Synthesis, in silico analysis and antidepressant activity of 1,3,4-oxadiazole derivatives
Abstract
The compounds 1,3,4-oxadiazole derivatives (1-8) were synthesized by the cyclization of 4-hydroxybenzhydrazide (1) with various substituted aromatic aldehydes (2) using FeCl3 as catalyst and methanol as a solvent medium. The structures of the newly synthesized compounds were assigned based on FT-IR, 1H-NMR, and mass spectral data. In vivo antidepressant activity was performed by tail suspension test and forced swimming test models. Using the Schrodinger Maestro, the in silico analysis was performed and docked to the glycogen synthase kinase 3β binding site (PDB: 3GB2). Compounds 8 [4,4'-(1,3,4-oxadiazole-2,5-diyl)diphenol] and 3 [3-(5-(4-hydroxyphenyl)-1,3,4-oxadiazol-2-yl) phenol] showed both potent inhibitory activity against GSK-3β with a docking score of -7.800 kcal/mol as well as good antidepressant activity in both tail suspension and forced swimming tests models. The synthesized derivatives showed good antidepressive potential.
Introduction
1,3,4-Oxadiazoles are an important class of compounds in medicinal chemistry because of their interesting diversified biological applications (Arshad et al., 2014). The activities include antibacterial (Maslat et al., 2002), cytotoxic (Puthiyapurayil et al., 2012), anti-cancer (Aboraia et al., 2006), antitubercular (Macaev et al., 2011), anti-inflammatory (Omar et al., 2006), antidepressant (Tantray et al., 2018), anticonvulsant (Omar et al., 2006), analgesic (Bhandari et al., 2008), hypolipidemic (Patel et al., 2014), anti-fungal (Chen et al., 2007), etc,. There are some examples of compounds currently used in clinical practice containing 1,3,4-oxadiazole moiety, such as raltegravir (used to treat HIV infection), nesapidil (antiarrhythmic), furamizole (antibacterial), tiodazocin (antihypertensive) and, zibotentan (anti-cancer).
Depression is a life-threatening psychological illness and is linked to impaired, poor quality of life, raised health-related costs, and is considered a major risk factor for many ailments, including cardiovascular, metabolic, and psychiatric disorders. (Lou, 2003; Otte, 2016; Casey et al., 2005). Current drug therapy approaches have limited effectiveness and are associated with many adverse health effects. Consequently, a better awareness of this depression's pathophysiology alongside the development of innovative and advanced therapies contributes significantly (Predictable, 2006; Tamminga et al., 2002).
Glycogen synthase kinase 3 (GSK-3), discovered in the late 1970s, occurs in two isoforms: GSK-3 alpha and GSK-3 beta, where β isozyme predominates the human brain. These receptors, a proline-directed serine-threonine kinase, are constitutively an active protein kinase requiring primed phosphorylation of most of its substrates (Beurel et al., 2015) increases activation of GSK-3β and has been found in the prefrontal cortices of post-mortem depressed suicide victim (Karege et al., 2012). Glycogen synthase kinase 3β (GSK-3β) inhibition is anticipated to be a desired pharmacological approach for treating depression. Lithium is used to treat bipolar disorder was reported to inhibit around 25% of total GSK-3 activity (Kramer et al., 2012). Both fluoxetine, which is a specific serotonin reuptake inhibitor (SSRI), and Imipramine, a tricyclic antidepressant (TCA), have been shown to increase the phospho-Ser9-GSK-3β level, which otherwise reduces the effects of antidepressants when phosphorylation is impeded (Li et al., 2004).
This inhibition has been achieved by the high binding affinity of Imipramine to 5-HT2A receptor, SERT (serotonin reuptake transporter), and norepinephrine transporter (NET). Norepinephrine acting through protein kinase A and C, and 5-HT acting through receptors other than 5-HT2A receptor, inactivate GSK3-β, whereas the blockade of 5-HT2A receptor prevents the inhibition of AKT. Similarly, fluoxetine inhibits SERT and increases the concentrations of 5-HT in the synaptic cleft (Duda et al., 2020).
