Effect of thymoquinone on cyclophosphamide-induced oxidative and inflammatory ovarian damage in rat
Abstract
This study intends to investigate the effect of thymoquinone against possible ovarian damage and infertility caused by cyclophosphamide in female rats. The rats in the experiment (n=36) were separated into three groups; cyclophosphamide administered, thymoquinone plus cyclophosphamide administered and healthy control. At the end of 30 days of administration, 6 rats from each group were killed and the biochemical and histopathological examinations were performed on the ovarian tissues. The remaining animals were kept in the same cage with mature male rats for two months. Infertility developed in 83% of cyclophosphamide group animals. Thymoquinone attenuated cyclophosphamide-related oxidative and inflammatory ovarian damage and reduced the development of infertility. The outcomes suggest that thymoquinone may be useful in treating infertility due to oxidative and inflammatory ovarian damage.
Introduction
Cyclophosphamide is a nitrogen mustard derivative anti-cancer drug that cross-links to DNA and RNA in the body and inhibits protein synthesis (Mills et al., 2019; Korkmaz et al., 2007). It is used to cure malignant lymphoma, multiple myeloma, breast cancer, small cell lung cancer, rheumatoid arthritis, and systemic lupus erythematosus. Cyclophosphamide remains one of the successful and widely used antineoplastic drugs (Zhang et al., 2021). Other FDA-approved indications of cyclophosphamide include its use in treating neuroblastomas, retinoblastoma, and ovarian adenocarcinoma (Emadi et al., 2009). Besides being an anti-cancer drug, cyclophosphamide has a broad spectrum of toxicity (Fraiser et al., 1991). It is toxic to the brain, liver, and kidney (Zhai et al., 2018). It is pulmonotoxic (Malik et al., 1996).
Chemotherapy also reduces the count of primordial and other follicles that lead to premature menopause and infertility in female patients (Anderson et al., 2006). The disruption of DNA synthesis and cell death by cyclophosphamide has been associated with acrolein and phosphoramide metabolites (Fleer et al., 1982).
These metabolites produce reactive oxygen species (ROS) and cause depletion of the ovarian antioxidant defense system (Tsai-Turton et al., 2007). This event causes an increase in lipid peroxidation (LPO) and malondialdehyde (MDA), a product of LPO, in the ovaries (Saleh and Mansour, 2016). Cyclophosphamide has been reported to cause oxidative damage and subsequent inflammation in the ovaries (Yener et al., 2013). The role of endogenous oxidants and antioxidants such as MDA, nitric oxide, myeloperoxidase (MPO), catalase (CAT), glutathione (GSH), glutathione peroxidase (GPX), and superoxide dismutase (SOD) has been shown in the pathogenesis of cyclophosphamide-associated ovarian damage. Also, it has been stated that proinflammatory cytokines such as tumor necrosis factor-α (TNF-α), interleukin-1β (IL-1β), and interleukin-6 (IL-6) are major components (Liu et al., 2021). This literature knowledges suggest that anti-inflammatory and antioxidant drugs may be useful in treating ovarian damage and infertility due to cyclophosphamide treatment.
Thymoquinone, whose effect will be tested on cyclophosphamide-related ovarian damage and infertility, is an active phenolic compound obtained from the essential oil of Nigella sativa seed (Guzelsoy et al., 2018). Thymoquinone exhibits anti-inflammatory and antioxidant activity by inhibiting the excessive production of LPO and pro-inflammatory cytokines such as TNF-α, IL-1β, and IL-6 (Ali et al., 2021). It has been informed that thymoquinone has antioxidant and anti-inflammatory impacts as well as immunomodulatory properties (Butt et al., 2021). All this information indicates that thymoquinone may be useful in treating cyclophosphamide-related ovarian damage and infertility. There is no know report about the effects of thymoquinone on cyclophosphamide-induced ovarian damage and infertility in animals. This study aims to investigate the impact of thymoquinone against possible ovarian damage and infertility of cyclophosphamide in female rats and examine the ovarian tissues biochemically and histopathologically.
