Vigna mungo possesses pharmacological potential for the management of hyperthyroidism and improves estrus cycle disturbances
Abstract
The study was designed to investigate the pharmacological potential of 70% methanolic extract of Vigna mungo for the management of hyperthyroidism and estrus cycle disturbances in female rats. The preliminary phytochemical analysis of the extract was performed. Hyperthyroidism was induced by administering levothyroxine (600 µg/kg; orally) for 14 days. Phytochemical analysis of the extract showed the presence of alkaloids, flavonoids, phenols, saponins, fats, and oils. The extract (300, 500, and 1,000 mg/kg; orally) and carbimazole (30 mg/kg; orally) were administered individually for the next 14 days. The extract showed dose-dependent antithyroid potential, as evidenced by the decrease in T3 and T4, and the increase in TSH levels. Body weight, body temperature, fasting blood glucose levels, and prolonged diestrus phase were reversed towards normal and significant effects on the morphology of the thyroid gland and ovary of the rats were observed. Therefore, the findings reveal that V. mungo has the potential to treat experimentally-induced hyperthyroidism and estrus cycle disturbances.
Introduction
Hyperthyroidism affects 0.2-1.3% of the world's population showing its high prevalence. After a 12-18 month course of antithyroid medication, about 50% of the patients experience a relapse of hyperthyroidism although fewer (15%) reoccurrences with long-term (5–10 years of treatment) antithyroid medication therapy (Wiersinga et al., 2023).
Nutritive natural products are used globally for the management of various disorders. Medicinal natural products provide a vital source for the development of therapeutically active pharmaceutical entities due to their less toxicity in comparison to synthetic drugs (Banik et al., 2021). Plants with reported antithyroid activity include Aegle marmelos, Aloe vera (Kar et al., 2002), Allium sativum, Trigonella foenum-graecum (Tahiliani and Kar, 2003), Althaea officinalis (Shah et al., 2011), Carica papaya (Udoh et al., 2004), Melissa officinalis, Lycopus europaeus, Rosmarinus officinalis, Salvia officinalis and Bacopa monnieri (Singh et al., 2021). Plants reported to be useful to treat menstrual irregularities include Abutilon indicum (Siriwardene et al., 2010), Aloe barbadensis (Radha et al., 2014), Allium cepa (Ghasemzadeh et al., 2013), Camellia sinensis (Chan et al., 2006), Cinnamomum verum (Wang et al., 2007), Corylus avellana (Demirel et al., 2016), Curcuma longa (Reddy et al., 2016), Glycyrrhiza glabra (Armanini et al., 2007), Glycine max (Vargas et al., 2011), Matricaria chamomilla (Zangeneh et al., 2010), Mentha spicata (Rooney and Pendry, 2014), Pergularia daemia (Reddy et al., 2016), Symplocos racemosa (Jadhav et al., 2013) and Trigonella foenum-graecum (Swaroop et al., 2015).
Lentils of genus Vigna are the popular food in Asia. Vigna mungo L. Hepper, commonly known as daal mash, is reported to have antioxidant, immunomodulatory, aphrodisiac, antihyperlipidemic, antiosteoarthritic, anti-diabetic, analgesic, hepatoprotective, nephroprotective, antifungal, antiviral, anti-inflammatory, diuretic and anticonvulsant properties (Zaheer et al., 2020).
The study aimed to investigate the pharmacological potential of V. mungo for the management of thyroxine-induced hyperthyroidism and also the estrus cycle disturbances in female albino rats.
Materials and Methods
Chemicals
Chemicals included thyroxine (GSK, Pakistan), carbimazole (Ray Pharma, Pakistan), crystal violet stain (BDH, India), ketamine (Hungary), xylazine (Prix Pharmaceutical, Pakistan), enzyme-linked immunosorbent assay (ELISA) kits for the determination of TSH, T3, and T4 (DiaMetra, Italy).
Preparation of crude extract
The dried seeds (2 kg) of V. mungo were purchased from the local market of Bahawalpur, Pakistan. The sample was submitted to the herbarium of Pharmacology research laboratory, Department of Pharmacology, Faculty of Pharmacy, The Islamia University of Bahawalpur, Pakistan (voucher No. VM-SD-10-20-169). Lentil seeds were cleaned of dirt, ground into a coarse powder, and soaked in 70:30 methanolic aqueous solution for three days thrice with occasional shaking followed by filtration through muslin cloth and then by Whatman filter paper No. 1. The filtrate was evaporated at reduced pressure and 40-50ºC using a rotary evaporator. The thick semisolid paste was obtained and the percent yield was calculated. The extract was labeled properly and stored in a freezer below 0ºC for future use.
Phytochemical analysis of the crude extract
Phytochemical screening of the crude extract was performed according to the standard test procedures to detect the primary and secondary metabolites; i.e. alkaloids, glycosides, flavonoids, tannins, saponins, coumarins, fats, oil, and phenolic compounds (Table I).