Further, 2,5-disubstituted-1,3,4-oxadiazoles are applied to various biological activities due to the -N=C-O- bonding in the ring (Desai, 2016; Roy, 2017), Considering the broad range of biological attributes, it was contemplated to synthesize a new series of 1,3,4-oxadiazole derivatives and evaluated for their in vivo antidepressant activity in mice. The compounds have been docked in the groove binding side of the GSK-3β receptor to verify their binding affinity and inhibition effect.
Materials and Methods
The required chemicals and solvents were procured from Sigma Aldrich, India and Himedia India. Melting points were measured by digital melting point equipment from Equiptronics and were uncorrected. Thin layer chromatography plates, precoated with silica gel G, were used to monitor the completion of the reaction (solvent system: ethyl acetate: methanol (2:1 v/v). IR spectra were measured using KBr disc on Alpha Bruker FT-IR infrared spectrophotometer (cm-1). Agilent 400MR DD2 spectrometer was used to generate 1H-NMR spectra with d6-DMSO or CDCl3 solvent and tetramethylsilane as an internal standard. In δ ppm, the chemical shift values were recorded, and the mass spectrum was acquired using a Waters LC-MS/MS.
General procedure for the synthesis of 1,3,4-oxadiazoles (1-8)
4-Hydroxybenzhydrazide (0.01 mol) (1) and substituted aromatic aldehydes (2) (0.01 mol) were dissolved in 30 ml of methanol and a of pinch of FeCl3 is added and the reaction contents were refluxed for 6-8 hours. After refluxing, the reaction contents were poured into crushed ice and stirred vigorously. The crude product obtained was washed with water and recrystallized from ethanol.
In silico analysis
The molecular modeling studies (Lipinski’s RO5, molecular docking, ADMET properties) were carried out using Schrodinger 2018-3 suite device Maestro 11.7.012, (Ligprep, Glide XP docking, QikProp). The three-dimensional crystal structure of Human GSK-3β inhibitor complex with 2-methyl-5-(3-{4-[(S)-methyl-sulfinyl]phenyl}-1-benzofuran-5-yl)-1,3,4-oxadiazole (PDB ID: 3GB2) was downloaded from the data bank of protein molecules database with a recorded resolution of 2.40 Anstrom (Saitoh et al., 2009). In the Schrodinger suite of tools, the structures of the ligands were subjected to Maestro's LigPrep module before the molecular docking, and their structures were transformed from 2D to 3D by including ionization, stereochemical, tautomeric variations, including energy minimization and optimized for their geometry, desalinated and corrected for their chiralities and missing atoms of hydrogen. The protein 3GB2 was prepared using a protein preparation wizard, and the hydrogen atoms were optimized throughout the protein structure. At the groove binding site of the 3GB2 protein, the synthesized derivatives of 1,3,4-oxadiazoles were docked, and the docking process was performed using extraprecision (XP) mode. Using the Molinspiration (http://www.molinspiration.com/cgi-bin/properties), bioactivity scores for G-protein coupled receptor (GPCR) ligand, ion channel modulator, kinase inhibitor, nuclear receptor ligand, protease inhibitor and enzyme inhibitor were measured. The toxicity predictions of the synthesized derivatives were carried out using lazy structure-activity relationships (LAZAR) software that produces local QSAR (quantitative structure-activity relationship) models for each predictable compound.
Antidepressant activity
Animals
The animals were procured from Nitte University Center for Animal Research and Experimentation, Mangalore, India. Swiss albino mice weighing around 20–25 g have been collected. Animals were acclimatized, two weeks before the start of the study. Housing conditions were maintained at 25 ± 2°C for 12 hours day/night cycles, and the animals were supplied with regular commercial pellet feed and water.
Acute toxicity studies
Standard husbandry conditions were maintained for the acute toxicity studies of 1,3,4-oxadiazole derivatives (OECD, 2001) on female albino mice (20-25 g). Before the experimentation, the animals were fasted overnight and were treated with a single dose of 1,3,4-oxadiazoles derivatives and monitored for 48 hours for mortality (short-term toxicity). The next doses were calculated based on the short-term toxicity profile according to OECD guidelines No. 425 (Chemicals et al., 2003).
Box 1: Tail Suspension Test
Principle
In the tail suspension test, antidepressant effect of an experimental drug in investigated.
