Eckol, a potential inhibitor of aryl hydrocarbon receptor, inhibits proliferation and induces apoptosis in breast cancer cells
Abstract
The present study was designed to explore phlorotannin eckol protein targets and evaluate the anti-cancer effects of eckol against human breast cancer cells. ProTox-II server and protein-ligand docking analysis determined the aryl hydrocarbon receptor (AhR) as a putative target of eckol. The AhR has been determined as a potential target for breast cancer treatment. The effect of eckol treatment on proliferation, apoptosis, and cell cycle activities was detected in MDA-MB-231 and SK-BR-3 breast cancer cell lines which were selected dependent on AhR expression profiles. Consistent with the AhR expression profile, MDA-MB-231 cells were more sensitive to eckol treatment compared to SK-BR-3 cells in proliferation, apoptosis and cell cycle results. Eckol treatment shows a significant antiproliferative and apoptotic response in breast cancer cells. Overall, these results indicated that the action of eckol may be related to the AhR gene regulation in different breast cancer cell lines.
Introduction
Breast cancer is the most common cancer type in female populations and the second cancer-related death world-wide (Bray et al., 2018). Several chemotherapeutic agents and their combinations are used for breast cancer treatments and their cytotoxic roles on breast cancer cell line models were detailed and described (Waks and Winer, 2019). Although molecular characterization and treatment options for breast cancer are well known, it still is the leading cause of cancer-related death in females (Cardoso et al., 2017). Therefore, there is an urgent need to identify and characterize novel molecules associated with cancer malignancy for breast cancer therapy.
Around 60% of drugs in clinical trials for cancer are either natural products from natural sources or their derivatives (Demain and Vaishnav, 2011; Dyshlovoy and Honecker, 2015). New potential natural compounds (e.g., terpenes, steroids, alkaloids, polyketides, etc.) are screened for their therapeutic efficacy (Buyel, 2018; Yao and Bai, 2017) and some of these bioactive agents and some of these bioactive agents have already been isolated from marine organisms (MartÃnez Andra-de et al., 2018). Marine brown algae accumulate phlorotannins which are phloroglucinol-based polyphenols and consist of a monomeric unit of phloroglucinol (1,3,5-trihydroxy benzene) (Rajan et al., 2021). They are biosynthesized by the acetatemalonate pathway, also known as the polyketide pathway, and stored in vesicles called physodes.
Among the different types of bioactive phlorotannins, one of the essential metabolites is eckol (Zhang et al., 2012). The bioactivities of eckol-type phlorotannins can be classified as antioxidant, anti-diabetic, hepatoprotective, neuroprotective, radioprotective, anti-aging, anti-hypertensive, anticoagulative, anti-obesity, antihistaminic, anti-inflammatory, antihyperlipidemic, antityrosinase, anti-cancer, and cytotoxic (Manandhar et al., 2019). The broad variety of these activities put forward eckol as a highly potential therapeutic agent. Eckol is a phlorotannin isolated from a brown alga Ecklonia cava.
Eckol anti-cancer activity has been determined in limited studies, such as human HeLa (cervical), SW1990 (pancreatic), HepG2 (hepatocellular), and MCF7 (breast) cancer cells (Jun et al., 2014; Mwangi et al., 2017; Zhang et al., 2019). A recent study showed that eckol has anti-tumor activity in xenograft-bearing mice model by immunomodulatory activity (Zhang et al., 2019). Studies have shown that eckol is a very effective compound in various human cancer cell models. However, only limited data have been described in the literature explaining the mechanism of eckol related to its anti-cancer activity.
The present study aims to identify possible targets of eckol and the role of the eckol on proliferation, apoptosis, and cell cycle processes in different breast cancer cell lines.
Materials and Methods
Cell culture
The breast cancer cell lines MDA-MB-231 and SK-BR-3 were maintained in high-glucose (4.5 g/L) DMEM media, containing 10% fetal bovine serum (FBS), 1% penicillin-streptomycin, and 1% non-essential amino acids.
Cell proliferation assay and eckol concentration determination
The antiproliferative effects of eckol (Kozak et al., 2020) on MDA-MB-231 and SK-BR-3 cells were determined by the xCELLigence system which measures real-time cell impedance. The special biosensor plates (E-plate) are compatible with the system and the plates measure target cell adhesion and growth (Picot et al., 2017). The control cells were treated with 0.05% final concentration of DMSO same as Eckol-treated cells (Kim et al., 2014) remaining protocol is mentioned in Box I. All experiments were performed at least three times.