Table I: Methods of qualitative assessment of phytochemicals
Phytoconstituents | Procedure | Indication | Reference |
---|---|---|---|
Alkaloids | 500 mg extract + 8 mL HCl (1%), heated, filtered, 2 mL of filtrate + few drops of Dragendroff's reagent | Red color precipitates | Tiwari et al., 2011 |
Carbohydrates | 500 mg extract + 2 mL of Molisch's reagent, shaken vigorously + concentrated H2SO4 (few drops) | Violet ring at the interphase | Yadav and Agarwala, 2011 |
Coumarins | 1 g extract, boiled + covered with filter paper (soaked in diluted sodium hydroxide), few min later observed filter paper under UV lamp | Yellow color fluorescence | Gilani et al., 2008 |
Fats and oils | Pressed the extract between two filter papers | Oil stain on first filter paper | Marka et al., 2013 |
Flavonoids | 1 g extract + 5 mL water + few drops of lead acetate | Yellow color | Tiwari et al., 2011 |
Glycosides | 500 mg extract + 2 mL chloroform + 2 mL of concentrated H2SO4 | Reddish brown color | Audu et al., 2007 |
Phenols | Extract solution, filtered, filtrate + 5% ferric chloride solution | Bluish black precipitates | Mandal et al., 2013 |
Phlobatannins | 500 mg extract + 2 mL of distilled water, boiled on water bath + HCl (2%) | Red color precipitates | Jana and Shekhawat, 2010 |
Quinones | 100 mg extract + concentrated HCl | Yellow color precipitates | Ugochukwu et al., 2013 |
Resins | 200 mg extract + 5 mL ethanol, poured resulting solution in 10 mL of distilled water | Precipitates | Velavan, 2015 |
Saponins | Boiling water + 500 mg extract, cooled and then shaken vigorously | Froth formation | Zohra et al., 2012 |
Tannins | 500 mg extract + 10 mL distilled water + gelatin solution (1%) (having 10% sodium chloride) | White precipitates | Tiwari et al., 2011 |
Terpenes | Extract + copper acetate solution (1%) | Emerald green color | Mandal et al., 2013 |
Experimental animals
Cyclic female Wistar albino rats, weighing 180-280 g and Swiss albino mice of either sex weighing 20-30 g were employed for the study. Animals were housed in the animal house of the Pharmacology Research Laboratory, Department of Pharmacology, Faculty of Pharmacy, The Islamia University of Bahawalpur. Animals were kept in standard polycarbonate cages under controlled temperature (23 ± 2°C), along with 55 ± 5% humidity and a 12 hour light/dark cycle. Food and water were provided ad libitum and the animals were acclimatized to experimental conditions for one week before the start of the study.
Animal diet
The diet (1 kg) was prepared by mixing poultry feed, choker, and dry milk, obtained from the local market of Bahawalpur. The energy of the diet was calculated as 402 kcal/100 g, containing carbohydrates, protein, fat, fibers, minerals, and moisture (Akram et al., 2023).
Experimental model for induction of hyperthyroidism
Induction of hyperthyroidism was done as described elsewhere with slight modifications (Jabeen et al., 2021). Rats were selected for the study. At day 0, body weight, body temperature, fasting blood glucose levels, and estrus cycle of rats were observed, then they were anesthetized, and blood samples were collected through retro-orbital puncture and sera separated to determine TSH, T3, and T4 levels. Rats were divided into different groups each comprising five to six animals. The control group was administered distilled water (5 mL/kg) and the rest of the groups were intoxicated with thyroxine (600 µg/kg) orally for 14 days. The treatment groups were treated with the extract at doses of 300, 500, and 1,000 mg/kg orally. The standard control group was administered carbimazole (30 mg/kg; orally); whereas, the intoxicated group was administered distilled water (5 mL/kg; orally) for the next 14 days. All the physical parameters; i.e. body weight, body temperature, estrus cycle and biochemical parameters; i.e. serum TSH, T3, T4, and fasting blood glucose levels were observed at different intervals throughout the study.
Determination of body weight, body temperature, and fasting blood glucose levels
The body weight of rats was noted throughout the study from day 0 to 28 using an electronic weighing balance. Body temperature was observed at day 0, 7, 14, 21, and 28 using a digital instant forehead thermometer. They were kept on overnight fasting for 12-14 hours, blood was drawn from the tail vein of animals, and glucose levels were noted using a glucometer at day 0, 7, 14, 21, and 28 of the study.
Box I: Estimation of serum TSH by ELISA
Principle
The ELISA process for the estimation of thyroid-stimulating hormone (TSH) relies on the binding of specific antibodies to the target antigen to detect the presence and quantity of antigens. To enhance the accuracy and sensitivity of the assay, the antibodies used to coat the plate must have a high affinity.