Requirements
Synthesized 1,3,4-oxadiazole derivatives (100 mg/kg; 0.5 mL/kg); Imipramine (standard drug; 10 mg/kg); tween 80 (1%);
Procedure
Step 1: The mice were assigned to different groups on the day of the experiment (n=6 for each group), and the test substance was administered orally 60 min prior to the test in a fixed rotation and was placed back in their home cages.
Step 2: The mice were then hung, using adhesive tape, at the height of 65 cm from the floor, and 1 cm from the tail tip.
Step 3: The mice were allowed to hang for 6 min, and during the last 4 min, the time of immobility was observed.
Calculation
The percentage change from control was calculated using the formula below.
% Change of immobility = [(test/control) × 100) - 100]
References
Steru et al., 1985; Aslam, 2016
References (Video)
Can et al., 2012; Haq et al., 2019
Forced swimming test
In forced swimming test, mice are forced to swim in a small cylinder where they are unable to escape. The suspension of synthesized 1,3,4-oxadiazole derivatives (100 mg/kg) and standard drug in 1% aqueous solution of tween 80 was administered (p. o.) to mice at a volume of 0.5 mL/body weight, where control was maintained, and Imipramine (10 mg/kg) was employed as the standard drug. The mice were assigned to different groups on the day of the experiment (n=6 for each group), and the test substance was administered 60 min before the test in a fixed rotation and was placed back in their home cages. In an open cylindrical container (25 cm in height), 10 cm in diameter containing water to a height of 18 cm and observed for 6 min, the mice were individually forced to swim. Animals were allowed to swim for 6 min, and during the last 4 min, the period of immobility was observed. In the first 2 min, the animals will demonstrate initial vigorous struggle. During the last 4 min, the immobility time was recorded (Can et al., 2012). The percentage change from control was calculated using the formula below.
% Change of immobility = [(test/control) × 100) - 100]
Statistical analysis
The findings are described as mean ± SEM, n=6.For a statistical demonstration followed by Dunnett's multiple comparison test, a one-way ANOVA was used, and p<0.05 was deemed significant. The above data is presented as mean ± SEM, n=6.
Results
Chemistry
The reaction sequence leading to the formation of title compounds is shown in Scheme 1. The physical data of the compounds (1-8) is given in Table I.
Scheme 1: Reaction sequence leading to the formation of 8 compounds
Table I: Physicochemical properties of compounds (1-8)
Compound | R-CHO | Molecular Formula |
MR [cm3/mol] | tPSA (<140) [â„«] | Polariz ability [â„«3] | Nrobs (<10) | Volume (<2000) [cm3] |
---|---|---|---|---|---|---|---|
1 | 4-Cl | C14H9Cl N2O2 | 70.7 ± 0.3 | 59.2 | 28.0 ± 0.5 10-24 | 2 | 221.7 |
2 | 4-OCH3 | C15H12N2O3 | 72.5 ± 0.3 | 68.4 | 28.7 ± 0.5 10-24 | 3 | 233.7 |
3 | 3-OH | C14H10N2O3 | 67.7 ± 0.3 | 79.4 | 26.8 ± 0.5 10-24 | 2 | 216.2 |
4 | 3-NO2 | C14H9N3O4 | 72.3 ± 0.3 | 105 | 28.7 ± 0.5 10-24 | 3 | 231.5 |
5 | 4-N(CH3)2 | C16H15N3O2 | 80.1 ± 0.3 | 62.4 | 31.8 ± 0.5 10-24 | 3 | 254 |
6 | 2-Cl | C14H9Cl N2O2 | 70.7 ± 0.3 | 59.2 | 28.0 ± 0.5 10-24 | 2 | 221.7 |
7 | C6H5 | C14H10N2O2 | 65.8 ± 0.3 | 59.2 | 26.1 ± 0.5 10-24 | 2 | 208.1 |
8 | 4-OH | C14H10N2O3 | 67.7 ± 0.3 | 79.4 | 26.8 ± 0.5 10-24 | 2 | 216.2 |
Standard (Imipramine) |
--- | ---- | 88.9 ± 0.3 | 6.5 | 35.3 ± 0.5 10-24 | 1 | 269.2 |
4-(5-(4-chlorophenyl)-1,3,4-oxadiazol-2-yl)phenol (1): M.P.: 260-262 ºC, Yield: 82.08%. FT-IR (KBr, v, cm-1): 3239 (OH), 2925 (C-H), 1600 (C=N), 1549 (C=C), 1076 (C-O-C), 746 (C-Cl). 1H-NMR (DMSO-d6, 400 MHz) δ = 6.91-8.41 (m, Ar-H, 8H), 11.86 (s, OH, 1H). MS (m/z): 273.69 (M+1). 82.08%
4-(5-(4-methoxyphenyl)-1,3,4-oxadiazol-2-yl)phenol (2): M.P.: 246-248 ºC. Yield: 75.05% FT-IR (KBr, v, cm-1): 3277 (OH), 1603 (C=N), 1550 (C=C),2926 (C-H),1030 (C-O-C). 1H-NMR (DMSO-d6, 400 MHz) δ = 3.78 (s, OCH3, 3H), 6.93-8.38 (m, Ar-H, 8H), 11.74 (s, OH, 1H). MS (m/z): 269.27 (M+1).
