing on nuclear factor E2-related factor 2 (Nrf2) in a mouse model of Parkinson's disease. We measured NURR1-related neurotrophic signaling and the levels of antioxidant factors in vitro and in vivo. The effects of Cicadidae Periostracum against MPTP-induced neurotoxicity were associated with inhibition of the neurotrophic signaling pathways and up-regulation of Nrf2 pathways. Thus, Cicadidae Periostracum mitigates neurotoxicity via neurotrophic signaling not only by increasing Nrf2 activity but also by increasing antioxidant activity.
Introduction
Parkinson's disease is a neurodegenerative disease caused by diminished dopamine due to damage to dopaminergic neurons in the substantia nigra pars compacta (SNpc) of the midbrain. The first-line treatment strategy of Parkinson's disease is dopamine replacement therapy (Dauer and Przedborski, 2003) using L-DOPA, a dopamine precursor. However, this agent cannot be used for long periods due to adverse effects including loss of appetite and vomiting (Lannielli et al., 2018). Thus, it is necessary to expand treatment strategies.
Drugs derived from natural products can have fewer adverse effects from long-term use than synthetic drugs. Many researchers are focusing their research and development efforts on such products based on traditional evidence of their usage that has been described in medical books.
Nuclear factor E2-related factor 2 (Nrf2) plays an important role in neurodegenerative diseases, including Parkinson's disease (Tonelli et al., 2018; Wang et al., 2019). It binds to antioxidant response elements (AREs) to promote the transcription of phase 2 enzyme-related genes (Wang et al., 2019). These Nrf2 signaling changes are associated with abnormal redox homeostasis (Kerins and Ooi, 2018). The loss of Nrf2-mediated transcription exacerbates the vulnerability of dopami-nergic neurons to oxidative damage (Kim et al., 2020). Nrf2 knockout mice show a greater loss of dopaminer-gic neurons than wild-type mice when exposed to 1-methyl-4-phenyl-1,2,3,6-tetrahydropyridine (MPTP) (Chen et al., 2009). Thus, Nrf2 could serve as an important signaling molecule in neural protection strategies for Parkinson's disease pathogenesis.
Cicadidae Periostracum, the cast-off skin of cicada (Sun-Tae in Korea), is described in the Chungbu Category of Donguibogam, a Korean oriental medicine book (Lim et al., 2019b). Cicadidae Periostracum has been used to treat various disorders including convulsions, itchy rashes, and eczema (Park et al., 2021). Although the neuroprotective effects have been demonstrated, its effect on Nrf2 regulation has not been examined, and no study has investigated whether it can influence oxidative damage or stress via Nrf2 regulation in MPTP models of Parkinson's disease.
Here, we investigated the inhibitory effects of Cicadidae Periostracum on 1-methyl-4-phenylpyridinium (MPP+)-induced reactive oxygen species (ROS), and the expression and regulation of Nrf2, in vitro. To confirm whether Cicadidae Periostracum directly affected Nrf2, ML385, an Nrf2 transcriptional inhibitor, was used. We verified that Nrf2 pre-inhibition with ML385 neutra-lized the effects of Cicadidae Periostracum on the neurotrophic factors induced by MPTP in vivo.
Materials and Methods
Chemicals
Ethanol, dimethyl sulfoxide, nerve growth factor, MPTP, MPP+, phosphate-buffered saline, and sodium citrate buffer were purchased from Sigma-Aldrich (USA). Roswell Park Memorial Institute medium (RPMI), penicillin-streptomycin, and fetal bovine serum were purchased from Gibco (USA). Rabbit anti-heme oxygenase 1 (HO-1) and quinone oxidoreductase 1 (NQO1) antibodies were purchased from Abcam (UK). Goat and rabbit anti-tyrosine hydroxylase (TH), dopamine transporter (DAT), and vesicular monoamine transporter 2 (VMAT2) antibodies were purchased from Millipore Bioscience Research (USA). The 2-CAT (N-D) Research ELISA was purchased from Rocky Mountain Diagnostics (USA). TransAM assays for Nrf2 were purchased from Active Motif (USA). The Nrf2 inhibitor, ML385, was purchased from Axon Medchem (Netherlands). All other reagents used were of guaranteed or analytical grade.