Materials required
ELISA microplate reader; Serum; TMB (3,3',5,5'-tetramethylbenzidine) substrate
Solution
Wash solution: Before using, dilute the 50x concentrated wash solution to a final volume of 1,000 mL by adding distilled water to each vial's contents. Be mindful of the 1:50 dilution ratio for lesser amounts.
Procedure
Step 1: Allow all reagents to reach room temperature (22-28°C) for at least 30 min. Frozen serum specimens were taken and well mixed after thawing.
Step 2: It was performed in duplicate to improve accuracy of the test results. Two wells were prepared for each point of the calibration curve (C0-C6), control, each sample and one for blank
Step 3: Add 50 μL of each calibrator and 50 μL of the each sample into the wells individually.
Step 4: Then add 100 μL of the conjugate.
Step 5: Cover the plate and incubate in dark for 60 min at room temperature
Step 6: After incubation, remove the content of each well and wash all the wells 6 times with 300 μL of diluted wash solution
Step 7: Add 100 μL of TMB substrate, cover the plate and again incubate for 20 min at room temperature
Step 8: After 20 min add 100 μL of stop solution and gently shake the plate.
Step 9: Within five min, read the absorbance (E) at 450 nm to a reference wavelength of 620-630 nm or against blank
Calculation
Calibrators: The calibrators have various TSH concentrations and are calibrated against WHO 2nd IRP 80/558; i.e. the concentration of C0, C1, C2, C3, C4 and C5 is 0, 0.2, 0.5, 2.5, 5.0 and 10 mlU/L respectively.
Calculate the average absorbance for each sample and for each point on the calibration curve (C0-C6). Plot the concentration vs. the mean absorbance of the calibrators (C0-C6). Plot the points with the best-fitting curve (cubic spline or four para-meter logistic). To get the corresponding values of the concentrations given in mlU/L, interpolate the sample values on the calibration curve.
Precautions
All reagents should be stored in refrigerator at 2-8°C
Unused reagents should never be transferred into the original vials to prevent microbiological and chemical contamination
References
Maes et al., 1997
Study of the estrus cycle
To evaluate hyperthyroidism-induced menstrual dis-turbance, estrus cycle of female rats was observed throughout the study. Estrus cycle is usually 4-5 days long in rats and is generally divided into 4 phases, proestrus, estrus, metestrus/pseudodiestrus, and diestrus, but for the convenient characterization of cycle phases, it was divided into three phases; i.e. ‘proestrus’ characterized by nucleated epithelial cells, ‘estrus’ cha-racterized by non-nucleated, keratinized ‘corn flake’ like cells and ‘diestrus’ characterized by predominant neutrophils.
Vaginal smears were collected and observed on day 0, 7, 14, 21, and 28 of the study. The vaginal orifice was flushed with 0.9% normal saline with the help of a dropper and vaginal cell samples were collected. The collected sample was placed on a glass slide to form a smear. After drying the smear, slides were stained with crystal violet stain and dried again. Slides were then observed under a light microscope using a 10x objective lens (Cora et al., 2015).
Determination of TSH, T3, and T4 levels
All the rats were anesthetized by using a combination of ketamine and xylazine (10:1). Blood samples were collected on day 0, 14, and 28, allowed to clot for 30 min, and centrifuged at 5,000 rpm for 15 min to separate serum. Then the levels of TSH, T3, and T4 were determined using an ELISA microplate reader.
Histological analysis of the thyroid gland and ovaries
At the end of the study, the rats were sacrificed, thyroid gland and ovaries of one representative animal from each group were fixed in 10% formalin for histological analysis. Hematoxylin and eosin staining was applied to the tissue sections before being studied under a light microscope with a camera for photomicrography. The images were thoroughly examined to observe morphological changes in the thyroid gland and ovarian tissues among various groups.
Acute toxicity assay
The acute toxicity assay was performed according to the OECD guidelines, to determine the safety of the extract, mice of either sex were included in the acute toxicity assay. Mice were provided food and water ad libitum and were divided randomly into different groups after six hours of fasting. The extract was administered orally at the dose of 2000, 5,000, and 10,000 mg/kg orally. Distilled water (10 mL/kg orally) was administered to the control group. Animals were monitored for any behavioral change or ill health; like changes in body weight, grooming, alertness, convulsions, hyperactivity, lacrimation, salivation, sweating, urination, touch response, pain response, writhing reflex, corneal reflex, gripping reflex, and righting reflex at 0, 0.5, 1, 2, 4, 6, 12, 24, and 48 hours up to 14 days (Chinedu et al., 2013).
Statistical analysis
Graph pad prism 8 was used for the statistical analysis of data. The data were evaluated using a two-way Analysis of Variance (ANOVA) followed by the Bonferroni post hoc test and p< 0.05 was considered statistically significant. The values are expressed as mean ± SEM.
