Zoledronic acid inhibits the bone metastasis of breast cancer by repressing exosome-loaded DKK1
Abstract
This study is designed to investigate the mechanism underlying zoledronic acid for the bone metastasis of breast cancer. Dickkopf 1 (DKK1) was both expressed in breast cancer cells and exosomes isolated from breast cancer cells. The expression of DKK1 was synchronously inhibited in breast cancer cells and exosomes with zoledronic acid or transfecting with cDNA3.1-DKK1. The proliferation and migration of MDA-MB-453 cells were greatly repressed and the differentiation and maturity of osteoclasts were dramatically repressed by exosomes derived from zoledronic acid-treated MDA-MB-453 cells. In the co-cultural system of RAW264.7 cells and MDA-MB-453 cells, the proliferation and migration of MDA-MB-453 cells were facilitated by exosomes derived from MDA-MB-453 cells. Lastly, the EMT progression and TGF-β signaling were significantly blocked by exosomes derived from zoledronic acid-treated MDA-MB-453 cells. Zoledronic acid suppressed the bone metastasis of breast cancer by inhibiting the exosomes loaded with DKK1.
Introduction
A high possibility of bone metastasis is reported in breast cancer, which is observed in approximately 70-80% of advanced breast cancer patients (Mundy, 2002; Weilbaecher et al., 2011). The bone metastases in breast cancer belong to osteolytic metastases with a series of complications, such as progressive refractory bone pain, pathological fracture, osteoporosis, spinal cord compression, and hypercalcemia. The life quality of patients is significantly influenced by the bone metastasis and the 5-year survival rate has been significantly decreased (Mundy, 2002). In the normal bone microenvironment, the bone formation mediated by osteoblasts and the bone disruption induced by osteoclasts is maintained with a dynamic equilibrium (Roodman and Silbermann, 2015). Osteoclasts originate from the monocyte-macrophage system and are only cells with osteolytic destructive function (Clezardin 2011). Mature osteoclasts are characterized by multiple nuclei and large size, which induce osteolysis destruction by secreting acids and proteases (Boyce, 2013). Currently, several important factors have been found involved in the molecular mechanism of inducing the differentiation and maturity of osteoclasts, such as Dickkopf-1 (DKK-1), RANKL, and M-CSF (Miyamoto, 2011). As the endogenous inhibitor for the Wnt signal pathway, DKK-1 is reported to suppress bone formation by inhibiting the differentiation from adipocytes to osteoblasts (Chen et al., 2007) and activates osteoclasts to trigger osteolysis destruction (Qiang et al., 2010). DKK-1 has been regarded as an important biomarker for bone loss (Huang et al., 2018).
The development of bone metastasis is closely related to the regulatory effects of cancer cells on the bone microenvironment. Disseminated tumor cells (DTCs) induce the formation of metastatic niche in the bone microenvironment by secreting such cytokines as parathyroid hormone-related protein (PTHrP), heparanase (HPSE), and osteopontin (OPN), which further facilitates the planting of tumor cells in the bone microenvironment (Ell and Kang, 2012). Planted tumor cells recruit osteoclasts and induce the overactivation and mature of osteoclasts by interacting with osteoblasts and osteoclasts, which further induces the serious reac-tion of osteolysis destruction on the interface between bone tissues and tumor tissues. The growth factors located in bone tissues are triggered to be released into the bone microenvironment, which further facilitates the proliferation of tumor cells (Weilbaecher et al., 2011).
Exosomes are a group of 30-100 nm extracellular vesi-cles generated from endocytosis (Kahlert and Kalluri, 2013), which mediate the communication among cells by delivering signals through releasing multiple types of biomolecules, such as nucleic acids and proteins, into the recipient cells (Zhang et al., 2015). It is reported that tumor cell-derived exosomes contribute to the growth and proliferation of tumor cells by releasing oncogenic miRNAs (Rabinowits et al., 2009; Bryant et al., 2012). Recently, researchers propose that bone microenvironment can be regulated by tumor cell-derived exosomes to facilitate the bone metastasis of tumor cells (Chicon-Bosch and Tirado, 2020; Yuan et al., 2021).
Bone-modifying agents therapy was one of the treatment options for bone metastasis. Of the medications for bone metastases, bisphosphonates were used to treat bone metastasis, which could help to suppress the breakdown of bone and improve bone density (Krempien et al., 2005). Bisphosphonates treatment might be the pros outweigh the cons among breast cancer patients with metastatic disease (Goldvaser et al., 2019).