3-(5-(4-hydroxyphenyl)-1,3,4-oxadiazol-2-yl)phenol (3): M.P.: 218-220ºC. Yield: 78.46% FT-IR (KBr, v, cm-1): 3237 (OH), 2962 (C-H), 1606 (C=N), 1521 (C=C), 1080 (C-O-C). 1H-NMR (DMSO-d6, 400 MHz) δ = 6.93-8.38(m, Ar-H, 8H), 11.75 (s, OH, 1H), 11.86 (s, OH, 1H). MS (m/z): 255.24 (M+1). 78.46%
4-(5-(3-nitrophenyl)-1,3,4-oxadiazol-2-yl) phenol (4): M.P.: 182-184ºC. Yield: 79.87%. FT-IR (KBr, v, cm-1): 3226 (OH), 2928 (C-H), 1606 (C=N), 1552 (C=C), 1032 (C-O-C). 1H-NMR (DMSO-d6, TMS, 400 MHz) δ 6.93-8.55(m, Ar-H, 8H), 11.67(s,OH,1H). MS (m/z):284.35(M+1).
4-(5-(4-(dimethyl amino) phenyl)-1,3,4-oxadiazol-2-yl) phenol (5): M.P.: 236-238ºC. Yield: 77.37%. FT-IR (KBr, v, cm-1): 3276 (OH), 2962 (C-H), 1626 (C=N), 1555 (C=C), 1087 (C-O-C). 1H-NMR (DMSO-d6, TMS, 400 MHz) δ 2.55 (s, 2XCH3, 6H), 6.72-8.29 (m, Ar-H, 8H), 11.60(s, OH, 1H). MS (m/z): 282.33 (M+1).
4-(5-(2-chlorophenyl)-1,3,4-oxadiazol-2-yl)phenol (6): M.P.: 255-257ºC. Yield: 81.88%. FT-IR (KBr, v, cm-1): 3214 (OH), 2928 (C-H), 1600 (C=N), 1545 (C=C), 1087 (C-O-C), 752 (C-Cl). 1H-NMR (DMSO-d6, TMS, 400 MHz) δ ppm= 6.92-8.84 (m, Ar-H, 8H), 11.77 (s, OH, 1H). MS (m/z): 273.32 (M+1).
4-(5-phenyl-1,3,4-oxadiazol-2-yl) phenol (7): M.P.: 205-207ºC. Yield: 76.33%. FT-IR (KBr, v, cm-1): 3214 (OH), 2933 (C-H), 1606 (C=N), 1558 (C=C), 1063 (C-O-C). 1H-NMR (DMSO-d6, TMS, 400 MHz) δ 6.92-8.65 (m, Ar-H, 8H), 11.82 (s, OH, 1H). MS (m/z): 239.30 (M+1).
4,4'-(1,3,4-oxadiazole-2,5-diyl) diphenol (8): M.P.:223-225ºC. Yield: 75.15%. FT-IR (KBr, v, cm-1): 3265 (OH), 2975 (C-H), 1598 (C=N), 1555 (C=C), 1063 (C-O-C). 1H-NMR (DMSO-d6, TMS, 400 MHz) δ 6.81-8.33 (m, Ar-H, 8H), 11.67 (s, OH, 1H), 11.95 (s, OH, 1H). MS (m/z): 255.30 (M+1).