Results
The percent yield of the crude extract was 7.5% (150 g).
Phytochemical analysis
Phytochemical analysis of the crude extract of V. mungo showed the presence of primary and secondary metabolites; i.e. alkaloids, carbohydrates, coumarins, flavonoids, glycosides, phenols, phlobatanins, quinones, resins, saponins, tannins, terpenes, fats, and oils.
Percent change in body weight
The control group showed normal body weight gain throughout the study. Intoxication caused a gradual decrease in the body weight of rats in all the groups. Rats treated with carbimazole as well as crude extract showed restoration in the body weight in a dose-dependent manner (Figure 1A).
Figure 1: Change in body weight (%) and temperature after thyroxine (14 days), and treatment with V. mungo or carbimazole for the next 14 days. Mean ± SEM; n= 5 to 6; nsp>0.05; ap<0.05; bp<0.01 and cp<0.001 vs. respective group after intoxication and zp<0.001 vs. respective group on day 0
Body temperature
The control group did not show any change in body temperature throughout the study. At day 0, rats of all the groups showed normal body temperature. Whereas, after 14 days of intoxication, an increase in body temperature was recorded, which was normalized after 14 days of treatment with carbimazole and crude extract (Figure 1B).
Fasting blood glucose levels
Fasting blood glucose levels of the control group were found normal throughout the study. At day 0, the fasting blood glucose levels of all the rats were also found normal. After 14 days of intoxication, an increase in fasting glucose level was recorded, which was then normalized with the administration of carbimazole or crude extract (Table II).
Table II: Effects of V. mungo on fasting blood glucose levels
Treatment 1 | Treatment 2 | n | Fasting blood glucose level (mg/dL) | ||
---|---|---|---|---|---|
Day 0 | Day14 | Day 28 | |||
None | None | 6 | 71.2 ± 4.3 | 72.2 ± 4.1 | 71.0 ± 0.9 |
Thyroxine | None | 6 | 70.6 ± 2.4 | 118.3 ± 5.2z | 109.3 ± 3.7ns |
Thyroxine | Carbimazole | 6 | 69.6 ± 0.2 | 103.0 ± 2.1y | 63.6 ± 0.8c |
Thyroxine | V. mungo (300 mg/kg) | 5 | 71.5 ± 1.2 | 107.5 ± 5.5z | 73.5 ± 2.4b |
Thyroxine | V. mungo (500 mg/kg) | 6 | 68.5 ± 1.6 | 110.0 ± 3.2y | 73.2 ± 2.4b |
Thyroxine | V. mungo (1,000 mg/kg) | 6 | 67.3 ± 0.8 | 109.3 ± 1.7y | 75.0 ± 1.9c |
Data are mean ± SEM. All drugs and extract were administered orally. Dose of thyroxine and carbimazole was 600 µg/kg and 30 mg/kg respectively; nsp>0.05; ap<0.05; bp<0.01 and cp<0.001: vs. respective group after intoxication; yp<0.01 and zp<0.001 vs. respective group on day 0 |
Estrus cycle
The estrus cycle was observed for the proestrus, estrus, and diestrus phases. It was observed that after 14 days of intoxication with thyroxine, vaginal smears of rats showed disturbance in the estrus cycle with a small number of epithelial or keratinized cells and an abundant number of neutrophils. Most of the rats showed a disturbed estrus cycle even after the 7th day of intoxication. The intoxicated group showed a disturbed cycle till the 28th day and animals remained in the diestrus phase. Reversing of the diestrus phase into normal cyclicity in a dose-dependent manner was observed after the treatment with crude extract in comparison with the carbimazole-treated group (Figure 2).
Figure 2: Effects of different doses of V. mungo on estrus cycle
Serum levels of TSH, T3 and T4
TSH, T3, and T4 levels of the control group remained normal throughout the study. At day 0, levels of TSH, T3, and T4 of all the animals were in normal range. After 14 days of intoxication, TSH levels were found to be decreased, whereas, T3 and T4 levels increased significantly (p<0.001). On the 28th day of the study, the intoxicated group showed a persistent decrease in the levels of TSH as well as an increase in T3 and T4 levels. Whereas, animals treated with carbimazole and crude extract showed a dose-dependent increase in serum TSH (Figure 3A) and a decrease in T3 (Figure 3B) and T4 (Figure 3C) levels, which showed the antithyroid potential of crude extract and the results were found compa-rable with those of carbimazole, the standard drug.