Materials and Methods
Animals
In this study, a total of 36 albino Wistar-type female rats weighing 225-237 g of 3.5-4 months were obtained from Erzincan Binali Yildirim University Experiment Animals Application and Research Center. Animals were housed and fed in the laboratory at normal room temperature (22°C) for one week before experimentation, to allow them to adapt to the environment.
Chemicals
Cyclophosphamide used in the experiment was obtained from EIP Eczacıbaşı (Turkey), Thymoquinone Sigma-Aldrich (USA), and thiopental sodium from İ.E ULAGAY (Turkey).
Experimental groups
The rats which would be used in the experiment (n=36) were separated into three groups cyclophosphamide administered (CYC), thymoquinone + cyclophosphamide administered (TCYC), and healthy control (HG).
Experimental procedure
For the implementation of this experiment, the TCYC (n=12) group was given thymoquinone at a dose of 20 mg/kg orally into the stomach. Olive oil as solvent was administered orally in the same volume (0.2 mL) to CYC (n=12) and HG (n=12) groups. One hour after thymoquinone and olive oil were administered, cyclophosphamide was injected intraperitoneally at a dose of 75 mg/kg to the TCYC and CYC groups. This procedure was repeated once a day for 30 days. At the end of this period, six (n=6) rats from each group were euthanized with a high dose (50 mg/kg) of thiopental sodium anesthesia. MDA, total glutathione (tGSH), TNF-α, IL-1β and IL-6 levels were measured in the removed ovarian tissues biochemically and histopathological evaluation was made. The remaining animals stayed for breeding (6 female rats from each group) for two months in normal laboratory conditions in the same cage with mature male rats. Rats that did not become pregnant and did not give birth within this period were considered infertile. All experimental results were evaluated by making comparisons between groups.
Biochemical analyses
Preparation of samples
Before dissection, all tissues were rinsed with phosphate-buffered saline solution. The tissues were homogenized in ice-cold phosphate buffers (50 mM, pH 7,4) that were appropriate for the variable to be measured. The tissue homogenates were centrifuged at 5,000 x g for 20 min at 4°C, and the supernatants were extracted to analyze GSH and MDA. All tissue results were expressed by dividing into gram protein. All spectrophotometric measurements were performed via a microplate reader (Bio-Tek, USA).
MDA and tGSH analysis
MDA (µmol/g protein) measurement was based on the method using spectrophotometric measurement of the absorbance of the pink-colored complex formed by thiobarbituric acid (TBA) and MDA (Ohkawa et al., 1979). tGSH (nmol/g protein) measurement was made according to the method described elsewhere (Sedlak et al., 1968).
TNF-α, IL-1β and IL-6 analysis
The weights of the samples were measured. Then, we cut all of the tissues, rapidly frozen with liquid nitrogen and homogenized by pestle and mortar; maintained samples at 2-8°C after melting. PBS (pH 7.4), 1/10 (w/v) was added. After that, vortex for 10 sec and centrifuged 20 min at 10,000 x g were made. The supernatants were collected carefully. The levels of TNF-α (ng/L), IL-1β(pg/L), and IL-6 (ng/L) were measured using according to a commercial kit supplied by Eastbiopharm Co Ltd., ELISA kit, China.
Box I: Histopathological Procedure
Histopathology is the study of pathological tissues using a light microscope.
Requirements
Formalin (10%); Light microscope; Microtome; Olympus BX53 (Japan); Ovary; Paraffin
Procedure
Step 1: The ovary was fixed in a formalin solution for histopathological evaluation.
Step 2: It was embedded in paraffin after going through routine histological tissue follow-up stages.
Step 3: Sections of 5 µm thickness were taken on the microtome. Sections taken were stained with hematoxylin-eosin and examined with a light microscope. For histopathological evaluation, random sampling was made for each animal in the groups, and evaluation was made in an average of 12-15 areas. The findings were evaluated semi-quantitatively. The histopathological damage severity in each ovarian tissue section was scored between grades 0-3 (0- normal, 1- mild injury, 2-moderate injury, and 3- severe injury).