Preparation of the extract and treatments of differentiated PC12 cells
Standardized Cicadidae Periostracum extracts were prepared according to previously published methods and were stored at 4°C (3-18-0038 code) in the Korea Institute of Oriental Medicine (Lim et al., 2019; Park et al., 2021). The PC12 cell line was obtained from the Korean Cell Line Bank and maintained in RPMI medium supplemented with 10% fetal bovine serum and 1% penicillin-streptomycin. To differentiate PC12 cells, the culturing medium was changed every 2–3 days, and cultured cells were treated with nerve growth factor for 7 days. Differentiated PC12 cells were then pretreated with Cicadidae Periostracum (0.1–50 μg/mL) for 1 hour, followed by MPP+ (100 μg/mL) for 23 hours.
Measurement of ROS and cytotoxicity
The release of lactic acid dehydrogenase (LDH) was used to assess cytotoxicity. LDH was determined using a CytoScan according to the manufacturer's instruc-tions. The experiment was conducted using a culture medium obtained after Cicadidae Periostracum treatment. Briefly, the culture medium (100 μL) was removed, centrifuged, transferred to an analysis tube, then reacted with the dye solution (100 μL) in the dark for 30 min. The absorbance of the solution was mea-sured at 490 nm. For the ROS assay, differentiated PC12 cells were pre-treated with Cicadidae Periostracum (0.1–50 μg/mL) for 1 hour, stimulated with MPP+ (100 μg/mL), then incubated with 20 μM 2,7-dichlorofluorescein diacetate at 30 min at 37°C. The fluorescence was measured at 485/530 nm.
Drug administration
Mice were assigned to each of the following 10 groups: 1) control; 2) MPTP; 3) MPTP + Cicadidae Periostracum 5 mg/kg/day; 4) MPTP + Cicadidae Periostracum 25 mg/kg/day; 5) MPTP + Cicadidae Periostracum 25 mg/kg/day + ML385 10 mg/kg/day; 6) MPTP + ML385 10 mg/kg/day; 7) control; 8) Cicadidae Periostracum 1 mg/kg/day; 9) Cicadidae Periostracum 5 mg/kg/day; and 10) Cicadidae Periostracum 25 mg/kg/day. Cicadidae Periostracum was dissolved in normal saline and administered to mice for five consecutive days. Control mice received the same volume of normal saline for the same period. MPTP (20 mg/kg; dissolved in saline) was injected four times into the abdominal cavity at 2 hours intervals on day 3 of Cicadidae Periostracum treatment; control mice received the same volume (0.25 mL) of saline.
Antioxidant protein level assays
Differentiated PC12 cells or SNpc from brain samples were lysed in RIPA lysis and extraction Buffer contain-ing a protease inhibitor cocktail. Protein concentrations in the tissue extracts were determined using the Bradford method. Western blots were prepared similarly to previously published methods (Lim et al., 2019a,b; Lim et al., 2020). Glutathione (GSH) levels were measured by an enzyme-linked immunosorbent assay.
Trans-AM Nrf2 DNA-binding activity and dopamine contents
The efficiency of Nrf2 DNA-binding activity was evaluated using a commercially available Trans-AM Nrf2 kit (50296; Active Motif, USA). Briefly, the nuclear extract was incubated with competitor oligonucleotides for the ARE. Then, the primary Nrf2 antibody was added at 1:1000, washed after 1 hour, and the secondary horseradish peroxidase-conjugated antibody was added at 1:1000 for 1 hour. After reacting by adding the developing solution for x hour, the stop solution was added and the absorbance was measured at 450 nm.