The final synthesized compounds were characterized by spectral analysis (FT-IR,1H NMR, and LC-MS/MS). A good yield ranging from 75-82% was obtained from the synthesized derivatives. The IR spectrum of compound 8 showed an absorption band at 2975 cm-1 due to aromatic C-H stretch. The absorption bands at 1598 and 1555 cm−1 were attributed due to the C=N stretching and C=C stretching, respectively. The presence of OH group was observed at 3265 cm-1. The absence of carbonyl band supported the formation of 1,3,4-oxadiazole system. The 1H-NMR spectrum of compound 8 showed the aromatic protons resonated as multiplets at δ 6.81-8.33. The presence of OH group was observed at δ 11.67 and 11.95 respectively integrating for one proton. Further evidence for the formation of 1,3,4-oxadiazoles (8) was obtained by recording its mass spectrum. The mass spectrum of the compound (8) showed a molecular ion peak at m/z 255.30 (M+1) in conformity with the molecular formula C14H10N2O3.
In silico analysis
All the newly synthesized compounds possessed desired physicochemical properties with no violations from the normal ranges and are tabulated in Table I. For all design derivatives, the number of rotatable bonds is less than 10. The tPSA values of all the compounds are within the limit indicating the cell permeability. The synthesized compounds obey Lipinski’s rule of five (Table II). The compounds possess hydrogen bond donors ranging from 1(1) – 2(8) and hydrogen bond acceptors ranging from 2.250 (1) – 4.250 (5). The affinity of these derivatives (1-8) with GSK-3β receptor (PDB ID: 3GB2) was expressed in the G score.
Table II: Lipinski’s rules and dock scores at active site of 3GB2 (1-8)
Compound | Molecular weight |
Log P | Donor HB | Acceptor HB | Dock score |
---|---|---|---|---|---|
1 | 272.7 | 4.55 | 1 | 2.25 | -7.132 |
2 | 268.3 | 2.75 | 1 | 4 | -6.56 |
3 | 254.3 | 3.43 | 2 | 4 | -7.524 |
4 | 283.2 | 3.21 | 1 | 4.25 | -6.529 |
5 | 281.3 | 3.16 | 1 | 4.25 | -6.963 |
6 | 272.7 | 3.44 | 1 | 3.25 | -6.465 |
7 | 238.3 | 2.88 | 1 | 3.25 | -6.398 |
8 | 254.3 | 2.49 | 2 | 4 | -7.8 |
Standard (Imipramine) |
280.4 | 4.32 | 0 | 4 | -9.08 |
The synthesized derivatives were found to have binding free energy in the range of -7.800 to -6.398 kcal/mol. The active residues in 3GB2 are ILE 62, GLY 63, ASN 64, GLY 65, SER 66, PHE 67, LYS 65, ASP 200, GLY 202, ASP 181, LYS 183, GLN 185, ASN 186, THR 138, TYR 140, ARG 141, ARG 144, VAL 61,VAL 70, LYS 85. Compound 8 displays the highest affinity and binding energy of -7.800 in comparison to the other synthesized derivatives. Compound 8 and 3 fit into the binding cleft of 3GB2 receptor with the binding energy of -7.800 kcal/mol and -7.524 kcal/mol, respectively. The hydrogen bond interactions are formed with ASP 200, LYS 183 and ARG 144 (Figure 1) in the case of 8 and ILE 62, and ASP 200 in the case of 3. The hydrophobic interaction between the ligand and the receptor also represents good interaction.
Figure 1: 2D interaction of compound 8 with 3GB2
The ADME studies of the synthesized compounds helped to conclude that all the compounds have good BBB penetration as that of the standard (data not shown). QPlogKp score indicates that all the test compounds and the standard are having skin permeability. QPlogKhsa score depicted all the compounds bound to the human serum albumin.
The 1,3,4-oxadiazole derivatives displayed moderate activity with bioactivity scores ranging from -0.01 to -0.05 against the properties of kinase and enzyme inhibition. The kinase inhibition of the derivatives indicates their significance towards antidepressant activity (data not shown).
LAZAR program was used to predict the toxicity of the synthesized compounds (data not shown). The compounds 4, 5, and 7 were liable to cause mutagenicity. Whereas compounds 4, 5, and 8 were liable to cause carcinogenicity, the remaining synthesized compounds were not found to be liable to cause any type of mutagenicity or carcinogenicity. All the synthesized compounds were not susceptible to cause acute toxicity and hence can be termed as safe.