Zoledronic acid is the third generation of bisphosphonates and is mainly used for the treatment of oncological bone metastasis and anti-metabolic bone disease. However, the underlying mechanism for the therapeutic effects of zoledronic acid against bone metastasis remains unknown. The present study aims to explore the potential mechanism of zoledronic acid against bone metastasis in breast cancer by investigating the effects of zoledronic acid on the exosomes extracted from breast cancer cells.
Materials and Methods
Cell lines, reagents, and transfection
Breast cancer cells (MDA-MB-453 and MDA-MB-231) and mouse macrophages (RAW264.7) were purchased from ATCC (USA) and cultured in DMEM medium containing 10% FBS and 1% penicillin/streptomycin under the condition of 37℃ and 5% CO2. Zoledronic acid was obtained from Sigma-Aldrich (USA). To establish DKK1-overexpressed breast cancer cells, cells were transfected with the vector containing pcDNA3.1-DKK1 (Genscript, China), together with the transfection reagent lip 3000 (Invitrogen, USA). The efficacy of transfection was identified using the Western blotting assay.
Extraction of exosomes
After incubating for 48 hours, the medium was changed to serum-free DMEM medium, followed by 48-hour incubation and centrifugation at 300 × g for 10 min, 2,000 × g for 10 min, and 10,000 × g for 30 min at 4°C successively. Subsequently, the supernatants were collected and centrifugated at 4°C and 100,000 × g for 70 min, followed by washing and resuspending using the PBS buffer. The extracted exosomes were stored at -80°C for the subsequent experiments.
Transmission electron microscopy (TEM) for the identification of extracted exosomes
The extracted exosomes were fixed using 2.5% glutaraldehyde at 4°C for 12 hours, followed by being loaded onto formvar/carbon-coated grids. Subsequently, the samples were stained with aqueous phosphotungstic acid for several mins, followed by taking images using a transmission electron microscope (Laird, USA).
Cell viability
In brief, after digesting cells using 0.25% pancreatin, cells were planted on 96-well plates to be incubated for 24 hours, followed by replacing the culturing medium supplemented with 5 mg/mL MTT solution. Then dimethyl sulfoxide was introduced following 10 min incubation. Lastly, the microplate reader (Bruker, USA) was utilized to detect the absorbance at 490 nm (Yan et al., 2018).
Wound healing assay
After seeding cells onto 6-well plates to 80% confluence, the sterile plastic pipette tips were utilized to scrap the cell monolayer, followed by twice washes using the serum-free medium. After different strategies for 24 hours, images of the wound closure were taken using the inverted microscope (Laird, USA) (Liu et al., 2021).
Box I: Transwell Invasion Assay
The migration assay is the change of the cell-covered area (gap closure) over time. The cells are monitored immediately after the gap creation, in most cases by using inverted microscopy.
Requirements
Crystal violet, Fetal bovine serum (10%), Incubator with 5% CO2, Breast cancer cells (MDA-MB-453 and MDA-MB-231) suspensions (1×105 cells), RPMI 1640 medium, Zoledronic acid, Paraformaldehyde, Inverted microscope (Laird, USA), Phosphate-buffered saline, Transwell chamber (Corning, USA)
Procedure
Step 1: The cell suspensions (0.1 mL) containing 1, 5, 10, and 100 μmol/L zoledronic acid were seeded into the Transwell upper chamber.
Step 2: The medium (0.6 mL of RPMI 1640 medium) containing 1% fetal bovine serum was added into the lower chamber
Step 3: All chambers were incubated for 24 hours at 37°C in a humidified air containing 5% CO2.
Step 4: Then, the upper surface of the membrane was removed .
Step 5: Cells in the lower chamber were fixed with 4% paraformaldehyde for 10 min after removing the Transwell chamber.
Step 6: The cells were stained with 0.1% crystal violet for 25 min, washed with phosphate-buffered saline, placed under Inverted microscope and photographed five random fields. Each treatment was repeated three times.
Reference
Yang et al., 2020
Reference (video)
Chen, 2018; Guo et al., 2021
Clone formation assay
Breast cancer cells were planted into the 6-well plates, followed by being incubated for 1 week at 37°C. Cells were then fixed with 4% formaldehyde for 15 min, followed by being stained with GIMSA. Lastly, images were taken using the inverted microscope (Laird, USA) to calculate the number of clones (Zhou et al., 2021).