Figure 3: The graph showing serum TSH, T3 and T4 levels before and after 14 days intoxication, and after treatment with V. mungo or carbimazole for the next 14 days. Data are mean ± SEM; n= 5 to 6
Thyroid gland and ovarian histological study
Animals in the control group showed normal cell morphology (Figure 4; Figure 5). However, intoxication caused inflammation, disturbed cell morphology, and hyperplastic nodules in the thyroid gland (Figure 5B) whereas, disturbed cell arrangement and chronic inflammation in the ovarian cells (Figure 5B). The thyroid and ovarian morphology was normalized and inflammation in cells was reduced to negligible in animals treated with carbimazole (Figure 4C; Figure 5C). V. mungo showed dose-dependent effects, normalized the inflammation, and altered cell arrangement in both the thyroid gland (Figure 4D,E,F) and ovarian cells (Figure 5D,E,F), leading to the normal cell morphology. Histology of the thyroid gland and ovarian cells had also been graded based on the severity of inflammation and altered cell morphology (Table III).
Figure 4: The effects of V. mungo on thyroid histology. Control (A); thyroxine (B); carbimazole (C), V. mungo 300 mg/kg (D), 500 mg/kg (E) and 1000 mg/kg (F). Inflammation (thick arrow sign); altered cell morphology (thin arrow sign)
Figure 5: The effects of V. mungo on ovarian histology. Control (A); thyroxine (B), carbimazole (C); V. mungo 300 mg/kg (D); 500 mg/kg (E) and 1,000 mg/kg (F). Inflammation (thick arrow sign); altered cell morphology (thin arrow sign)
Table III: Histological scoring
Treatment 1 | Treatment 2 | n | Thyroid gland | Ovary | ||
---|---|---|---|---|---|---|
Inflammation | Altered cell morphology | Inflammation | Altered cell morphology | |||
None | None | 6 | 0 | 0 | 0 | 0 |
Thyroxine (600 µg/kg) | None | 6 | 4 | 4 | 4 | 4 |
Thyroxine (600 µg/kg) | Carbimazole (30 mg/kg ) | 6 | 0 | 1 | 0 | 1 |
Thyroxine (600 µg/kg) | V. mungo (300 mg/kg) | 5 | 0 | 2 | 3 | 3 |
Thyroxine (600 µg/kg) | V. mungo (500 mg/kg) | 6 | 0 | 1 | 2 | 2 |
Thyroxine (600 µg/kg) | V. mungo (1,000 mg/kg) | 6 | 0 | 0 | 1 | 1 |
All drugs and extract were administered orally. 0 (Normal), 1(Ë‚ 25%), 2 (26-50%), 3 (51-75%), 4 (76-100%) |
Acute toxicity assay
The crude extract was found safe up to the dose of 10 g/kg. None of the animals showed signs of toxicity during the initial 24 hours, no lethality after 48 hours, and no toxicity was observed till 14 days.
Discussion
The present study revealed that decreased TSH and increased T3 and T4 levels after induction with thyroxine were normalized when treated with either carbimazole or V. mungo in a dose-dependent manner. However, the decreased body weight of hyperthyroid rats was regained, and increased body temperature and fasting blood glucose levels were normalized. The prolonged diestrus phase of hyperthyroid rats was also reversed towards the normal conversion of one phase into another. Destruction of thyroid follicles and ovarian cells was also found to be normalized after treatment.
Phytochemical analysis showed the presence of various phytoconstituents including flavonoids which have been reported to possess antithyroid effects, and fats which could be considered responsible to affect TSH secretion, thyroid peroxidase and hepatic deiodinase activity, and T3 binding to nuclear receptors (Bajaj et al., 2016).
As far as mechanism is concerned, thyroid peroxidase catalyzes the oxidation of iodide to iodine in the thyroid gland, which results in the iodination of iodotyrosine and the synthesis of triiodothyronine and thyroxine. Conversion of iodide into iodine by thyroid peroxidase needs H2O2, a key element in thyroid hormone biosynthesis which is produced when NADPH is oxidized to NADP. H2O2, is the substrate used by thyroid peroxidase for oxidation and incorporation of iodine into thyroglobulin. H2O2, in the thyroid gland, is activated by NADPH oxidase induction, which is stimulated by thyroid stimulating hormone and superoxide dismutase which accelerate the rate of iodination in the thyroid gland. Thyroid stimulating hormone, as well as intra-cellular signaling that activates the cAMP and phosphatidyl-inositol cascade, control the formation of the follicular thyroid. The glutathione peroxidase family of antioxidant enzymes, which contain selenium, typically protect the thyroid gland from oxidative damage caused by reactive oxygen species and H2O2. When the blood iodine level drops and the synthesis of thyroid-stimulating hormone rises, there is an increase in the formation of H2O2, which results in severe oxidative damage to the thyroid gland. Reduced selenium levels make it difficult for glutathione peroxidase to eliminate H2O2, which can further increase oxidative stress and damage thyroid tissue (Szanto et al., 2019). Increased reactive oxygen species production also affects the transcription of NRF2 target genes, including respiratory NRF1, which regulates mitochondrial gene expression. The activity of these transcription factors is co-ordinated by the PGC1-α co-activator, a member of the PGC1 co-activator family. It has also been suggested that NRF1 could be directly activated by redox signa-ling. It is reported that the NRF1 content increases in hyperthyroid animals and is attenuated by antioxidant supplementation (Fasciolo et al., 2023). In the present study, antithyroid effects produced by V. mungo may be due to the presence of antioxidant potential of phytoconstituents present in the crude extract.