In vivo antidepressant activity
Forced swimming test results revealed that the compound 8 [4,4'-(1,3,4-oxadiazole-2,5-diyl) diphenol], 3 [3-(5-(4-hydroxyphenyl)-1,3,4-oxadiazol-2-yl) phenol] showed moderate activity when compared to the standard. The compound 1 [4-(5-(4-chlorophenyl)-1,3,4-oxadiazol-2-yl) phenol] also showed moderate antidepressant activity (Table III). Whereas, tail suspension test results revealed that compounds 8 and 1 showed moderate activity when compared to the standard. Compound 3 showed moderate antidepressant activity.
Table III: Antidepressant activity
Compound | Forced swimming test | Tail suspension test | ||
---|---|---|---|---|
Duration of immobility (s) | %Change in immobility | Duration of immobility (s) | %Change in immobility | |
1 | 150.2 ± 2.4b | -30 | 147.0 ± 12.0b | -36.6 |
2 | 183.6 ± 1.7b | -14.5 | 201.4 ± 5.8 | -13.2 |
3 | 98.2 ± 2.2b | -54.3 | 148.4 ± 14.5b | -36 |
5 | 175.2 ± 1.9b | -18.4 | 178.0 ± 7.2a | -23.3 |
8 | 88.2 ± 1.9b | -52.9 | 106.0 ± 20.0b | -54.3 |
Control | 214.8 ± 12.9 | --- | 232.0 ± 0.9 | --- |
Standard (Imipramine) | 75.4 ± 1.1 | -64.9 | 71.6 ± 1.4 | -69.2 |
Superscript "a" means p value <0.01; "b" means p value <0.0001 |
Discussion
Among the synthesized 1,3,4-oxadiazole derivatives (1-8), five compounds (1, 2, 3, 5 and 8) were selected on the basis of the highest binding affinity. All these selected compounds a very high binding affinity to the selected GSK-3β receptor (PDBID: 3GB2). All the new compounds have significant interactions with the GSK-3β protein target. These five compounds screened for in vivo antidepressant activity by using the forced swimming test and tail suspension test models. These methods have been identified as making a situation where behavioral desperation is induced; that is, the animal loses hope of escaping the stressful environment that has been shown to play a role in major depression.
Imipramine and the tested compounds significantly reduced mice immobility time. Compounds 8, 3, 1 are the most efficient antidepressants, with immobility times that are identical to Imipramine. The molecule with the substitution of hydroxyl group (electron donating group) resulted in the strongest antidepressant efficacy in both test models (i.e. Compound 8). The recent literature also showed a promising activity against GSK-3β inhibitors (Tantray et al., 2018), and the same compounds were further evaluated as antidepressants. However the compounds were clubbed with 1,3,4-oxadiazole and benzimidazole moiety. Based on the putative role of GSK-3β activity (Tantray et al., 2016,), 1,3,4-oxadiazole-1,2,3-triazole conjugates were further investigated by antidepressants by adopting TST and FST methods. The later compounds were showed promising activity.
Substitution of hydroxyl group at position 4 and due to electron donating capacity showed significant activity, i.e., compound 8. Compounds with substitutions with electron withdrawing groups (chloro) at positions 4 showed moderate activity, i.e., compound 1. Compounds with substitutions with electron donating groups (hydroxy) at positions 3 showed moderate activity, i.e., compound 3. Compounds with substitutions with electron donating groups (methoxy) at position 4 showed weak activity, i.e., compound 2.
Imipramine have been shown in numerous investigations to increase serotonin activity by decreasing biogenic amine reuptake in the CNS. In the present work, compound 8 and 3 showed highest affinity and binding energy with the GSK-3β target protein, resulting in highest antidepressants activity. The positive findings of this study imply that 1,3,4-oxadiazole derivatives synthesized as GSK-3β inhibitors could be employed as a therapeutic tool to analyze GSK-3β clinical implications and design viable therapeutics to treat these diseases.
Conclusion
A new series of 1,3,4-oxadiazole derivatives were synthesized and evaluated for antidepressant activity. Some of the synthesized compounds (8) also showed significant in vivo antidepressant-like activity.