Reference (video)
Rajasekaran, 2019
Statistical analysis
IBM SPSS 22 (IBM Corp. Released 2013. Version 22.0) was used for all statistical analysis. Biochemical results were presented as mean ± standard error of the mean (SEM), and histopathological results were presented as median (minimum-maximum). One-way ANOVA was used for group comparisons for biochemical data, and the Tukey HSD was used as post hoc. Kruskal-Wallis test was used in the evaluation of histopathological data, and the Dunn test was used as post hoc. The statistical level of significance for all tests was considered to be p<0.05.
Results
Biochemical findings
MDA and tGSH analysis results
As seen in Table I, MDA levels increased and tGSH levels decreased when cyclophosphamide group was compared with healthy and cyclophosphamide plus thymoquinone (p<0.001). When the thymoquinone group was compared with the healthy group, no statistically significant difference was found between them in terms of MDA and tGSH levels (p>0.05).
TNF-α, IL-1β, and IL-6 analysis results
The levels of proinflammatory cytokines such as TNF-α, IL-1β, and IL-6 in the ovarian tissue of rats in the cyclophosphamide group were higher than those in the healthy and cyclophosphamide plus thymoquinone groups (p<0.001; Table I). Healthy and cyclophosphamide plus thymoquinone groups were close to each other in terms of TNF-α and IL-1β values (p>0.05).
Table I: Biochemical findings
Target | Groups | Groups | Groups | Pairwise comparison | Pairwise comparison | Pairwise comparison |
---|---|---|---|---|---|---|
Target | Healthy (HG) | Cyclophosphamide (CYC) | Thymoquinone + cyclophosphamide (TCYC) | HG vs. CYC | HG vs. TCYC | CYC vs. TCYC |
Malondialdehyde | 2.8 ± 0.0 | 6.2 ± 0.2 | 3.2 ± 0.1 | <0.001 | 0.031 | <0.001 |
Total glutathione | 5.8 ± 0.0 | 2.2 ± 0.0 | 4.7 ± 0.1 | <0.001 | <0.001 | <0.001 |
Tumor necrosis factor alfa | 3.2 ± 0.0 | 6.1 ± 0.1 | 3.4 ± 0.0 | <0.001 | 0.161 | <0.001 |
Interleukin 1-beta | 3.1 ± 0.2 | 6.2 ± 0.1 | 3.4 ± 0.1 | <0.001 | 0.218 | <0.001 |
Interleukin 6 | 2.2 ± 0.0 | 4.2 ± 0.1 | 2.4 ± 0.1 | <0.001 | 0.021 | <0.001 |
Results are presented as mean ± standard error of the mean (SEM). As post-hoc test Tukey HSD was performed after ANOVA; n=6 |
Reproduction test results
As seen in Table II, all six animals in the healthy group gave birth within two months. In the cyclophosphamide administered group, one of the six breeding animals gave birth, but the remaining five female rats did not give birth within two months. Three out of six animals gave birth in the thymoquinone-administered group, and the other three were considered infertile.
Table II: Reproductive findings
Group | Fertile | Non-fertile |
---|---|---|
Healthy (n=6) | 6 | - |
Cyclophosphamide (n=6) | 1 | 5 |
Thymoquinone + cyclophosphamide (n=6) | 3 | 3 |
Histopathological findings
As seen in Figure 1A, no histopathological findings were found in the follicle structures and corpus luteum in the ovaries of the healthy animal group. However, diffuse and severely congested blood vessels, hemorrhage, and edema were observed in the ovarian tissue of the cyclophosphamide group administered with cyclophosphamide alone (Figure 1B). Again, polymorphonuclear leukocytes infiltration and significant degeneration in the follicles were observed in the ovarian tissue of the cyclophosphamide group (Figure 1C). As seen in Figure 3D, mildly congested vascular structures and mild follicle degeneration were observed in the ovarian tissue of the TCYC group treated with cyclophosphamide plus thymoquinone (Table III).