Tartrate-resistant acid phosphatase (TRAP) staining
After treating with exosomes, RAW 264.7 cells were planted onto the 24-well plates, followed by exchanging the medium every 2 days. After incubating for 9 days, cells were fixed and stained with the TRAP activity staining kit according to the instruction of the manufacturer. Dark red represented TRAP-positive cells under the inverted microscope (Laird, USA) and the number of TRAP-positive multinucleated cells (>3 nuclei) was counted (Song et al., 2019).
Immunostaining assay
RAW 264.7 cells were collected and washed using the PBS buffer and subsequently fixed with 4% paraformal-dehyde on microslide for 10 min, followed by 3 washes, and treated with triton-100 for 10 min. After blocking, cells were incubated with the primary antibody against phalloidine (R&D, USA), PKH67 (R&D, USA) at 37℃ for 1.5 hours, respectively, followed by incubation with solution diluted with secondary antibody (R&D, USA) at 37℃ for 45 min. Lastly, the slides were sealed using nail polish after adding the Hoechst solution. The fluorescence was observed under the fluorescence microscope (KEYENCE, Japan) (Meng et al., 2020).
ELISA assay
The release of CTX, NTX, and TGF-β was determined using the ELISA assay (Elabscience, China). In brief, the supernatant and standards were collected and seeded in the 96-well plates, followed by being incubated for 1 hour at 37℃. After removing the medium, the conjugate solution was added to be incubated for 30 min, followed by adding the TMB solution to be incubated for 15 min. The stop solution was then added to termi-nate the reaction, followed by determining the absorbance at 450 nm with the microplate reader (Bruker, USA) to determine the concentration of the proteins (Gürbüz et al., 2019).
Real-time PCR
The TRIZOL© reagent (Invitrogen, USA) was utilized for the extraction of total RNAs from the chondrocytes in each group, which were further reverseâ€transcribed to cDNA using the TaqMan miRNA reverse transcription kit (Invitrogen, USA). The RT-PCR was conducted with SYBR® green realâ€time PCR master mix (Roche Diagnostics, Switzerland). GAPDH was used for the normalization of the expression of genes, which was determined using the 2−ΔΔCt method (Liang et al., 2007).
Western blot assay
The lysis buffer was utilized to extract total proteins from cells or exosomes and the isolated proteins were quantified with the BCA kit (Takara, Japan), followed by loading 30 μg proteins for each sample onto the 12% SDS-PAGE. After separating for 1 hour, proteins in the gel were further transferred onto the PVDF membrane (Takara, Japan), followed by being incubated with 5% skim milk. Then, the membrane was incubated with the primary antibody against DKK1 (1:1000), CD63 (1:1000), CD81 (1:1000), TRAP (1:1000), OSCAR (1:1000), c-Fos (1:1000), NFATcl (1:1000), E-cadherin (1:1000), β-catenin (1:1000), N-cadherin (1:1000), vimentin (1:1000), SMAD2 (1:1000), SMAD3 (1:1000), SNAIL (1:1000), TWIST (1:1000), ZEB1 (1:1000), ZEB2 (1:1000), and GAPDH (1:1000). Then the secondary antibody (1:2000) was utilized to incubate with the membrane for 1.5 hours under the room temperature. Primary and secondary antibodies were collected from Affintiy, Australia. Lastly, the bands were incubated with the ECL solution, followed by quantification using the Image J software (Ohno et al., 2013).
Statistical analysis
Data achieved in the present study were presented as mean ± SD and analyzed using the GraphPad software. The Student’s t-test was used to analyze the difference between 2 groups and the one-way ANOVA method was used to analyze the differences among groups. P<0.05 was considered a significant difference.