Fever is a secondary impact of infection, tissue damage, inflammation, and other diseased states. The infected or damaged tissue initiates the enhanced formation of proinflammatory mediators (cytokines; i.e. interleukin 1β and TNF-α), which increase the synthesis of prosta-glandin E2 near the preoptic hypothalamus area and trigger the hypothalamus to elevate body temperature (Snafi, 2018). In the present study, an increase in body temperature could be due to the hypersecretion and inflammation produced in the thyroid gland. As thyroid hormones also regulate metabolism and body tem-perature (Hassan and Ali, 2021), decreased body weight and heat intolerance were observed, which may also be due to increased metabolic activity in the hyperthyroid rats. However, hyperglycemia associated with hyperthyroidism may be due to insulin resistance which has been reported in hyperthyroid patients (Mitrou et al., 2010).
Moreover, hyperthyroidism may also have profound effects on the female reproductive system (Sharma and Sharma, 2012) as evidenced by the prolonged diestrus phase in the current study. However, V. mungo restored estrus cycle regularity completely, which could be due to the presence of saponins. As saponins have been reported to improve progesterone levels (Oshima et al., 2003) which regulate the estrogen receptors during the estrus cycle (Evans et al., 1980).
It has also been reported that disturbances in the levels of serum thyroid hormones may cause changes in the body’s growth and organ development, thus thyroxine-induced hyperthyroidism showed destruction of thyroid follicles (Li and Baloch, 2018) and ovarian cells (Elbandrawy et al., 2018). V. mungo showed a dose-dependent response, as disturbed cell morphology and inflammation induced by thyroxine were significantly normalized at the dose of 1,000 mg/kg. Hence, the aforementioned explanation may lead to the conclusion that V. mungo has the potential to treat experimentally-induced hyperthyroidism and associated complications. However, this study has some limitations. For example, thyroid peroxidase activity, test for free T3 and T4, serum levels of estrogen and progesterone which need to be evaluated in the future. V. mungo possesses diverse types of secondary metabolites which may serve as lead candidates for novel promising medicinal compounds after further exploration and isolation of the pure compounds and to investigate the underlying mechanism(s) to get a deep insight.
Conclusion
V. mungo has antithyroid potential as evidenced by the increase in TSH levels and body weight as well as a decrease in T3, and T4 levels, body temperature and fasting blood glucose levels, reversing of prolonged diestrus phase towards normal estrus cycle and normal cell morphology of thyroid gland and ovaries in rats.
Ethical Issue
Study protocols were approved by Pharmacy Animal and Ethics Committee under reference No. PAEC/2020/26
Acknowledgements
The authors acknowledge Prof. Saeed Ahmad, Department of Pharmaceutical Chemistry, Faculty of Pharmacy, IUB for providing ELISA research facilities. The authors also acknowledge Mr. Abdul Hameed, Department of Life Sciences, IUB for identification of lentils.
References
Akram A, Jamshed A, Anwaar M, Rasheed HMF, Haider SI, Aslam N, Jabeen Q. Evaluation of Caralluma edulis for its potential against obesity, atherosclerosis and hypertension. Dose-Response. 2023; 21: 1-12.
Armanini D, Castello R, Scaroni C, Bonanni G, Faccini G, Pellati D, Bertoldo A, Fiore C, Moghetti P. Treatment of polycystic ovary syndrome with spironolactone plus licorice. Eur J Obstet Gynecol Reprod Biol. 2007; 131: 61-67.
Audu SA, Mohammed I, Kaita HA. Phytochemical screening of the leaves of Lophira lanceolata (Ochanaceae). Life Sci J. 2007; 4: 75-79.
Bajaj JK, Salwan P, Salwan S. Various possible toxicants involved in thyroid dysfunction: A review. J Clin Diagn Res. 2016; 10: FE01-03.
Banik B, Das S, Das MK. Medicinal plants with potent anti-inflammatory and anti-arthritic properties found in eastern parts of the Himalaya: An ethnomedicinal review. Pharmacogn Rev. 2021; 14: 121-37.
Chan CC, Koo MW, Ng EH, Tang OS, Yeung WS, Ho PC. Effects of Chinese green tea on weight, and hormonal and biochemical profiles in obese patients with polycystic ovary syndrome: A randomized placebo-controlled trial. J Soc Gynecol Investig. 2006; 13: 63-68.