Table III: Histopathological damage of ovarian tissue
Group | Vascular congestion | Hemorrhage | Edema | Follicle degeneration | PMNL infiltration | |
---|---|---|---|---|---|---|
Healthy (n=6) | 0 | 0 | 0 | 0 | 0 | |
Cyclophosphamide (n=6) | 3a | 3a | 3a | 3a | 3a | |
Thymoquinone + cyclophosphamide (n=6) | 2b | 0b | 0b | 1b | 0b | |
Histopatological grading; 0-normal, 1- mild injury, 2-moderate injury, and 3-severe injury. ap<0.001, according to healthy group; bp>0.05, according to healthy group; Kruskal Wallis test was used and Dunn test was performed as post hoc. Results are presented as median (minimum-maximum); PMNL: polymorphonuclear leukocytes |
Figure 1: Histopathological evaluation of ovarian tissue in experimental groups. Ovarian tissue of the normal follicle structures (arrow) and corpus luteum (asterisk), H&E x40 (A). Cyclophosphamide administered cyclophosphamide group ovarian tissue; diffuse congestion (asterisk), hemorrhage (arrow) and edema (zigzag arrow), H&E x100 (B). Cyclophosphamide administered cyclophosphamide group ovarian tissue; degenerate follicle structures (arrow), polymorphonuclear leukocyte infiltration (square), H&E x100 (C). Ovarian tissue of cyclophosphamide plus thymoquinone group; vascular structures with congestion (asterisk), follicle degeneration (arrows), H&E x100 (D)
Discussion
In this study, the impact of thymoquinone against cyclophosphamide-induced ovarian harm and infertility in female rats was researched. Tissues were examined biochemically and histopathologically to evaluate ovarian damage. Oxidant and antioxidant parameters are used to determine oxidative stress in tissues (Salman et al., 2011). In damaged tissues, the oxidant/antioxidant balance changes in favor of oxidants, and an increment in oxidant values and a reduction in antioxidant values are monitored (Kisaoglu et al., 2013). The biochemical results, which are compatible with this literature, revealed that the amount of MDA in the cyclophosphamide group was higher than in the healthy and thymoquinone groups. The high amount of MDA in the tissues indicates that the LPO reaction is increased (Capuzzi et al., 2022). MDA produced at the end of the LPO reaction is a cytotoxic oxidant parameter (Saleh and Mansour, 2016). For this reason, MDA may cause exacerbation of LPO damage and further destruction (Suleyman and Ozcicek, 2019). In the studies conducted previously, it was observed that antioxidant levels decreased in ovarian tissue where MDA was increased (Turkler et al., 2020). Another study remarked that cyclophosphamide caused an increment in MDA levels and a reduction in antioxidants and had a toxic effect on the ovaries (Saleh and Mansour, 2016).
In this study, the level of tGSH in the ovarian tissue of rats administered with cyclophosphamide was found to be lower than that in the healthy and thymoquinone-treated groups. GSH is an endogenous antioxidant molecule (Singh, 2002). ROS produced as part of metabolic processes are neutralized by endogenous GSH and other enzymatic and non-enzymatic antioxidant defense systems (Urso and Clarkson, 2003). GSH scavenges cytotoxic hydrogen peroxide; reacts non-enzymetically with other ROSs such as singlet oxygen, superoxide radical and hydroxyl radical, and converts them into non-toxic products (Blokhina et al., 2003). However, if antioxidants are insufficient to neutralize oxidants, the balance between oxidants/antioxidants is disturbed in favor of oxidants (Clarkson and Thompson, 2000). Based on experimental results and literature, it can be said that the oxidant/antioxidant balance in the cyclophosphamide group is maintained by the superiority of oxidants. Existing studies showing the reduction of GSH stores in ovarian tissue with cyclophosphamide support the tGSH results (Saleh et al., 2016).
The literature has reported that cyclophosphamide causes oxidative damage and subsequent inflammation in the ovaries (Yener et al., 2013). Is also consistent with the present results. In the cyclophosphamide group, which we applied to the animals for a month, the levels of inflammation markers such as TNF-α, IL-1β, and IL-6 increased along with oxidant parameters. It was determined that cytokines such as TNF-α, IL-1β and IL-6 raised in parallel with the increment in oxidants and the reduction in antioxidants (Liu et al., 2021). The present results and literature suggest that there is a connection between proinflammatory cytokine production and oxidant production. It is known that ROSs induce proinflammatory cytokine production (Ha et al., 2002). In recent studies, it has been reported that TNF-α, IL-1β, and IL-6 levels are also increased in ovarian tissue where ROS product is measured high.