Results
DKK1 could be delivered by zoledronic acid-treated exosomes derived from breast cancer cells
Firstly, 2 breast cancer cell lines with different malignant degrees, MDA-MB-453 (lower malignant degree) and MDA-MB-231 (higher malignant degree), were chosen for the investigation. Compared to MDA-MB-453 cells, significantly higher wound width (Figure 1A) and number of migrated cells (Figure 1B) were observed in MDA-MB-231 cells (p<0.01). The expression level of DKK1 in MDA-MB-231 cells was greatly higher than the expression level of DKK1 in MDA-MB-453 cells (p<0.01). Zoledronic acid is reported to suppress the activity of osteoclasts by downregulating DKK1 (Polyzos et al., 2009; Gobel et al., 2015). To verify the effects of ZA, MDA-MB-231 cells were incubated with different concentrations of zoledronic acid (0, 25, 50, 100, and 200 μM), followed by determining the expression level of DKK1 and extracting exosomes. We found that DKK1 was dramatically downregulated by the treatment of zoledronic acid (p<0.05, p<0.01). Exosomes extracted from MDA-MB-231 cells treated with different concentrations of zoledronic acid were identified by TEM (Figure 1F) and determining the expression level of CD63 and CD81 (Fig 1G). Subsequently, the expression level of DKK1 in MDA-MB-231 exosomes was evaluated. Similar to parent cells, DKK1 in MDA-MB-231 exosomes (Figure 1H-I) was significantly down-regulated by the treatment of zoledronic acid (p<0.05, p<0.01). To further verify the consistency between parent cells and exosomes on the expression level of DKK1, DKK1 overexpressed MDA-MB-231 cells and MDA-MB-453 cells were established. The transfection efficacy was first identified using the PCR (p<0.01) and Western blotting assay (Figure 1J). Exosomes extracted from cells were identified by TEM (Figure 1K) and determining the expression level of CD63 and CD81 (Figure 1L). Compared to the blank vector, the expression level of DKK1 in exosomes extracted from DKK1-overexpressed MDA-MB-231 cells or MDA-MB-453 cells was much higher (p<0.01). These data revealed that DKK1 could be delivered by exosomes extracted from breast cancer cells.
Figure 1: DKK1 was delivered by zoledronic acid-treated breast cancer cells derived exosomes. The migration ability was measured by wound healing assay (A) and transwell assay (B). The expression level of DKK1 in MDA-MB-453 and MDA-MB-231 cells was determined by RT-PCR (C). The gene expression level of DKK1 in MDA-MB-453 cells after treatment of different concentration of zoledronic acid was evaluated by RT-PCR (D). The protein expression level of DKK1 in MDA-MB-453 cells after treatment of different concentration of ZA was evaluated by Western blotting (E). The morphology of extracted exosomes was visualized by TEM (F). The expression level of CD63 and CD81 in extracted exosomes was determined by Western blotting (G). The gene expression level of DKK1 in exosomes derived from zoledronic acid-treated MDA-MB-453 cells was evaluated by RT-PCR (H). The protein expression level of DKK1 in exosomes derived from zoledronic acid-treated MDA-MB-453 cells was evaluated by Western blotting (I). The efficacy of overexpression of DKK1 was evaluated by Western blotting (J). The morphology of extracted exosomes was visualized by TEM (K). The expression level of CD63 and CD81 in extracted exosomes was determined by Western blotting (L). The expression level of DKK1 in exosomes derived from MDA-MB-453 cells and DKK1-overexpressed MDA-MB-453 cells was determined by RT-PCR and Western blotting (M) (p<0.05, p<0.01)
Exosomes derived from zoledronic acid-treated MDA-MB-453 cells suppressed the migration of breast cancer cells
To explore whether zoledronic acid impacted the migration of breast cancer cells by acting on tumor cell decried exosomes, MDA-MB-453 cells were incubated with exosomes extracted from MDA-MB-453 cells treated by 200 μM zoledronic acid or PBS buffer. We found that compared to PBS, significantly lower cell viability (Figure 2A), a smaller number of colonies (Figure 2B) and migrated cells (Figure 2C), and larger wound width (Figure 2D) were observed in MDA-MB-453 cells by the treatment of 200 μM zoledronic acid-incubated MDA-MB-453 cells-derived exosomes (p<0.05, p<0.01). These results revealed that the migration of breast cancer cells was dramatically repressed by zoledronic acid-treated MDA-MB-453 cells-derived exosomes.