Cell cycle and apoptosis analyses
For cell cycle and apoptosis assays, MDA-MB-231 and SK-BR-3 cells (at a density of 5 × 105) were seeded in 25 cm2 flasks and incubated for 24 hours at 37ºC. Cells were treated with eckol at a concentration of 160 and 480 µM. After the treatment, cells were incubated for 2 hours at 37ºC. Cells were then trypsinized and washed in cold PBS. For cell cycle analysis, cells were treated with propidium iodide solution for 1 hour at 37ºC, and the dye was washed and removed by centrifugation after the incubation. Cells were then re-suspended in cold PBS. For the quantification of apoptosis (Rieger et al.,2011), cells were re-suspended at a density of 1 × 106 cells/mL in 1× binding buffer (10 mM HEPES/NaOH, pH 7.4; 140 mM NaCl; 2.5 mM CaCl2). Then, 100 µL of the cellular solution was stained with 5 µL annexin V-APC and 5 µL propidium iodide (BD 550475, BD 51-66211E) for 15 min at room temperature. After staining, 400 µL of 1× binding buffer was added to the cells. Cell cycle and apoptosis were then analyzed with Accuri C6 (Becton Dickinson) flow cytometry.
Retrieval of ligand and docking experiment
The chemical structure of eckol (CID: 145937) was obtained from PubChem Database. Open Babel GUI 2.3.1 was used to generate the 2D structure of eckol.
Auto Dock Vina 1.1.2 was employed as a program for docking experiments (Seeliger and de Groot, 2010). The fixing of the grid box was set using Auto Dock Tools 1.5.7, x, y, z of 80, 80, 80, and x, y, z center as 6.281, 30.257, 216.222 respectively. The docking result was visualized using pymol 2.5 and discovery studio visualizer 4.0 (Pawar and Rohane, 2021).
Box I: Real-time Cell Proliferation Analysis
The system is label-free technology monitors and dynamic monitoring of cell proliferation with gold electrodes embedded proprietary plates. The sensor's bottom of the plate measures a cell-electrode impedance response that indicates cell proliferation. The impedance differences within an electrical circuit are calculated and converted into cell index by software.
Requirements
Cell culture media, RTCA real-time cell analyzer and plate
Procedure
Step 1: Add 100 µL cell media in each well
Step 2: Background reading
Step 3: Cells were plated in 16-well plate at an initial density of 5 × 103 cells, with 50 µL media per well
Step 4: After 24 hours of incubation check the cell proliferation
Step 5: Eight different concentrations of 50 µL eckol (Control, 20, 40, 80, 160, 320, 400, and 480 µM) were added to each well
Step 6: Cell indexes values obtained through the software
Reference
Picot et al. 2017
Transcript and toxicity database analyses
The breast cancer cell lines AhR gene expression data were obtained by expression atlas from EMBL-EBI database. To predict the toxic potential of eckol was identified by the ProTox-II server.
Statistical analyses
Statistical significance was assessed using a student’s t and ANOVA test. A p value of <0.05 was considered statistically significant. All experiments were performed in triplicate.
Results
Eckol target identification and selection of breast cancer cell lines
To investigate the effect of eckol in breast cancer, the possible targets of the eckol were tested by the toxicity chemicals database. In the target analysis result, MMP and AhR were identified as active targets of eckol and the AhR has a high probability rate (0.76) (Table I).