In this study, it was understood that thymoquinone prevented the balance between oxidant/antioxidant in the ovarian tissue from changing in favor of oxidants with cyclophosphamide. There are no data about the effect of thymoquinone on cyclophosphamide-induced ovarian harm in the literature. On the other hand, thymoquinone's antioxidant, anti-inflammatory, immunomodulatory, antihistaminic, antimicrobial, and anti-tumor effects are known (Darakhshan et al., 2015). Also, it has been documented that thymoquinone protects heart tissue from oxidative stress by antagonizing the effects of cyclophosphamide on MDA and GSH (Nagi et al., 2011). It has been experimentally shown that thymoquinone suppresses cyclophosphamide-induced LPO, blood sugar, liver enzyme activities, bilirubin, urea, creatinine, triglyceride, cholesterol and low-density lipoprotein increase (Alenzi et al., 2010). Another study shows that thymoquinone protects ovarian tissue from ischemia-reperfusion injury with its antioxidant activity (Mete Ural et al., 2016). As mentioned above, it is thought that there is a connection between oxidant-antioxidant and proinflammatory cytokine production (Liu et al., 2021; Ha et al., 2002). This literature information confirms that thymoquinone inhibited cyclophosphamide-related MDA, TNF-α, IL-1β, and IL-6 increase and tGSH decrease in ovarian tissue in this study. Recent research has documented that thymoquinone exhibits anti-inflammatory and antioxidant activity by inhibiting the excessive production of LPO and pro-inflammatory cytokines such as TNF-α, IL-1β, and IL-6 (Ali et al., 2021).
The present study observed that the biochemical results get from all animal groups were consistent with the histopathological results. Severely congested blood vessels, hemorrhage, and edema were observed in the ovarian tissue of the cyclophosphamide group, in which oxidant and pro-inflammatory cytokines were measured high. There is no histopathological findings were observed in the ovary of the thymoquinone-treated group, except for mildly congested vascular structures and mild follicle degeneration. Previous studies showed histopathologically that vascular congestion, edema, and follicle degeneration developed in the cyclophosphamide group, whose levels of oxidative stress biomarkers such as MDA and ROS increased significantly. Moreover, it was emphasized that cyclophosphamide-related ovarian damage was alleviated by antioxidant therapy (Hamzeh et al., 2018). The fact that thymoquinone reduced cyclophosphamide-related histopathological damage in the ovarian tissue may be due to its antioxidant, anti-inflammatory and immunomodulatory effects (Darakhshan et al., 2015). The hemorrhage in the ovarian tissue of rats administered cyclophosphamide, congestion in the stroma, and significant mononuclear cell infiltration are caused by oxidative stress (Melekoglu et al., 2018).
In this study, the biochemical and histopathological findings of the present study are compatible with the reproductive test results. Infertility developed in 83% of the animals in the group in which cyclophosphamide, which causes an increase in oxidant and proinflammatory biomarkers, was administered. The infertility rate was 50% in the animal group administered with cyclophosphamide + thymoquinone. These results indicate that cyclophosphamide causes reproductive dysfunction. In a study conducted previously, it was stated that the oxidative stress caused by cyclophosphamide in the rat ovary might cause infertility and can be treated with antioxidant drugs (Khedr, 2015). It was argued that cyclophosphamide-induced infertility was given rise to by oxidative stress and inflammation in the ovaries; Besides, antioxidant and anti-inflammatory therapy has been reported to be beneficial (Saleh and Mansour, 2016). Thymoquinone has an antioxidant and anti-inflammatory effect on ovarian tissue. The information that thymoquinone inhibits the overproduction of LPO and TNF-α, IL-1β, IL-6 promote the present experimental results (Ali et al., 2021).
Conclusion
Thymoquinone may be useful in treating cyclophosphamide-induced infertility due to oxidative and inflammatory ovarian damage.
Ethical Issue
The protocols and procedures were approved by the local Animal Experimentation Ethics Committee (Date: 25.11.2021; meeting No.: 4/18).
References
Alenzi FQ , El-Bolkiny Yel S, Salem ML. Protective effects of Nigella sativa oil and thymoquinone against toxicity induced by the anti-cancer drug cyclophosphamide. Br J Biomed Sci. 2010; 67: 20-28.