Figure 2: Exosomes derived from zoledronic acid-treated MDA-MB-453 cells suppressed the migration of breast cancer cells. Cell viability was detected by MTT assay (A). The number of colonies was detected by the colon formation assay (B). The number of migrated cells was detected by transwell assay (C). The wound width was detected by wound healing assay (D) (p<0.05, p<0.01)
Exosomes derived from zoledronic acid-treated MDA-MB-453 cells inhibited the differentiation and maturity of osteoclasts
Firstly, the TRAP staining assay was conducted to evaluate the activity of bone resorption in osteoclasts. Compared to PBS, a significant more TRAP-positive cell number (Figure 3A, C) was observed in RAW 264.7 cells by the treatment exosomes derived from 200 μM zoledronic acid-treated MDA-MB-453 cells (p<0.01). To identify osteoclasts and trace the location of exosomes in RAW 264.7 cells, the immunostaining assay on phalloidine and PKH67 was conducted. As shown in Figure 3B, compared to PBS, a lower expression level of phalloidine was observed in RAW 264.7 cells incubated with exosomes derived from 200 μM zoledronic acid-treated MDA-MB-453 cells, accompanied by down-regulated PKH67. Subsequently, the expression level of biomarkers of mature osteoclasts was further determined. We found that compared to PBS, TRAP, OSCAR, c-Fos, and NFATc1 (Figure 3D-E) were dramatically down-regulated in RAW 264.7 cells by the treatment exosomes derived from zoledronic acid-treated MDA-MB-453 cells (p<0.01). In addition, the concentration of biochemical markers (Figure 3F-G) of bone metabolism (CTX and NTX) in RAW 264.7 cells incubated with exosomes derived from zoledronic acid- treated MDA-MB-453 cells were greatly lower than that in the PBS group (p<0.01). Lastly, compared to PBS, the production of TGF-β (Figure 3H) was found to decrease in RAW 264.7 cells as time went on by the treatment exosomes derived from zoledronic acid-treated MDA-MB-453 cells (p<0.05, p<0.01). These results revealed that differentiation and maturity of osteoclasts were drastically suppressed by exosomes derived from zoledronic acid-treated MDA-MB-453 cells.
Figure 3: Exosomes derived from zoledronic acid-treated MDA-MB-453 cells inhibited the differentiation and maturity of osteoclasts. The differentiation of osteoclasts was evaluated by TRAP staining assay (A). The identification of osteoclasts tracing the location of exosomes in RAW 264.7 cells were determined by immunostaining assay on phalloidine and PKH67, respectively (B). The quantification of TRAP staining assay was recorded (C). The gene expression level of TRAP, OSCAR, c-Fos, and NFATcl was determined by RT-PCR (D). The protein expression level of TRAP, OSCAR, c-Fos, and NFATcl was determined by Western blotting (E). The concentration of CTX was measured by the ELISA assay (F). The concentration of NTX was measured by the ELISA assay (G). The concentration of TGF-β was measured by the ELISA assay (H) (p<0.05, p<0.01)
Effects of exosomes-treated RAW 264.7 cells on the proliferation and migration of breast cancer cells
To further explore the interaction between exosomes-treated RAW 264.7 cells and breast cancer cells, the proliferation and migration ability of MDA-MB-453 cells were evaluated following the co-culture of exosomes or PBS-treated RAW 264.7 cells and MDA-MB-453 cells. Compared to the PBS group, significantly higher cell viability (Figure 4A), a greater number of colonies (Figure 4B) and migrated cells (Figure 4D), and a smaller wound width (Figure 4C) were found in MDA-MB-453 cells incubated with exosomes derived from MDA-MB-453 cells.
Figure 4: The effects of exosomes treated RAW 264.7 cells on the proliferation and migration of breast cancer cells. Cell viability was detected by MTT assay (A). The number of colonies was detected by the colon formation assay (B). The number of migrated cells was detected by transwell assay (C). The wound width was detected by wound healing assay (D) [p<0.05, p<0.01]
Exosomes derived from zoledronic acid-treated MDA-MB-453 cells suppressed the EMT progression and TGF-β signal pathway
We further checked the effects of exosomes on the EMT progression and TGF-β signal pathway to explore the potential mechanism. Compared to the PBS group, the expression level of E-cadherin and β-catenin was greatly elevated and the expression level of N-cadherin and vimentin was dramatically declined by the treatment of exosomes derived from zoledronic acid-treated MDA-MB-453 cells. In addition, compared to PBS, SMAD2, SMAD3, SNAIL, TWIST, ZEB1, and ZEB2 were dramatically down-regulated by the treatment of exosomes derived from zoledronic acid-treated MDA-MB-453 cells.