Table I: Target identification of eckol according to protox II database
Target | Prediction | Probability |
---|---|---|
Hepatotoxicity | Inactive | 0.78 |
Carcinogenicity | Inactive | 0.64 |
Immunotoxicity | Inactive | 0.93 |
Mutagenicity | Inactive | 0.55 |
Cytotoxicity | Inactive | 0.91 |
Aryl hydrocarbon receptor (AhR) | Active | 0.76 |
Androgen receptor (AR) | Inactive | 0.97 |
Androgen receptor ligand binding domain (AR-LBD) | Inactive | 0.90 |
Aromatase | Inactive | 0.67 |
Estrogen receptor alpha (ER) | Inactive | 0.50 |
Estrogen receptor ligand binding domain (ER-LBD) | Inactive | 0.62 |
Peroxisome proliferator-activated receptor gamma (PPAR-gamma) | Inactive | 0.96 |
Nuclear factor (erythroid-derived 2)-like 2/antioxidant responsive element (nrf2/ARE) | Inactive | 0.86 |
Heat shock factor response element (HSE) | Inactive | 0.86 |
Mitochondrial membrane potential (MMP) | Active | 0.64 |
Phosphoprotein (tumor suppressor) p53 | Inactive | 0.84 |
ATPase family AAA domain-containing protein 5 (ATAD5) | Inactive | 0.85 |
The toxicity chemicals database results prompted us to perform molecular docking studies to understand the eckol-AhR (ligand-protein) interactions by Autodock Vina 1.1.2 (Seeliger and de Groot, 2010). The accommodation of eckol revealed various amino acid residues engaged in interaction. These interactions were attributed to evidence of the in silico ligand-protein interaction. Eckol showed a prominent interaction with the AhR protein accompanied by an affinity of − 8.5 kcal/mol and one hydrogen bond specifically with MET237 and seven pi-alkyl interactions with ALA267, LEU224, VAL158, and VAL129. The eckol displayed binding in the helix and beta-strands (PAS-A domain with VAL129 and VAL158) of the AhR protein (Figure 1).
Figure 1: Binding residues and interactions of AhR with eckol
After determining that eckol interacted with AhR protein with a high energy level, the breast cancer cell lines were selected according to their AhR gene expression profile. The three breast cancer cell lines, luminal MCF-7, triple-negative MDA-MB-231, and HER2 enrich SK-BR-3, were interesting for expression profile analysis determined by the expression database. AhR expression was the highest in MDA-MB-231, medium in MCF-7, and the lowest in SK-BR-3 detected as17 FKPM, 10 FKPM, and 5FKPM respectively (Figure 2).
Figure 2: AhR gene expression profile in MDA-MB-231, MCF-7, and SK-BR-3 human breast cancer cell lines. AhR expression data were taken from the ebi. ac.UK database. FKPM: Fragments per kilobase of exon model per million reads mapped
Effect of eckol on breast cancer cell lines proliferation
After the selection of the AhR the highest expressed MDA-MB-231 and lowest expressed cell lines SK-BR-3, we tested the role of eckol in cell proliferation. Cells were treated at 0, 20 µM, 40 µM, 80 µM, 160 µM, 400 µM, and 480 µM eckol for 2, 4, 6, and 8 hours. The MDA-MB-231 cell proliferation was significantly inhibited on 320 µM eckol concentration after 2 hours of treatment(77.6%) and 480 µM eckol concentration inhibited proliferation ~50% (2 hours 47.7%, 4 hours 48%, 6 hours 47.2%, and 8 hours 45.9%) in all-time analyses (Figure 3A). The SK-BR-3 proliferation was significantly ~20% (2 hours 84.2%, 4 hours 83.8%, 6 hours 81.4% and 8 hours 81.5%) decreased after 480 µM eckol treatment in all-time analysis (Figure 3B). As a result, compatible with the AhR gene expression profile, the triple-negative MDA-MB-231 breast cancer cells which have high AhR gene expression, were more sensitive to eckol treatments at high concentrations compared to SK-BR-3 cells (480 µM).