Ali MY, Akter Z, Mei Z, Zheng M, Tania M, Khan MA. Thymoquinone in autoimmune diseases: Therapeutic potential and molecular mechanisms. Biomed Pharmacother. 2021; 134: 111157.
Anderson RA, Themmen AP, Al-Qahtani A, Groome NP, Cameron DA. The effects of chemotherapy and long-term gonadotrophin suppression on the ovarian reserve in pre-menopausal women with breast cancer. Hum Reprod. 2006; 21: 2583-92.
Blokhina O, Virolainen E, Fagerstedt KV. Antioxidants, oxidative damage and oxygen deprivation stress: A review. Ann Bot. 2003; 91: 179-94.
Butt MS, Imran M, Imran A, Arshad MS, Saeed F, Gondal TA, Shariati MA, Gilani SA, Tufail T, Ahmad I, Rind NA. Therapeutic perspective of thymoquinone: A mechanistic treatise. Food Sci Nutr. 2021; 9: 1792-809.
Capuzzi E, Ossola P, Caldiroli A, Auxilia AM, Buoli M. Malondialdehyde as a candidate biomarker for bipolar disorder: A meta-analysis. Prog Neuropsychopharmacol Biol Psychiatry. 2022; 113: 110469.
Clarkson PM, Thompson HS. Antioxidants: What role do they play in physical activity and health? Am J Clin Nutr. 2000; 72: 637S-46S.
Darakhshan S, Bidmeshki Pour A, Hosseinzadeh Colagar A, Sisakhtnezhad S. Thymoquinone and its therapeutic potentials. Pharmacol Res. 2015; 95-96: 138-58.
Emadi A, Jones RJ, Brodsky RA. Cyclophosphamide and cancer: Golden anniversary. Nat Rev Clin Oncol. 2009; 6: 638-47.
Fraiser LH, Kanekal S, Kehrer JP. Cyclophosphamide toxicity. Characterising and avoiding the problem. Drugs 1991; 42: 781-95.
Fleer R, Brendel M. Toxicity, interstrand cross-links and DNA fragmentation induced by activated cyclophosphamide in yeast: Comparative studies on 4-hydroperoxycyclophosphamide, its monofunctional analogon, acrolein, phosphoramide mustard, and nornitrogen mustard. Chem Biol Interact. 1982; 39: 1-15.
Guzelsoy P, Aydin S, Basaran N. Potential effects of thymoquinone the active constituent of black seed (Nigella sativa L.) on human health. J Lit Pharm Sci. 2018; 7: 118-35.
Ha H, Yu MR, Choi YJ, Kitamura M, Lee HB. Role of high glucose-induced nuclear factor-kappaB activation in monocyte chemoattractant protein-1 expression by mesangial cells. J Am Soc Nephrol. 2002; 13: 894-902.
Hamzeh M, Hosseinimehr SJ, Mohammadi HR, Yaghubi Beklar S, Dashti A, Talebpour Amiri F. Atorvastatin attenuates the ovarian damage induced by cyclophosphamide in rat: An experimental study. Int J Reprod Biomed. 2018; 16: 323-34.
Khedr NF. Protective effect of mirtazapine and hesperidin on cyclophosphamide-induced oxidative damage and infertility in rat ovaries. Exp Biol Med (Maywood). 2015; 240: 1682-89.
Kisaoglu A, Borekci B, Yapca OE, Bilen H, Suleyman H. Tissue damage and oxidant/antioxidant balance. Eurasian J Med. 2013; 45: 47-49.
Korkmaz A, Topal T, Oter S. Pathophysiological aspects of cyclophosphamide and ifosfamide induced hemorrhagic cystitis: Implication of reactive oxygen and nitrogen species as well as PARP activation. Cell Biol Toxicol. 2007; 23: 303-12.
Liu J, Wang W, Chen L, Li Y, Zhao S, Liang Y. Chemoprotective effect of syringic acid on cyclophosphamide induced ovarian damage via inflammatory pathway. J Oleo Sci. 2021; 70: 675-83.
Malik SW, Myers JL, DeRemee RA, Specks U. Lung toxicity associated with cyclophosphamide use: Two distinct patt-erns. Am J Respir Crit Care Med. 1996; 154: 1851-56.