Striatal dopamine contents were assessed using the 2-CAT (A-N) Research ELISA fluorometric assay kit following the manufacturer's protocol. Briefly, each tissue sample was assessed separately according to its volume. When the volume was less than 100 μL, ultra-pure water was added to reach a final volume of 100 μL. We then used the TE buffer and the acylation buffer for acylation. Afterward, samples and standards were added to each well of a 96-well microtiter plate and incubated with the enzyme solution. After substrate treatment to form the conjugate and incubation for 20 min, the stop solution was added the absorbance was measured at 450 nm.
Statistical analyses
All statistical parameters were calculated using Prism 5.0 software (Graphpad Software, USA). Values are expressed as means ± standard error of the mean. Statistical comparisons between the different treatments were performed using a one-way analysis of variance with Tukey's multiple comparison post-test. A p-value <0.05 was considered to be statistically significant.
Results
Effects on MPP+-induced neurotoxicity in differentiated PC12 cells
Treatment with Cicadidae Periostracum at a concentration of 100 μg/mL, but not at 1–50 μg/mL, decreased the viability of PC12 and differentiated PC12 cells 24 hours after treatment (data not shown). Thus, further experiments were performed with 1–50 μg/mL Cicadidae Periostracum. To investigate the effects on cytotoxicity, we determined the LDH level in differentiated PC12 cells. Cells exposed to 100 µg/mL MPP+ a showed a significant increase in the LDH level (153 ± 4.5%) compared with control cells. In contrast, cells treated with 0.1–50 μg/mL Cicadidae Periostracum before exposure to MPP+ were protected against injury (125 ± 6.6% – 103 ± 2.4%) compared with control cells (Figure 1A).
Figure 1: Effects of Cicadidae Periostracum (CP) on cytotoxicity and reactive oxygen species (ROS) levels. (A) Effects of CP on 1-methyl-4-phenylpyridinium (MPP+)-mediated toxicity to differentiated PC12 cells. (B) ROS levels were measured using the 2,7-dichlorofluorescin diacetate assay. Values are presented as means ± S.E.M. ap<0.001 compared with the control group; bp<0.001 compared with the MPP+-treated group
Effect on MPP+-induced ROS generation in differentiated PC12 cells
Exposure to 100 µg/mL MPP+ significantly elevated the ROS levels in differentiated PC12 cells (166 ± 4.0%) compared with control cells. Cicadidae Periostracum pre-treatment at 1–50 µg/mL inhibited ROS generation (132 ± 6.4% – 82.4 ± 3.4%) compared with the control cells (Figure 1B).
Effects on Nrf2 activity and the induction of oxidative defense proteins
The Nrf2/ARE DNA-binding ability, assessed using the Trans-AM-ELISA assay, was increased by Cicadidae Periostracum treatments in the nuclear fraction of differentiated PC12 cells (133 ± 4.6% – 195 ± 25.3% of control) and the SNpc of mouse brains (106 ± 0.5% – 190 ± 11.4% of control) (Figure 2 and Figure 4). Moreover, the HO-1, NQO1, and GSH levels were elevated to 116 ± 2.9% – 153 ± 2.6%, 125 ± 4.5% – 185 ± 9.8%, and 134 ± 7.8% – 347 ± 9.1% at 1, 10, and 50 µg/mL Cicadidae Periostracum, respectively, compared to those in control differentiated PC12 cells (Figure 3A–D). Additionally, the HO-1, NQO1, and GSH levels were elevated to 96 ± 4.0% – 241 ± 11.9%, 264 ± 6.5% – 283 ± 7.4%, and 133 ± 14.7% – 167 ± 13.1% at 1, 5, and 25 mg/kg Cicadidae Periostracum, respectively, compared to those in the control SNpc (Figure 3A–D).