Figure 3: Effect of eckol on the proliferation of MDA-MB-231 and SK-BR-3 cells. MDA-MB-231 cells proliferation analysis, cells exposed control– different concentrations of eckol for 2, 4, 6 and 8 hours (A). B) SK-BR-3 cells treated with control- different concentrations of eckol for 2, 4, 6 and 8 hours (B). aindicates p<0.05, bp<0.01, cp<0.001
Effect of eckol on apoptosis in breast cancer cell lines
After the determination of the cell proliferation role of eckol on breast cancer cell models, we assessed whether the observed cellular growth inhibition by eckol treatment was accompanied by apoptotic death in two different concentrations in two cell lines to a minimum time manner (2 hours). We selected 160 µM which did not significantly affect cell line proliferation and 480 µM concentrations which significantly affected proliferation on both cell lines conversely. Following treatment with 160 µM of eckol, the percentage of early apoptotic cells was significantly different on treated compared to untreated MDA-MB-231 cells (6.0 ± 0.3% vs 0.1 ± 0.0% in controls; Figure 4A), at 2 hours of treatment. After 2 hours of treatment with 480 µM eckol, MDA-MB-231 cells displayed significantly higher levels of apoptosis compared to untreated cells, totally in over half of MDA-MB-231 cells were apoptotic; early apoptosis was 0.1% in control vs 8.2% in 480 µM eckol treated and late apoptosis 1.7% in control vs 50.6% in 480 µM eckol treated, respectively (Figure 4A). SK-BR-3 cells were treated with 160 µM for 2 hours and the apoptotic cell rate did not significantly change early apoptotic cell rate (2% in 160 µM eckol treated vs. 1.6% in controls). In a dose-dependent manner, SK-BR-3 cell responses were changed and the late apoptotic cell rate was significantly increased at 480 µM compared to control cells, 19.4% and 4.8%, respectively (Figure 4B). The proliferation results were consistent with the apoptosis data, suggesting that eckol treatment more effectively inhibits proliferation and also caused nearly 60% of the cells to apoptotic in MDA-MB-231 cells, which is a high AhR expressed cell line.
Figure 4: Eckol treatment caused apoptotic cell death in MDA-MB-231 and SK-BR-3 cell lines. A) The apoptotic cell rate was analyzed in MDA-MB-231 cells after incubation with eckol at increasing concentrations (C, 160, and 480 µM) for 2 hours B) SK-BR-3 cells treated with C, 160, and 480 µM eckol concentrations for 2 hours. Apoptotic cell rate was analyzed by flow cytometry. aindicates p<0.05, bp<0.01, cp<0.001
Effect of eckol on cell cycle progression
Given our data on the effect of eckol on the inhibition of cellular growth and apoptosis, we tested the effect of eckol at a concentration of 160 µM and 480 µM on cell cycle progression at 2 hours of treatment on MDA-MB-231 and SK-BR-3 cell lines. As shown in Figure 5A, 2 hours of 160 µM eckol treatment resulted in significant cell cycle arrest at the G1 phase (58.5% vs. 46.1% in controls) and 480 µM treatment caused G2/M arrest in MDA-MB-231 cells (60.1% vs. 24.7% in controls). G1 arrest was not detected after 160 (57.9%) and 480 µM (55.9%) eckol treatment in SK-BR-3 cells (56.4% in controls). G2/M phase cell amount was increased at 480 µM (21.1%) compared to 160 µM (15.8%) but cells did not significantly arrest compare to control (19.9% in controls) (Figure 5B). Cell cycle responses to eckol treatment were similar to proliferation and apoptosis responses in MDA-MB-231 cells, on the contrary, SK-BR-3 cells cell cycle progression rate was not significantly changed after eckol treatment.
Figure 5: Eckol treatment after 2 hours cell cycle analysis. MDA-MB-231 cells were exposed to 160 and 480 µM eckol for 2 hours for determinization of the cell cycle phase (A). SK-BR-3 cells were treated with 160 and 480 µM eckol for 2 hours for determinization of the cell cycle phase (B). aindicates p<0.05, bp<0.01, cp<0.001
Discussion
Humans have long used naturally occurring substances for medical purposes. Plants, in particular, have played a leading medical role in most cultures (Dyshlovoy and Honecker, 2015). Cancer is the second leading cause of death in the world and breast cancer is the first cause of mortality in the female population. Breast cancer is a complex and heterogeneous disease and is well-characterized according to molecular profile (Yersal and Barutca, 2014). Breast cancer is modeled by cell lines according to molecular classification and cell lines have been widely used for anti-cancer drug studies. For breast cancer treatment, multiple chemotherapeutic drugs use, such as doxorubicin, paclitaxel, cisplatin, 5-fluorouracil, and these drugs target proliferative cells without cancer cell-specific molecular targeting (Fisusi and Akala, 2019). Although breast cancer is a well-determined cancer type, still research work on new therapeutic strategies for breast cancer cure.