Melekoglu R, Ciftci O, Eraslan S, Cetin A, Basak N. Beneficial effects of curcumin and capsaicin on cyclophosphamide-induced premature ovarian failure in a rat model. J Ovarian Res. 2018; 11: 33.
Mete Ural U, Bayoglu Tekin Y, Sehitoglu I, Kalkan Y, Cumhur Cure M. Biochemical, histopathological and immunohistochemical evaluation of the protective and therapeutic effects of thymoquinone against ischemia and ischemia/reperfusion injury in the rat ovary. Gynecol Obstet Invest. 2016; 81: 47-53.
Mills KA, Chess-Williams R, McDermott C. Novel insights into the mechanism of cyclophosphamide-induced bladder toxi-city: Chloroacetaldehyde's contribution to urothelial dysfunction in vitro. Arch Toxicol. 2019; 93: 3291-303.
Nagi MN, Al-Shabanah OA, Hafez MM, Sayed-Ahmed MM. Thymoquinone supplementation attenuates cyclophosphamide-induced cardiotoxicity in rats. J Biochem Mol Toxicol. 2011; 25: 135-42.
Ohkawa H, Ohishi N, Yagi K. Assay for lipid peroxides in animal tissues by thiobarbituric acid reaction. Anal Biochem. 1979; 95: 351-58.
Rajasekaran M. Nephroprotective effect of Costus pictus extract against doxorubicin-induced toxicity on Wistar rat. Bangladesh J Pharmacol. 2019; 14: 93-100.
Saleh DO, Mansour DF. Ovario-protective effects of genistein against cyclophosphamide toxicity in rats: Role of anti-mullerian hormone and oestradiol. Eur J Pharmacol. 2016; 789: 163-71
Salman S, Kumbasar S, Yilmaz M, Kumtepe Y, Borekci B, Bakan E, Suleyman H. Investigation of the effects of the chronic administration of some antihypertensive drugs on enzymatic and non-enzymatic oxidant/antioxidant parameters in rat ovarian tissue. Gynecol Endocrinol. 2011; 27: 895-99.
Sedlak J, Lindsay RH. Estimation of total, protein-bound, and nonprotein sulfhydryl groups in tissue with Ellman's reagent. Anal Biochem. 1968; 25: 192-205.
Singh RJ. Glutathione: A marker and antioxidant for aging. J Lab Clin Med. 2002; 140: 380-81.
Suleyman H, Ozcicek A. Molecular mechanism of ischemia reperfusion injury. Arch Basic Clin Res. 2019; 2: 25-27.
Tsai-Turton M, Luong BT, Tan Y, Luderer U. Cyclophosphamide-induced apoptosis in COV434 human granulosa cells involves oxidative stress and glutathione depletion. Toxicol Sci. 2007; 98: 216-30.
Turkler C, Onat T, Yildirim E, Kaplan S, YAZICI G, Mammadov R. An experimental study on the use of lycopene to prevent infertility due to acute oxidative ovarian damage caused by a single high dose of methotrexate. Adv Clin Exp Med. 2020; 29: 5-11.
Urso ML, Clarkson PM. Oxidative stress, exercise, and antioxidant supplementation. Toxicology 2003; 189: 41-54.
Yener NA, Sinanoglu O, Ilter E, Celik A, Sezgin G, Midi A, Deveci U, Aksungar F. Effects of spirulina on cyclophosphamide-induced ovarian toxicity in rats: Biochemical and histomorphometric evaluation of the ovary. Biochem Res Int. 2013; 2013: 764262.
Zhai J, Zhang F, Gao S, Chen L, Feng G, Yin J, Chen W. Schisandra chinensis extract decreases chloroacetaldehyde production in rats and attenuates cyclophosphamide toxicity in liver, kidney and brain. J Ethnopharmacol. 2018; 210: 223-31.
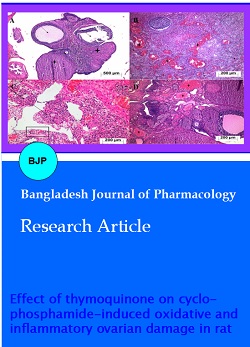