Ethical Issue
The study has been certified by the Ethical Committee for Animal Science (certificate No: NGSMIPS/IAEC/120). The treatment and handling of the animals were carried out in compliance with the guidelines defined by the Committee for the Management and Supervision of Animal Experiments.
References
Aboraia AS, Abdel-Rahman HM, Mahfouz NM, El-Gendy MA. Novel 5-(2-hydroxyphenyl)-3-substituted-2, 3-dihydro-1, 3, 4-oxadiazole-2-thione derivatives: Promising anticancer agents. Bioorg Med Chem. 2006; 14: 1236-46.
Arshad M. 1, 3, 4-oxadiazole nucleus with versatile pharmacological applications: A review. Int J Pharm Sci Res. 2014; 5: 1124-37.
Aslam M. Tail suspension test to evaluate the antidepressant activity of experimental drugs. Bangladesh J Pharmacol. 2016; 11: 292-94.
Beurel E, Grieco SF, Jope RS. Glycogen synthase kinase-3 (GSK3): Regulation, actions, and diseases. Pharmacol Ther. 2015; 148: 114-31.
Bhandari SV, Bothara KG, Raut MK, Patil AA, Sarkate AP, Mokale VJ. Design, synthesis and evaluation of anti-inflammatory, analgesic and ulcerogenicity studies of novel S-substituted phenacyl-1, 3, 4-oxadiazole-2-thiol and Schiff bases of diclofenac acid as nonulcerogenic derivatives. Bioorg Med Chem. 2008; 16: 1822-31.
Can A, Dao DT, Arad M, Terrillion CE, Piantadosi SC, Gould TD. The mouse forced swim test. JoVE. 2012; 29: e3638.
Casey DE. Metabolic issues and cardiovascular disease in patients with psychiatric disorders. Am J Med (Suppl). 2005; 118: 15-22.
Chemicals DO. OECD Guideline for testing of chemicals. The Organization for Economic Co-operation and Development. Paris, 2005, pp 1-3.
Chen CJ, Song BA, Yang S, Xu GF, Bhadury PS, Jin LH, Hu DY, Li QZ, Liu F, Xue W, Lu P. Synthesis and antifungal activities of 5-(3, 4, 5-trimethoxyphenyl)-2-sulfonyl-1, 3, 4-thiadiazole and 5-(3, 4, 5-trimethoxyphenyl)-2-sulfonyl-1, 3, 4-oxadiazole derivatives. Bio-org Med Chem. 2007; 15: 3981-89.
Desai NC, Kotadiya GM, Trivedi AR, Khedkar VM, Jha PC. Design, synthesis, and biological evaluation of novel fluorinated pyrazole encompassing pyridyl 1, 3, 4-oxadiazole motifs. Med Chem Res. 2016; 25: 2698-17.
Duda P, Hajka D, Wójcicka O, Rakus D, Gizak A. GSK3β: A Master player in depressive disorder pathogenesis and treatment responsiveness. Cells 2020; 9: 727-40.
Haq IU, Aslam M, Ahmed H, Sultana N. Antidepressant-like activity of hydroalcoholic extract of Agaricus blazei in stressed and unstressed mice. Bangladesh J Pharmacol. 2019; 14: 136-43.
Karege F, Perroud N, Burkhardt S, Fernandez R, Ballmann E, La Harpe R, Malafosse A. Protein levels of beta-catenin and activation state of glycogen synthase kinase-3beta in major depression: A study with post-mortem prefrontal cortex. J Affect Disord. 2012; 136: 185-88
Kramer T, Schmidt B, Lo Monte F. Small-molecule inhibitors of GSK-3: Structural insights and their application to Alzhei-mer's disease models. Int J Alzheimer’s Dis. 2012; 2012.
Li X, Zhu W, Roh MS, Friedman AB, Rosborough K, Jope RS. In vivo regulation of glycogen synthase kinase-3 β (GSK3 β) by serotonergic activity in mouse brain. Neuropsychopharmacology 2004; 29: 1426-31.
Lou JS, Reeves A, Benice T, Sexton G. Fatigue, and depression are associated with poor quality of life in ALS. Neurology 2003; 60: 122-33.