Figure 5: Exosomes derived from zoledronic acid-treated MDA-MB-453 cells suppressed the EMT progression and TGF-β signal pathway. The expression level of E-cadherin, β-catenin, N-cadherin, and vimentin was determined by Western blotting (A). The expression level of SMAD2, SMAD3, SNAIL, TWIST, ZEB1, and ZEB2 was determined by Western blotting (B)
Discussion
The formation of osteoclasts is precisely regulated by the osteoprotegerin ligand (OPGL)/RANKL/RANK system. RANKL facilitates the differentiation from precursor osteoclasts to mature osteoclasts by binding with RANK located on the membrane of osteoclasts (Khosla 2001). Wnt/β-catenin axis regulates the differentiation and maturity of osteoclasts by mediating the expression level of RANKL on osteoblasts (Glass et al., 2005). The mRNA expression levels of RANKL on osteoblasts were suppressed by the activation of Wnt/β-catenin pathway, which contributed to the declined production of osteoclasts and the increase of bone mass (Spencer et al., 2006).
The facilitated proliferation of osteoclasts will be facilitated, the bone mass will be decreased, and the bone density will be declined by the knockdown of β-catenin (Holmen et al., 2005). When investigating the function of DKK family in the differentiation progression from mesenchymal cells to osteoblasts, the expression level of M-CSF and RANKL could be elevated by DKK1, which further induced the down-regulation of OPG and the production of osteoclasts (Fujita and Janz, 2007). Therefore, as an important inhibitor of Wnt/β-catenin pathway, DKK1 exerts important roles in facilitating the differentiation and maturity of osteoclasts. We found that DKK1 was highly expressed in breast cancer cells, which was significantly down-regulated by zoledronic acid, an inhibitor of osteoclasts. Interestingly, after the treatment of zoledronic acid, DKK1 in exosomes derived from breast cancer cells was also down-regulated, indicating that DKK1 might be delivered by exosomes from breast cancer cells. To verify our hypothesis, the DKK1 overexpressed breast cancer cells were established. We found that DKK1 was also up-regulated in exosomes extracted from DKK1 overexpressed breast cancer cells. Therefore, we suspected that exosomes carrying DKK1 might be an important stimulator for the differentiation and maturity of osteoclasts, which contributed to bone metastasis.
Tumor-stromal interaction exerts an important role in facilitating the bone metastasis of breast cancer and the tumor-stromal interface during bone metastasis is mainly composed of tumor cells and osteoclasts (Liotta and Kohn 2001; Lynch et al., 2005). The differentiation and maturity of osteoclasts can be stimulated by cytokines released by tumor cells to induce the degradation of bone stromal and the production of growth factors stored in the bone stromal, which further facilitates the growth and proliferation of tumor cells (Weilbaecher et al., 2011). It is important to investigate the molecular mechanism underlying the tumor-stromal interaction for the diagnosis, prevention, and treatment of bone metastasis of breast cancer (Kang, 2016). We treated breast cancer cells with zoledronic acid and found that DKK1 was significantly down-regulated in extracted exosomes, which were utilized to be incubated with breast cancer cells. As a consequence, the proliferation and migration of treated breast cancer cells were greatly suppressed. In addition, after incubating exosomes with RAW264.7 cells, the differentiation and maturity of osteoclasts were dramatically inhibited. To further verify the effects of exosomes derived from cancer cells on the interaction between breast cancer cells and osteoclasts, exosomes were added into the co-cultural system of RAW264.7 cells and breast cancer cells. We found that the proliferation and migration of breast cancer cells were dramatically facilitated. These data revealed that the differentiation and maturity of osteoclasts could be suppressed by exosomes derived from zoledronic acid-treated breast cancer cells and subsequently prevented the matured osteoclasts from facilitating the proliferation and migration of breast cancer cells.
EMT progression is an important marker for the migra-tion of cancer cells, especially in bone metastasis (Ren et al., 2017). In addition, the production of TGF-β stored in bone stromal will be significantly induced by the stimulation of cytokines released by breast cancer cells and the produced TGF-β further activates the growth of tumor cells by targeting Jagged1 (Kang, 2016). We found that after the treatment of exosomes derived from zoledronic acid-treated MDA-MB-453 cells, the EMT progression and TGF-β pathway were significant-ly suppressed, indicating that the interaction between tumor cells and osteoclasts might be connected by exosomes derived from tumor cells, which were blocked by the treatment of zoledronic acid.
Conclusion
Zoledronic acid inhibits the bone metastasis of breast cancer by repressing exosome-loaded DKK1.
References
Boyce BF. Advances in the regulation of osteoclasts and osteoclast functions. J Dent Res. 2013; 92: 860-67.
Bryant R, Pawlowski T, Catto JW, Marsden G, Vessella RL, Rhees B, Kuslich C, Visakorpi T, Hamdy FC. Changes in circulating microRNA levels associated with prostate cancer. Br J Cancer. 2012; 106: 768-74.