The anti-cancer effect of purified phlorotannin eckol has been determined on various cancer cell lines including breast cancer cell line MCF-7 (Jun et al., 2014; Mwangi et al., 2017; Zhang et al., 2019). However, the target of eckol has not been identified yet. For this purpose, first, we detected molecular target of the eckol in the Protox II database. Database results showed us that AhR is a possible target of eckol with a high probability rate. The AhR protein, a basic helix-loop-helix/per-arnt-sim (bHLH/PAS) family member, is a ligand-activated transcription factor that is an essential regulator of a broad spectrum of biological activities. The binding of a ligand to AhR generates conformational changes and AhR translocates to the nucleus. AhR dimerizes with its partner protein AhR nuclear translocator (ARNT) and the PAS-A domain dictates AHR–ARNT dimerization and complex binds to xenobiotic-responsive elements (XREs) in the regulatory region of target genes, inducing their transcription (Paris et al., 2021). The overexpression of AhR mRNA levels were detected in many cancers, including breast, lung, thyroid, and oral squamous cell carcinoma (Su et al., 2013; Li et al., 2014; Stanford et al., 2016; Vacher et al., 2018; Moretti et al., 2020). The previous study pointed out that the AhR is a potential target for breast cancer therapy (Powell et al., 2013).
Next, we analyzed eckol-AhR interaction with protein docking analysis. The in silico docking result revealed that eckol binds the AhR protein and this binding include the PAS-A domain which is essential for the dimerization of AhR protein (Corrada et al., 2016).
Concerning that results, we decided to research the role of eckol on breast cancer cells according to the AhR expression profile. According to the European Bioinformatics Institute database results, triple-negative breast cancer cell line MDA-MB-231 cells expressed higher levels of AhR than SK-BR-3 HER2 enriched cells. These results were compatible with previous data that showed the AhR expression profile in breast cancer cell lines (Gilbert et al., 2018).
The AhR is a ligand-activated transcription factor and mediates the effects of a large class of polycyclic and polyhalogenated aromatic hydrocarbons pollutants on cellular toxicity. Furthermore, recent studies pointed out that AhR has cellular functions including proliferation, cell cycle, pluripotency, and stemness (Baker et al., 2019). Interestingly, the cellular functions of AhR match cancer the hallmarks, and new studies showed that AhR overexpression is important for breast cancer and AhR is a remarkable drug target candidate for breast cancer cure (Vacher et al., 2018; Mohamed et al., 2019). Identification of eckol target AhR and the importance of AhR in breast cancer prompted us to analyze eckol role in the proliferation of breast cancer cell lines dependent on AhR expression profile. We treated cells with various concentrations and times with eckol and eckol has antiproliferative effect in both breast cancer cell lines. Interestingly the responses of the MDA-MB-231 cell line were more sensitive to eckol treatment compared to the SK-BR-3 cell line. Although low AhR expressed cell line SK-BR-3 proliferation was decreased ~20 percent, high AhR expressed cell line MDA-MB-231 cell line proliferation was decreased ~50% after eckol treatment.
In this apoptosis analysis, we found that in response to the 160 µM concentration of eckol treatment MDA-MB-231 cells had significantly higher rates of early apoptosis than controls but apoptotic response of SK-BR-3 cells to eckol treatment was similar to control conditions. In a dose-dependent manner, MDA-MB-231 cells apoptotic rate was over half percent after 480 µM eckol treatment. On the contrary, eckol has a protective role against apoptosis, such as lethal radiation or oxidative stress (Zhang et al., 2008; Piao et al., 2012). Only limited data is available about the effect of eckol on the cell cycle. After eckol treatment, pancreatic cancer cells accumulated in the sub-G1 phase in the cell cycle (Zhang et al., 2019). Our analysis demonstrated that cell cycle progression changed in a dose-dependent manner; we observed an accumulation of cells in the G2/M-phase in MDA-MB-231 cells. On the contrary, SK-BR-3 cell cycle progression did not change significantly after the eckol treatment compared to untreated cells.
Conclusion
The present results suggest that eckol interacts with AhR protein and there are eckol response differences associated with AhR gene expression in breast cancer subtypes.
Acknowledgement
I would like to thank Dilek Unal and Inci Tuney Kızılkaya for eckol reagent.
References
Baker JR, Sakoff JA, McCluskey A. The aryl hydrocarbon receptor (AhR) as a breast cancer drug target. Med Res Rev. 2020; 40: 972-1001.