Macaev F, Ribkovskaia Z, Pogrebnoi S, Boldescu V, Rusu G, Shvets N, Dimoglo A, Geronikaki A, Reynolds R. The structure–antituberculosis activity relationships study in a series of 5-aryl-2-thio-1, 3, 4-oxadiazole derivatives. Bioorg Med Chem. 2011; 19: 6792-807.
Maslat AO, Abussaud M, Tashtoush H, AL-Talib M. Synthesis, antibacterial, antifungal and genotoxic activity of bis-1, 3, 4-oxadiazole derivatives. Pol J Pharmacol. 2002; 54: 55-60.
McGuffin P, Katz R. The genetics of depression and manic-depressive disorder. The Bri J Psy. 1989; 155: 294-304.
Murray CJ, Lopez AD. Alternative projections of mortality and disability by cause 1990–2020: Global burden of disease study. Lancet 1997; 349: 1498-504.
Omar AM, Aboulwafa OM. Synthesis and anticonvulsant properties of a novel series of 2â€substituted aminoâ€5â€arylâ€1, 3, 4â€oxadiazole derivatives. J Het Chem. 1984; 21: 1415-18.
Omar FA, Mahfouz NM, Rahman MA. Design, synthesis and anti-inflammatory activity of some 1, 3, 4-oxadiazole deriva-tives. Eur J Med Chem. 1996; 31: 819-25.
Otte C, Gold SM, Penninx BW, Pariante CM, Etkin A, Fava M, Mohr DC, Schatzberg AF. Major depressive disorder. Nat Rev Dis Primers. 2016; 2:1-20.
Patel KD, Prajapati SM, Panchal SN, Patel HD. Review of synthesis of 1, 3, 4-oxadiazole derivatives. Syn Commun. 2014; 44: 1859-75.
Predictable SE. Side effects of antidepressants: An overview. Clevel Clin J Med. 2006; 73:351-53.
Puthiyapurayil P, Poojary B, Chikkanna C, Buridipad SK. Design, synthesis and biological evaluation of a novel series of 1, 3, 4-oxadiazole bearing N-methyl-4-(trifluoromethyl) phenyl pyrazole moiety as cytotoxic agents. Eur J Med Chem. 2012; 53:203-10.
Roy PP, Bajaj S, Maity TK, Singh J. Synthesis and evaluation of anticancer activity of 1, 3, 4-oxadiazole derivatives against ehrlich ascites carcinoma bearing mice and their correlation with histopathology of liver receptor. Indian J Pharm Edu Res. 2017; 51: 260-69.
Saitoh M, Kunitomo J, Kimura E, Iwashita H, Uno Y, Onishi T, Uchiyama N, Kawamoto T, Tanaka T, Mol CD, Dougan DR. 2-{3-[4-(Alkylsulfinyl) phenyl]-1-benzofuran-5-yl}-5-methyl-1, 3, 4-oxadiazole derivatives as novel inhibitors of glycogen synthase kinase-3β with good brain permeability. J Med Chem. 2009; 52: 6270-86.
Steru L, Chermat R, Thierry B, Simon P. The tail suspension test: A new method for screening antidepressants in mice. Psychopharmacology 1985; 85: 367-70.
Tamminga CA, Nemeroff CB, Blakely RD, Brady L, Carter CS, Davis KL, Dingledine R, Gorman JM, Grigoriadis DE, Henderson DC, Innis RB. Developing novel treatments for mood disorders: Accelerating discovery. Bio Psy. 2002; 52: 589-609.
Tantray MA, Khan I, Hamid H, Alam MS, Dhulap A, Kalam A. Synthesis of benzimidazole-linked-1, 3, 4-oxadiazole carboxamides as GSK-3β inhibitors with in vivo antidepressant activity. Bioorg Chem. 2018; 77: 393-401.
Tantray MA, Khan I, Hamid H, Alam MS, Dhulap A, Kalam A. Synthesis of benzimidazole-based 1,3,4-oxadiazole-1,2,3-triazole conjugates as glycogen synthase kinase-3β inhibitors with antidepressant activity in in vivo models. RSC Adv. 2016; 6: 43345-55.
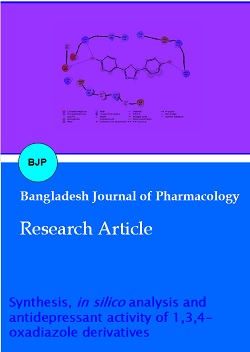