Chen XL. Lycorine inhibits MDA-MB-231 breast cancer cells proliferation, migration and invasion is associated with Wnt/β-catenin signaling. Bangladesh J Pharmacol. 2018; 13: 192-95.
Chen Y, Whetstone HC, Youn A, Nadesan P, Chow EC, Lin AC, Alman BA. Beta-catenin signaling pathway is crucial for bone morphogenetic protein 2 to induce new bone formation. J Biol Chem. 2007; 282: 526-33.
Chicón-Bosch M, Tirado OM. Exosomes in bone sarcomas: Key players in metastasis. Cells 2020; 9: 241.
Clezardin P. Therapeutic targets for bone metastases in breast cancer. Breast Cancer Res. 2011; 13: 207.
Roodman GD, Silbermann R. Mechanisms of osteolytic and osteoblastic skeletal lesions. Bonekey Rep. 2015; 4: 753.
Ell B, Kang Y. SnapShot: Bone metastasis. Cell 2012; 151: 690-90.
Fujita KI, Janz S. Attenuation of WNT signaling by DKK-1 and -2 regulates BMP2-induced osteoblast differentiation and expression of OPG, RANKL and M-CSF. Mol Cancer. 2007; 6: 71.
Glass II DA, Bialek P, Ahn JD, Starbuck M, Patel MS, Clevers H, Taketo MM, Long F, McMahon AP, Lang RA, Karsenty G. Canonical Wnt signaling in differentiated osteoblasts controls osteoclast differentiation. Dev Cell. 2005; 8: 751-64.
Göbel A, Browne AJ, Thiele S, Rauner M, Hofbauer LC, Rachner TD. Potentiated suppression of Dickkopf-1 in breast cancer by combined administration of the mevalonate pathway inhibitors zoledronic acid and statins. Breast Cancer Res Treat. 2015; 154: 623-31.
Goldvaser H, Amir E. Role of bisphosphonates in breast cancer therapy. Curr Treat Options Oncol. 2019, 20: 1-17.
Guo HYB, Liu XX, Zhu XY, Yu Z. Mulberrofuran G inhibits proliferation and migration by inactivating JAK2/STAT3 signaling in lung cancer cells. Bangladesh J Pharmacol. 2021; 16: 134-40.
Gürbüz P, Düzova H, Yildiz A, Çakan P, Kaya GB, Bağ HGG, Durhan M, Gül CC, Taşlidere AÇ. Effects of noopept on cognitive functions and pubertal process in rats with diabetes. Life Sci. 2019; 233: 116698.
Holmen SL, Zylstra CR, Mukherjee A, Sigler RE, Faugere MC, Bouxsein ML, Deng L, Clemens TL, Williams BO. Essential role of beta-catenin in postnatal bone acquisition. J Biol Chem. 2005; 280: 21162-68.
Huang Y, Liu L, Liu A. Dickkopf-1: Current knowledge and related diseases. Life Sci. 2018; 209: 249-54.
Kahlert C, Kalluri R. Exosomes in tumor microenvironment influence cancer progression and metastasis. J Mol Med (Berl). 2013; 91: 431-37.
Kang Y. Dissecting tumor-stromal interactions in breast cancer bone metastasis. Endocrinol Metab (Seoul). 2016; 31: 206-12.
Khosla S. Minireview: The OPG/RANKL/RANK system. Endocrinology 2001; 142: 5050-55.
Krempien R, Niethammer A, Harms W, Debus J. Bisphosphonates and bone metastases: Current status and future directions. Expert Rev Anticancer Ther. 2005; 5: 295-305.
Liang Z, Wu H, Reddy S, Zhu A, Wang S, Blevins D, Yoon Y, Zhang Y, Shim H. Blockade of invasion and metastasis of breast cancer cells via targeting CXCR4 with an artificial microRNA. Biochem Biophys Res Commun. 2007; 363: 542-546.
Liotta LA, Kohn EC. The microenvironment of the tumour-host interface. Nature 2001; 411: 375-79.
Liu S, Wang L, Zhang R. Corylin suppresses metastasis of breast cancer cells by modulating miR-34c/LINC00963 target. Libyan J Med. 2021; 16: 1883224.
Lynch CC, Hikosaka A, Acuff HB, Martin MD, Kawai N, Singh RK, Vargo-Gogola TC, Begtrup JL, Peterson TE, Fingleton B, Shirai T. MMP-7 promotes prostate cancer-induced osteolysis via the solubilization of RANKL. Cancer Cell. 2005; 7: 485-96.