Bray F, Ferlay J, Soerjomataram I, Siegel RL, Torre LA, Jemal A. Global cancer statistics 2018: GLOBOCAN estimates of incidence and mortality worldwide for 36 cancers in 185 countries. CA: Cancer J Clin. 2018; 68: 394-424.
Buyel J. F. Plants as sources of natural and recombinant anti-cancer agents. Biotechnol Adv. 2018; 36: 506-20.
Cardoso F, Harbeck N, Barrios CH, Bergh J, Cortés J, El Saghir N, Francis PA, Hudis CA, Ohno S, Partridge AH, Sledge GW. Research needs in breast cancer. Ann Oncol. 2017; 28: 208-17.
Corrada D, Soshilov AA, Denison MS, Bonati L. Deciphering dimerization modes of PAS domains: Computational and experimental analyses of the AhR: ARNT complex reveal new insights into the mechanisms of AhR transformation. PLoS Comput Biol. 2016; 12: e1004981.
Demain AL, Vaishnav P. Natural products for cancer chemotherapy. Microb Biotechnol. 2011; 4: 687-99.
Dyshlovoy SA, Honecker F. Marine compounds and cancer: Where do we stand? Mar Drugs. 2015; 13: 5657-65.
Fisusi FA, Akala EO. Drug combinations in breast cancer therapy. Pharm Nanotechnol. 2019; 7: 3-23.
Gilbert J, De Iuliis GN, Tarleton M, McCluskey A, Sakoff JA. (Z)-2-(3, 4-Dichlorophenyl)-3-(1H-pyrrol-2-yl) acrylonitrile exhibits selective antitumor activity in breast cancer cell lines via the aryl hydrocarbon receptor pathway. Mol Pharmacol. 2018; 93: 168-77.
Jun YJ, Lee M, Shin T, Yoon N, Kim JH, Kim HR. Eckol enhances heme oxygenase-1 expression through activation of Nrf2/JNK pathway in HepG2 cells. Molecules 2014; 19: 15638-52.
Kim AD, Kang KA, Piao MJ, Kim KC, Zheng J, Yao CW, Cha JW, Hyun CL, Kang HK, Lee NH, Hyun JW. Cytoprotective effect of Eckol against oxidative stressâ€induced mitochondrial dysfunction: Involvement of the Foxo3a/Ampk path-way. J Cell Biochem. 2014; 115: 1403-11.
Kozak A, Unal D, Tuney-Kizilkaya I. Seasonal variations of epiphytic flora, abscisic acid production and physiological response in the brown alga Cystoseira foeniculacea (Linnaeus) Greville. Cah Biol Mar. 2020; 61: 169-79.
Li ZD, Wang K, Yang XW, Zhuang ZG, Wang JJ, Tong XW. Expression of aryl hydrocarbon receptor in relation to p53 status and clinicopathological parameters in breast cancer. Int J Clin Exp Pathol. 2014; 7: 7931.
Manandhar B, Paudel P, Seong SH, Jung HA, Choi JS. Characterizing eckol as a therapeutic aid: A systematic review. Mar Drugs. 2019; 17: 361.
MartÃnez Andrade KA, Lauritano C, Romano G, Ianora A. Marine microalgae with anti-cancer properties. Mar Drugs. 2018; 16: 165.
Mohamed HT, Gadalla R, El-Husseiny N, Hassan H, Wang Z, Ibrahim SA, El-Shinawi M, Sherr DH, Mohamed MM. Inflammatory breast cancer: Activation of the aryl hydrocarbon receptor and its target CYP1B1 correlates closely with Wnt5a/b-β-catenin signalling, the stem cell phenotype and disease progression. J Adv Res. 2019; 16: 75-86.
Moretti S, Nucci N, Menicali E, Morelli S, Bini V, Colella R, Mandarano M, Sidoni A, Puxeddu E. The aryl hydrocarbon receptor is expressed in thyroid carcinoma and appears to mediate epithelial-mesenchymal-transition. Cancers 2020; 12: 145.
Mwangi HM, Njue WM, Onani MO, Thovhoghi N, Mabusela WT. Phlorotannins and a sterol isolated from a brown alga Ecklonia maxima, and their cytotoxic activity against selected cancer cell lines HeLa, H157 and MCF7. Interdiscip J Chem. 2017; 2: 1-6.