Figure 2: Effects of Cicadidae Periostracum (CP) on the nuclear factor E2-related factor 2 (Nrf2) level. Nrf2 DNA-binding activity was measured using an enzyme-linked immunosorbent assay in differentiated PC12 cells. ap<0.05 and bp< 0.01 compared with the control group
Figure 3: Effects of Cicadidae Periostracum (CP) on oxidative defense protein levels using differentiated PC12 cells and substantia nigra pars compacta. Levels of (A) heme oxygenase-1 (HO-1) and (B) quinone oxidoreductase 1 (NQO1) were measured by Western blotting and normalized to β-actin. (C) Glutathione (GSH) levels were measured by an enzyme-linked immunosorbent assay. (D) Representative western blot. ap<0.01, bp<0.001 and cp<0.05 compared with the control group
Figure 4: Effects of Cicadidae Periostracum (CP) on the nuclear factor E2-related factor 2 (Nrf2) level in the substantia nigra pars compacta. Nrf2 DNA-binding activity was measured using an enzyme-linked immunosorbent assay. ap<0.001 compared with the control group
Effect of an Nrf2 inhibitor against the neuroprotective effect of Cicadidae Periostracum
Pretreatment with Cicadidae Periostracum significantly up-regulated the expression of oxidative defense proteins associated with the inhibition of neurological dysfunction and the reduction of dopaminergic neurodegeneration in the SNpc after MPTP intoxication. Pre-inhibiting the Nrf2 pathway might neutralize the protective effect of Cicadidae Periostracum against MPTP-induced neurotoxicity. Thus, we measured the levels of dopaminergic neurotrophic factors in the SNpc. Exposure to MPTP decreased the TH, DAT, and VMAT2 levels (27.4 ± 3.5%, 32.2 ± 7.8%, and 33.8 ± 4.1%, respectively) compared with the control. In contrast, pretreatment with 50 mg/kg ML385 impaired the effects of MPTP on TH, DAT, and VMAT2 (5.0 ± 4.4%, 9.0 ± 2.7%, and 11.6 ± 3.1%, respectively) compared with the control. The positive effect of Cicadidae Periostracum on these neurotrophic factors following MPTP intoxication (37.0 ± 9.6% – 108 ± 12.9%, 60.5 ± 8.26% – 69.9 ± 8.1%, and 63.9 ± 20.3% – 115 ± 11.9%, for TH, DAT, and VMAT2, respectively, compared with the control group) was significantly neutralized by pre-inhibiting Nrf2 signaling with 50 mg/kg ML385 (50.4 ± 6.6%, 30.1 ± 2.2%, and 53.1 ± 15.9%, for TH, DAT, and VMAT2, respectively, compared with the control) (Figure 5A–D).
Figure 5: Nuclear factor E2-related factor 2 (Nrf2) inhibition neutralizes the protective effect of Cicadidae Periostracum (CP) following 1-methyl-4-phenyl-1,2,3,6-tetrahydropyridine (MPTP) treatment in vivo. The Nrf2 inhibitor, ML385 (50 mg/kg), or saline were injected intraperitoneally once daily 30 min before CP treatment in the MPTP intoxication model. Seven days after the last MPTP treatment, the expression levels of (A) tyrosine hydroxylase (TH), (B) dopamine transporter (DAT), and (C) vesicular monoamine transporter 2 (VMAT2) in the substantia nigra pars compacta were measured by Western blotting. Dopamine levels were measured using an enzyme-linked immunosorbent assay. (D) Representative Western blot. ap<0.01 and bp<0.001 compared with the control group; cp<0.05, dp<0.01, and ep<0.001 compared with the MPTP-treated group; fp<0.05 and gp<0.01 compared with the MPTP + CP 25 mg/kg-treated group
Exposure to MPTP decreased the dopamine level (1.9 ± 0.1 nmol/mL), whereas treatment with 50 mg/kg ML385 augmented the MPTP-induced effect (0.4 ± 0.1 nmol/mL). Moreover, the positive effect of 25 mg/kg Cicadidae Periostracum on the dopamine level after MPTP-intoxication (3.0 ± 0.4 nmol/mL) was significantly neutralized by pre-inhibiting Nrf2 signaling with 50 mg/kg ML385 (1.55 ± 0.15 nmol/mL) (Figure 6). Although the Nrf2 inhibitor alone did not significantly affect the dopaminergic neurotrophic factors TH, DAT, and VMAT2, applying this inhibitor to the MPTP model caused further neuronal dysfunction. Thus, Cicadidae Periostracum does not exert its neuroprotective effects after MPTP intoxication and Nrf2 inhibitor treatment, and the effects of Cicadidae Periostracum are likely related to the activation of Nrf2 signaling.