Meng Z, Zhang R, Wang Y, Zhu G, Jin T, Li C, Zhang S. miR-200c/PAI-2 promotes the progression of triple negative breast cancer via M1/M2 polarization induction of macro-phage. Int Immunopharmacol. 2020, 81: 106028.
Miyamoto T. Regulators of osteoclast differentiation and cell-cell fusion. Keio J Med. 2011; 60: 101-05.
Mundy GR. Metastasis to bone: Causes, consequences and therapeutic opportunities. Nat Rev Cancer. 2002; 2: 584-93.
Ohno SI, Takanashi M, Sudo K, Ueda S, Ishikawa A, Matsu-yama N, Fujita K, Mizutani T, Ohgi T, Ochiya T, Gotoh N, Kuroda M. Systemically injected exosomes targeted to EGFR deliver antitumor microRNA to breast cancer cells. Mol Ther. 2013; 21: 185-91.
Polyzos SA, Anastasilakis AD, Efstathiadou Z, Kita M, Litsas I, Avramidis A, Arsos G, Moralidis E, Gerou S, Pavlidou V, Papatheodorou A. The effect of zoledronic acid on serum dickkopf-1, osteoprotegerin, and RANKL in patients with Paget's disease of bone. Horm Metab Res. 2009; 41: 846-50.
Qiang YW, Chen Y, Brown N, Hu B, Epstein J, Barlogie B, Shaughnessy Jr JD. Characterization of Wnt/beta-catenin signalling in osteoclasts in multiple myeloma. Br J Haematol. 2010; 148: 726-38.
Rabinowits G, Gerçel-Taylor C, Day JM, Taylor DD, Kloecker GH. Exosomal microRNA: A diagnostic marker for lung cancer. Clin Lung Cancer. 2009; 10: 42-46.
Ren D, Yang Q, Dai Y, Guo W, Du H, Song L, Peng X. Oncogenic miR-210-3p promotes prostate cancer cell EMT and bone metastasis via NF-kappaB signaling pathway. Mol Cancer. 2017; 16: 117.
Song C, Yang X, Lei Y, Zhang Z, Smith W, Yan J, Kong L. Evaluation of efficacy on RANKL induced osteoclast from RAW264. 7 cells. J Cell Physiol. 2019; 234: 11969-75.
Spencer GJ, Utting JC, Etheridge SL, Arnett TR, Genever PG. Wnt signalling in osteoblasts regulates expression of the receptor activator of NFkappaB ligand and inhibits osteoclastogenesis in vitro. J Cell Sci. 2006; 119: 1283-96.
Weilbaecher KN, Guise TA, McCauley LK. Cancer to bone: A fatal attraction. Nat Rev Cancer. 2011; 11: 411-25.
Yan W, Ma X, Zhao X, Zhang S. Baicalein induces apoptosis and autophagy of breast cancer cells via inhibiting PI3K/AKT pathway in vivo and vitro. Drug Des Devel Ther. 2018, 12: 3961.
Yang SS, Ma S, Dou H, Liu F, Zhang SY, Jiang C, Xiao M, Huang YX. Breast cancer-derived exosomes regulate cell invasion and metastasis in breast cancer via miR-146a to activate cancer associated fibroblasts in tumor microenvironment. Exp Cell Res. 2020, 391: 111983.
Yuan X, Qian N, Ling S, Li Y, Sun W, Li J, Du R, Zhong G, Liu C, Yu G, Cao D. Breast cancer exosomes contribute to pre-metastatic niche formation and promote bone metastasis of tumor cells. Theranostics 2021; 11: 1429-45.
Zhang X, Yuan X, Shi H, Wu L, Qian H, Xu W. Exosomes in cancer: Small particle, big player. J Hematol Oncol. 2015; 8: 83.
Zhou Y, Wang L, Lin H, Wang Y, Hou K. Bufalin inhibits the growth and epithelial to mesenchymal transition of human gastric cancer cells via modulation of MEK/ERK pathway. Bangladesh J Pharmacol. 2021, 16: 27-33.
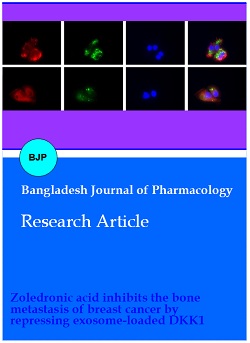