Chinedu E, Arome D, Ameh FS. A new method for determining acute toxicity in animal models. Toxicol Int. 2013; 20: 224-26.
Cora MC, Kooistra L, Travlos G. Vaginal cytology of the laboratory rat and mouse: Review and criteria for the staging of the estrous cycle using stained vaginal smears. Toxicol Pathol. 2015; 43: 776-93.
Demirel MA, Ilhan M, Suntar I, Keles H, Akkol EK. Activity of Corylus avellana seed oil in letrozole-induced polycystic ovary syndrome model in rats. Rev Bras Farmacogn. 2016; 26: 83-88.
Elbandrawy M, Taha A, Tousson E, Hassan N. Different stages of hyperthyroidism: Alterations in proliferation, apoptosis, and histology of female rat ovary. Res J Pharm Biol Chem Sci. 2018; 9: 1458-71.
Evans RW, Chen TJ, Hendry WJ, Leavitt WW. Progesterone regulation of estrogen receptor in the hamster uterus during the estrous cycle. Endocrinology 1980; 107: 383-90.
Fasciolo G, Napolitano G, Aprile M, Cataldi S, Costa V, Muscari Tomajoli MT, Lombardi A, Di Meo S, Venditti P. Muscle oxidative stress plays a role in hyperthyroidism-linked insulin resistance. Antioxidants 2023; 12: 592.
Ghasemzadeh A, Farzadi L, Khaki A, Khan Ahmadi S. Effect of Allium cepa seeds ethanolic extract on experimental polycystic ovary syndrome (PCOS) apoptosis induced by estradiol-valerate. Life Sci J. 2013; 10: 170-75.
Gilani AH, Jabeen Q, Khan AU, Shah AJ. Gut modulatory, blood pressure lowering, diuretic and sedative activities of cardamom. J Ethnopharmacol. 2008; 115: 463-72.
Hassan AA, Ali MM. Effect of combined carbimazole and Curcuma longa powder in human thyroid-stimulating hormone and thyroperoxidase antibody in hyperthyroidism. Int J Pharmacol Pharm Sci. 2021; 15: 80-87.
Jabeen Q, Khan MS, Qureshi AW, Rasheed HMF. Effect of Abutilon indicum in thyroxine-induced hyperthyroidism in rat. Bangladesh J Pharmacol. 2021; 16: 103-113.
Jadhav M, Menon S, Shailajan S. Anti-androgenic effect of Symplocos racemosa Roxb. against letrozole induced polycystic ovary using rat model. J Coast Life Med. 2013; 1: 309-14.
Jana S, Shekhawat GS. Phytochemical analysis and antibacterial screening of in vivo and in vitro extracts of Indian medicinal herb: Anethum graveolens. Res J Med Plant. 2010; 4: 206-12.
Kar A, Panda S, Bharti S. Relative efficacy of three medicinal plant extracts in the alteration of thyroid hormone concentrations in male mice. J Ethnopharmacol. 2002; 81: 281-85.
Li VVA, Baloch ZW. The pathology of hyperthyroidism. Front Endocrinol. 2018; 9: 737-37.
Maes M, Mommen K, Hendrickx D, Peeters D, D’Hondt P, Ranjan R, De Meyer F, Scharpe S. Components of biological variation, including seasonality, in blood concentrations of TSH, TT3, FT4, PRL, cortisol and testosterone in healthy volunteers. Clin Endocrinol. 1997; 46: 587-98.
Mandal S, Patra A, Samanta A, Roy S, Mandal A, Mahapatra TD, Pradhan S, Das K, Nandi DK. Analysis of phytochemical profile of Terminalia arjuna bark extract with antioxidative and antimicrobial properties. Asian Pac J Trop Biomed. 2013; 3: 960-66.
Marka R, Talari S, Penchala S, Rudroju S, Swamy Nanna R. Preliminary phytochemical analysis of leaf, stem, root and seed extracts of Arachis hypogaea L. Int J Pharm Sci Rev Res. 2013; 20: 134-39.
Mitrou P, Boutati E, Lambadiari V, Tsegka A, Raptis AE, Tountas N, Economopoulos T, Raptis SA, Dimitriadis G. Insulin resistance in hyperthyroidism: The role of IL6 and TNFα. Eur J Endocrinol. 2010; 162: 121-26.
Oshima M, Gu Y, Tsukada S. Effects of Lepidium meyenii Walp and Jatropha macrantha on blood levels of estradiol-17β, progesterone, testosterone and the rate of embryo implantation in mice. J Vet Med Sci. 2003; 65: 1145-46.
Radha M, Padamnabhi N, Laxmipriya N. Evaluation of Aloe barbadensis mill. Gel on letrozole induced polycystic ovarian syndrome (pcos) rat model: A dose dependent study. Int J Pharm Sci Res. 2014; 5: 5293-300.