Figure 6: Nuclear factor E2-related factor 2 (Nrf2) inhibition neutralizes the protective effect of Cicadidae Periostracum (CP) following 1-methyl-4-phenyl-1,2,3,6-tetrahydropyridine (MPTP) treatment in vivo. The Nrf2 inhibitor, ML385 (50 mg/kg), or saline were injected once daily intraperitoneally 30 min before CP treatment in the MPTP intoxication model. Dopamine levels in the striatum were measured using an enzyme-linked immunosorbent assay. ap<0.001 compared with the control group; bp<0.01 and cp<0.001 compared with the MPTP-treated group; dp<0.001 compared with the MPTP + CP 25 mg/kg-treated group
Discussion
The nuclear receptor related-1 (Nurr1) protein regulates genes that are critical to the development, maintenance, and survival of dopaminergic neurons. In addition, Nurr1 plays a fundamental role in maintaining dopamine homeostasis by regulating the transcription of genes involved in dopamine synthesis, reabsorption, and packaging (Jankovic et al., 2005). Nurr1 is also known to regulate the survival of dopamine neurons by stimulating gene coding, anti-inflammatory reactions, and oxidative stress management (Jankovic et al., 2005). We reported previously that Cicadidae Periostracum up-regulates Nurr1 expression and regulates the expression of neurotrophic factors (Lim et al., 2019b). Furthermore, effects on the activity of Nurr1 by Cicadidae Periostracum indicate that neural protection signals are associated with mitochondria-mediated apoptosis and neuroinflammation (Lim et al., 2019b). Thus, Nurr1 has a close association with dopamine neurons, and Cicadidae Periostracum could exert its anti-Parkinson's disease effect by regulating its activity (Lim et al., 2019b).
Nurr1 shuttling between the cytosol and nucleus is controlled by specific nuclear import and export signals that can be affected by oxidative stress to disturb the balance of Nurr1 in favor of cytoplasmic accumulation. The Nurr1 gene is also upregulated by the increased expression of ROS-removal genes such as SOD1 and Abl2. Nurr1 is an essential mediator of CREB-dependent neuroprotection under oxidative stress. Nurr1 overexpression protects neuronal stem cells from oxidative damage by inhibiting caspase-3 and -11.
Overall, Nurr1 and oxidative stress regulation are closely related. In the current study, we focused on the regulation of the oxidative stress gene, Nrf2, to demons-trate the efficacy of Cicadidae Periostracum. Nrf2, a master regulator of the antioxidant response in neu-rons, controls antioxidant response signaling, involving SOD, catalase, GSH, HO-1, and NQO1 (Zhao et al., 2009). Research on the dopamine transporter in Nrf2 knockout mice has shown that Nrf2 activation is a key factor in regulating cell-protective gene expression pathways (Burton et al., 2006). Nrf2 activators modulate the Nrf2/ARE/HO-1 and NQO1 pathways to counter-act apoptosis, neuroinflammation, and neurotrophic dysfunction caused by neurotoxic agents (Lim et al., 2020; Burton et al., 2006; Oh et al., 2013). Thus, the function of Nrf2 is important in the pathology of Parkinson's disease. Our results elucidating the basic effects of Cicadidae Periostracum on Nrf2 activity suggest that Nrf2 and Nurr1 may be interrelated. Furthermore, we assessed the effect of Cicadidae Periostracum after Nrf2 inhibitor treatment to confirm the Nrf2 signaling mechanism in the Parkinson's disease mouse model.