Paris A, Tardif N, Galibert MD, Corre S. AhR and cancer: From gene profiling to targeted therapy. Int J Mol Sci. 2021; 22: 752.
Pawar SS, Rohane SH. Review on discovery studio: An important tool for molecular docking. Asian J Res Chem. 2021; 14: 86-88.
Piao MJ, Lee NH, Chae S, Hyun JW. Eckol inhibits ultraviolet B-induced cell damage in human keratinocytes via a decrease in oxidative stress. Biol Pharm Bull. 2012; 35: 873-80.
Picot MC, Bender O, Atalay A, Zengin G, Loffredo L, Hadji-Minaglou F, Mahomoodally MF. Multiple pharmacological targets, cytotoxicity, and phytochemical profile of Aphloia theiformis (Vahl.) Benn. Biomed Pharmacother. 2017; 89: 342-50.
Powell JB, Goode GD, Eltom SE. The aryl hydrocarbon receptor: A target for breast cancer therapy. J Cancer Ther. 2013; 4: 1177.
Rajan DK, Mohan K, Zhang S, Ganesan AR. Dieckol: A brown algal phlorotannin with biological potential. Biomed Pharmacother. 2021; 142: 111988.
Rieger AM, Nelson KL, Konowalchuk JD, Barreda DR. Modified annexin V/propidium iodide apoptosis assay for accurate assessment of cell death. JoVE. 2011; 2011.
Seeliger D, de Groot BL. Ligand docking and binding site analysis with PyMOL and Autodock/Vina. J Comput Aided Mol Des. 2010; 24: 417-22.
Stanford EA, Ramirez-Cardenas A, Wang Z, Novikov O, Alamoud K, Koutrakis P, Mizgerd JP, Genco CA, Kukuruzinska M, Monti S, Bais MV. Role for the aryl hydrocarbon receptor and diverse ligands in oral squamous cell carcinoma migration and tumorigenesis: Role of the AhR in oral cancer migration and tumorigenesis. Mol Cancer Res. 2016; 14: 696-706.
Su JM, Lin P, Chang H. Prognostic value of nuclear translocation of aryl hydrocarbon receptor for non-small cell lung cancer. Anti-cancer Res. 2013; 33: 3953-61.
Vacher S, Castagnet P, Chemlali W, Lallemand F, Meseure D, Pocard M, Bieche I, Perrot-Applanat M. High AHR expression in breast tumors correlates with expression of genes from several signaling pathways namely inflammation and endogenous tryptophan metabolism. PloS One. 2018; 13: e0190619.
Waks AG, Winer EP. Breast cancer treatment: A review. JAMA. 2019; 321: 288-300.
Yao, XY, Bai Q. Taraxerol protects the human hepatic L02 cells from hydrogen peroxide-induced apoptosis. Bangladesh J Pharmacol. 2017; 12: 133-39.
Yersal O, Barutca S. Biological subtypes of breast cancer: Prognostic and therapeutic implications. World J Clin Oncol. 2014; 5: 412.
Zhang C, Li X, Kim SK. Application of marine biomaterials for nutraceuticals and functional foods. Food Sci Biotechnol. 2012; 21: 625-31.
Zhang M, Zhou W, Zhao S, Li S, Yan D, Wang J. Eckol inhibits Reg3A-induced proliferation of human SW1990 pancreatic cancer cells. Exp Ther Med. 2019; 18: 2825-32.
Zhang R, Kang KA, Piao MJ, Ko DO, Wang ZH, Lee IK, Kim BJ, Jeong IY, Shin T, Park JW, Lee NH. Eckol protects V79-4 lung fibroblast cells against γ-ray radiation-induced apoptosis via the scavenging of reactive oxygen species and inhibiting of the c-Jun NH2-terminal kinase pathway. Eur J Pharmacol. 2008; 591: 114-23.
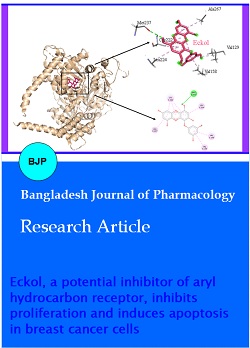