Reddy PS, Begum N, Mutha S, Bakshi V. Beneficial effect of curcumin in letrozole induced polycystic ovary syndrome. Asian Pac J Reprod. 2016; 5: 116-22.
Rooney S, Pendry B. Phytotherapy for polycystic ovarian syndrome: A review of the literature and evaluation of practitioners’ experiences. J Herb Med. 2014; 4: 159-71.
Shah SA, Akhtar N, Akram M, Shah PA, Saeed T, Ahmed K, Asif H. Pharmacological activity of Althaea officinalis L. J Med Plant Res. 2011; 5: 5662-66.
Sharma N, Sharma A. Thyroid profile in menstrual disorders. JK Sci. 2012; 14: 14-17.
Singh B, Sundar S, Shukla A. Herbal medicines for thyroid diseases In: Treating endocrine and metabolic disorders with herbal medicines. India, IGI Global, 2021, pp 256-77.
Siriwardene SAD, Karunathilaka LPA, Kodituwakku ND, Karunarathne YAUD. Clinical efficacy of ayurveda treatment regimen on subfertility with polycystic ovarian syndrome (PCOS). J Ayurveda Integr Med. 2010; 31: 24-27.
Snafi AAE. Arabian medicinal plants with analgesic and antipyretic effects: Plant based review (Part 1). IOSR J Pharm. 2018; 8: 81-102.
Swaroop A, Jaipuriar AS, Gupta SK, Bagchi M, Kumar P, Preuss HG, Bagchi D. Efficacy of a novel fenugreek seed extract (Trigonella foenum-graecum, FurocystTM) in polycystic ovary syndrome (PCOS). Int J Med Sci. 2015; 12: 825-31.
Szanto I, Pusztaszeri M, Mavromati M. H2O2 metabolism in normal thyroid cells and in thyroid tumorigenesis: Focus on NADPH oxidases. Antioxidants 2019; 8: 126
Tahiliani P, Kar A. The combined effects of Trigonella and Allium extracts in the regulation of hyperthyroidism in rats. Phytomedicine 2003; 10: 665-68.
Tiwari P, Kumar B, Kaur M, Kaur G, Kaur H. Phytochemical screening and extraction: A review. Int Pharm Sci. 2011; 1: 98-106.
Udoh P, Nju R, Udoh F. Effect of Carica papaya (pawpaw) seeds ethanolic extract on the pituitary, thyroid and parathyroid glands of male Wistar rats. Global J Pure Appl Sci. 2004; 10: 515-17.
Ugochukwu SC, Arukwe Uche I, Ifeanyi O. Preliminary phytochemical screening of different solvent extracts of stem bark and roots of Dennetia tripetala G. Baker. Asian J Plant Sci Res. 2013; 3: 10-13.
Vargas ML, Almario RU, Buchan W, Kim K, Karakas SE. Metabolic and endocrine effects of long-chain versus essential omega-3 polyunsaturated fatty acids in polycystic ovary syndrome. Metabolism 2011; 60: 1711-18.
Velavan S. Phytochemical techniques: A review. World J Sci Res. 2015; 1: 80-91.
Wang JG, Anderson RA, Graham GM, Chu MC, Sauer MV, Guarnaccia MM, Lobo RA. The effect of cinnamon extract on insulin resistance parameters in polycystic ovary syndrome: A pilot study. Fertil Steril. 2007; 88: 240-43.
Wiersinga WM, Poppe KG, Effraimidis G. Hyperthyroidism: Aetiology, pathogenesis, diagnosis, management, complications and prognosis. Lancet Diabetes Endocrinol. 2023; 11: 282-98.
Yadav R, Agarwala M. Phytochemical analysis of some medicinal plants. J Phytol. 2011; 3: 10-14.
Zaheer M, Ahmed S, Hassan MM. A review of medicinal uses, phytochemistry and pharmacology of Vigna mungo (L.) Hepper. J Pharmacogn Phytochem. 2020; 9: 1307-09.
Zangeneh FZ, Minaee B, Amirzargar A, Ahangarpour A, Mousavizadeh K. Effects of chamomile extract on biochemical and clinical parameters in a rat model of polycystic ovary syndrome. J Reprod Infertil. 2010; 11: 169-74.
Zohra SF, Meriem B, Samira S, Muneer M. Phytochemical screening and identification of some compounds from mallow. J Nat Prod Plant Resour. 2012; 2: 512-16.
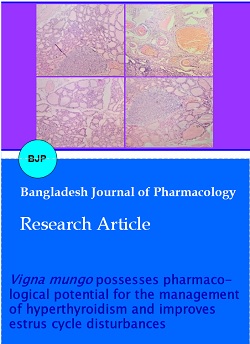