In this study, Cicadidae Periostracum increased the activation of Nrf2 and levels of the proteins it regulates (i.e., HO-1, NQO1, and GSH) in differentiated PC12 cells and the SNpc. Drugs that increase the expression of antioxidants generally inhibit ROS. This has a bene-ficial effect on dopaminergic neurons. In this study, Cicadidae Periostracum down-regulated ROS levels in differentiated PC12 cells by increasing antioxidant protein expression through the upregulation of Nrf2 activity. Our findings suggest that MPP+ inhibits the development of neurotoxin-induced cellular damage by decreasing ROS levels. Furthermore, we showed that the pre-inhibition of Nrf2 with ML385 could neutralize the effects of Cicadidae Periostracum against the neurotrophic factors and striatal dopamine level induced by MPTP. ML385 was used in a previous Nrf2 inhibitory study (Lim et al., 2020). It inhibits Nrf2 through its ability to block Nrf2 transcriptional activity. In particular, ML385 binds to the basic leucine zipper domain (Neh1) of Nrf2 and inhibits its expression by preventing the protein complex from binding to the regulatory DNA binding sequence (Singh et al., 2016).
Conclusion
The reduction in striatal dopamine loss and neurotrophic factor expression by Cicadidae Periostracum is dependent on Nrf2 and related antioxidant activities.
Ethical Issue
These studies were reviewed and approved by the institutional animal care ethical committee of the Korea Institute of Oriental Medicine, Republic of Korea (approval numbers KIOM-20-003 and -078) and performed according to the guidelines of the Care and Use of Laboratory Animals in Ethics Committee of the Korea Institute of Oriental Medicine. Male C57BL/6 mice (8 weeks of age, 23–24 g) were purchased from Doo Yeol Biotech (Seoul, Korea) and maintained under temperature- and light-controlled conditions (20–23°C, 12-hours light/12-hours dark cycle) with food and water provided ad libitum. All mice were acclimatized for 7 days before drug administration.
References
Burton NC, Kensler TW, Guilarte TR. In vivo modulation of the Parkinsonian phenotype by Nrf2. Neurotoxicology 2006; 27: 1094-100.
Chen PC, Vargas MR, Pani AK, Smeyne RJ, Johnson DA, Kan YW, Johnson JA. Nrf2-mediated neuroprotection in the MPTP mouse model of Parkinson's disease: Critical role for the astrocyte. Proc Nati Acad Sci USA. 2009; 106: 2933-38.
Dauer W, Przedborski S. Parkinson's disease: Mechanisms and models. Neuron 2003; 39: 889-909.
Jankovic J, Chen S, Le W. The role of Nurr1 in the development of dopaminergic neurons and Parkinson's disease. Prog Neurobiol. 2005; 77: 128-38.
Kerins MJ, Ooi A. The roles of NRF2 in modulating cellular iron homeostasis. Antioxid Redox Signal. 2018; 29: 1756-73.
Kim S, Viswanath ANI, Park JH, Lee HE, Park AY, Choi JW, Kim HJ, Londhe AM, Jang BK, Lee J, Hwang H, Lim SM, Pae AN, Park KD. Nrf2 activator via interference of Nrf2-Keap1 interaction has antioxidant and anti-inflammatory properties in Parkinson's disease animal model. Neuropharmacology 2020; 167: 107989.
Lannielli A, Bido S, Folladori L, Segnali A, Cancellieri C, Maresca A, Massimino L, Rubio A, Morabito G, Caporali L, Tagliavini F, Musumeci O, Gregato G, Bezard E, Carelli V, Tiranti V, Broccoli V. Pharmacological inhibition of necroptosis protects from dopaminergic neuronal cell death in Parkinson’s disease models. Cell Rep. 2018; 22: 2066-79.
Lim HS, Kim JS, Moon BC, Ryu SM, Lee J, Park G. Batryticatus bombyx protects dopaminergic neurons against MPTP-induced neurotoxicity by inhibiting oxidative damage. Antioxidants 2019a; 8: 574.
Lim HS, Kim SJ, Moon BC, Choi G, Ryu SM, Lee J, Ang MJ, Jeon M, Moon C, Park G. Cicadidae Periostracum, the cast-off skin of cicada, protects dopaminergic neurons in a model of Parkinson’s disease. Oxi Med Cell Longev. 2019b; 2019.
Lim HS, Moon BC, Lee J, Choi G, Park G. The insect molting hormone 20-hydroxyecdysone protects dopaminergic neurons against MPTP-induced neurotoxicity in a mouse model of Parkinson's disease. Free Radic Biol Med. 2020; 159: 23-36.
Lim HS, Jang Y, Moon BC, Park G. NF-κB signaling contributes to the inhibitory effects of Bombyx batryticatus on neuroinflammation caused by MPTP toxicity. Bangladesh J Pharmacol. 2021; 16: 96-102.
Liu XX, Zhu XY, Yu Z. Mulberrofuran G inhibits proliferation and migration by inactivating JAK2/STAT3 signaling in lung cancer cells. Bangladesh J Pharmacol. 2021; 16: 134-40.
Oh H, Hur J, Park G, Kim HG, Kim YO, Oh MS. Sanguisorbae Radix protects against 6â€ydroxydopamineâ€induced neurotoxicity by regulating NADPH oxidase and NFâ€E2â€related factorâ€2/heme oxygenaseâ€1 expressions. Phytother Res. 2013; 27: 1012-17.
Park G, Moon BC, Ryu SM, Kim WJ, Lim HS. Cicadidae Periostracum attenuates atopic dermatitis symptoms and pathology via the regulation of NLRP3 inflammasome activation. Oxi Med Cell Longev. 2021; 2021.
Shen S, Hou Y, Wu Y. Tongxinluo preserves the renal function in diabetic kidney disease via protecting the HK-2 cells from the patterns of programmed cell death. Bangladesh J Pharmacol. 2021; 16: 52-64.
Singh A, Venkannagari S, Oh KH, Zhang YQ, Rohde JM, Liu L, Nimmagadda S, Sudini K, Brimacombe KR, Gajghate S, Ma J, Wang A, Xu X, Shahane SA, Xia M, Woo J, Mensah GA, Wang Z, Ferrer M, Gabrielson E, Li Z, Rastinejad F, Shen M, Boxer MB, Biswal S. Small molecule inhibitor of NRF2 selectively intervenes therapeutic resistance in KEAP1-deficient NSCLC tumors. ACS Chem Biol. 2016; 11: 3214-25.
Tonelli C, Chio IIC, Tuveson DA. Transcriptional regulation by Nrf2. Antioxid Redox Signal. 2018; 29: 1727-45.
Wang GQ., Zhang B, He XM., Li DD, Shi JS, F. Zhang F. Naringenin targets on astroglial Nrf2 to support dopaminergic neurons. Pharmacol Res. 2019; 139: 452-59.
Zhao F, Wu T, Lau A, Jiang T, Huang J, Wang XJ, Chen W, Wong PK, Zhang D. Nrf2 promotes neuronal cell differentiation. Free Radic Biol Med. 2009; 47: 867-79.
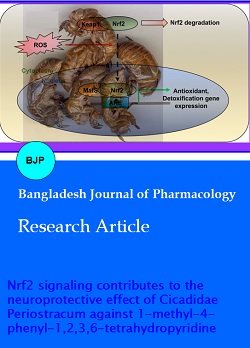