Use of guanine-modified glassy carbon electrode as an electrochemical sensor for the determination of paracetamol
Abstract
In this study, guanine-modified glassy carbon electrode sensor was developed for the determination of paracetamol. The use of overdose continuously for a long time, its metabolites accumulate and affect the kidneys and liver. Therefore, the detection of paracetamol is of great significance. Differential pulse voltammetry and cyclic voltammetry techniques were employed to analyze the behavior of paracetamol in 0.1 M Britton-Robinson buffer solution of pH 5.0 on guanine-modified electrode. Guanine-modified glassy carbon electrode showed a good linear response in the concentration range from 0.5 mM to 10 μM for the quantitative determination of paracetamol with the lower limit of detection of 0.9 µM.
Introduction
One of the most commonly used drugs is paracetamol. Overdose of it can lead to the accumulation of toxic metabolites, which may lead to severe and even fatal hepatotoxicity and nephrotoxicity (Bui et al., 2012). Therefore, it is important to develop a method to determine paracetamol.
There are many reports in this area, including titration (Kumar et al., 1997), spectrophotometry (Filik et al., 2009), capillary electrophoresis (Pérez-Ruiz et al., 2005), fluorometry (Al-Ghannam et al., 2002), FIA (Wangfuengkanagul et al., 2002) and HPLC (Nebot et al., 2007). However, these methods also have some disadvantages, including the need for sample pretreatment, high cost, and long analysis time. Spectrophotometry is a simple, rapid, and inexpensive method for the determination of paracetamol, but it has some limitations (Montaseri et al., 2018). The existence of spectral interference caused by other components of the drug, the maximum wavelength of paracetamol is 244 nm, which can be interfered with propyphenazone (266 nm) and caffeine (273 nm) (Delvadiya et al., 2013). Therefore, methods for the determination of paracetamol in pharmaceuticals need more selective and sensitive methods.
For this purpose, a chemical-modified electrode can be modified with guanine. The chemical-modified electrode is coupled to an adapter that converts its interaction with the analyte into a measurable electrochemical signal (Boumya et al., 2021).
Materials and Methods
Chemicals and Reagents
All reagents used for the development of this work were of analytical grade, obtained from Merck, Fluka, Riedel, and Sigma-Aldrich, including the pharmaceuticals paracetamol and guanine. All the chemicals were used without any purification. All solutions of used chemicals in characterization were prepared as 1 mM in non-aqueous medium; 100 mM tetrabutylammonium tetrafluoroborate (NBu4BF4) in acetonitrile (CH3CN). The employed BR buffer solutions were prepared by mixing H3PO4, H3BO3, and CH3COOH according to preparation conditions in the literature, completed to the level by using ultrapure water. The pH adjustments were carried out by dropwise addition of 0.1 M/1 M NaOH by a digital pH meter. All guanine solutions used in modification were 1 mM concentration in 0.1 M H2SO4 solution. The modification was carried out only in aqueous media, while the electrochemical characterization was done in both non-aqueous and aqueous media.
Instruments
Ultrapure water with a resistance of 18.2 MΩ cm (MP minipure purification system, USA) was used to preparation all aqueous solutions, measurements, cleaning of the glassware, and polish the electrodes.
All electrochemical experiments were completed at room temperature (25 ± 1°C) under a traditional three-electrode cell system for all electrochemical experiments.
pH meter with a combined glass pH electrode (VWR pHenomenal) was used to measure the pH value in the aqueous solutions.
CV and electrochemical impedance spectroscopic (EIS) techniques were performed using a GAMRY reference PCI4/Series 750 and Reference 600+ Potentiostat/Galvanostat/ZRA from GAMRY Instruments (USA) equipped with a BAS (Bioanalytical Systems, USA) model C3 cell stand.
Ag/AgCl/KCl (sat) (BAS Model MF-2063) reference electrode was used in aqueous solutions. Ag/Ag+ (10 mM AgNO3) (BAS Model MF 2042) in 0.1 M NBu4BF4 in CH3CN reference electrode was used in non-aqueous solutions. A platinum wire (BAS Model MW-1033) was used as a counter electrode. Bare GC electrode with a geometric area of 0.071 cm2 or guanine-modified GC electrode BAS (Bioanalytical Systems, USA) model MF-2012 and the Tokai GC-20 (Japan) GC electrode were used as a working electrode in all electrochemical experiments such as CV, EIS and DPV. The GC electrode was cleaned and polished with 100 and 50 nm Al2O3 suspension (Baikowski Int. Corp., USA) on polishing pad (Buehler, USA) for about 5 min and then washed with ultrapure water (UPW). Polished GC electrodes were sonicated (Bandelin electronic, RK100H, Germany) in UPW for about 5 min in the following sonication GC electrodes were dipped in CH3CN media. All working electrodes were kept in CH3CN when they were not in use in all experiments.
Electrode preparation and modification
The GC electrodes were prepared for the experiments by polishing to gain a mirror-like appearance, first with fine wet emery papers (grain size 4000) and then with 1.0 μm and 0.3 μm alumina slurry on microcloth pads (Buehler, USA). After the initial polishing, the GC electrodes were resurfaced with 0.05 μm alumina slurry. First, in the following order, the GC electrodes were sonicated both in water and in CH3CN for 10 min, (Demir Mulazımoglu and Mulazımoglu, 2013). Electrochemical surface modification experiments were performed in the +0.5 and +1.7 V potential range at a 0.1 V s-1 scan rate with 20 cycles. Then reduction experiments were achieved by using 0.1 M HCl solution in the 0 and -1.2 V potential range at a 0.1 V s-1 scan rate with 5 cycles. After the modification of the GC electrode, the surface of the obtained rGu/GC electrode was washed to remove all impurities from the electrode surface, and it was then used for other investigations described in this study.
Results
Modification of GC electrodes
Electrode modification plays an important role in electrochemical research and has been widely used in the past decade. These modified electrodes have achieved remarkable results in the determination of organic and inorganic substances. Since the electrode surface can be prepared by electrochemical oxidation or reduction in a suitable medium and under optimal preparation conditions, modified electrodes can be physically prepared to get a sensor. Although electrode modification is widely performed in aqueous media, recent studies can also be applied to non-aqueous media. Modification methods in aqueous media are preferred since assays in research are often performed in aqueous media, which are considered to be more stable.
The electrochemical modification on the surface of the GC electrodes was achieved with 1 mM guanine in 0.1M H2SO4 as supporting electrolyte in aqueous media from +0.5 V to +1.7 V potential ranges using 0.1 V s−1 sweep rate with 20 cycles (Figure 1A).
Figure 1: Cyclic voltammograms of preparation of guanine modified GC electrode, 1 mM aqueous solution of guanine was taken in 0.1 M H2SO4 at 20 cycles with scan rate 0.1 V s−1 (A). Cyclic voltammograms of reduction of guanine modified GC electrode, 0.1 M HCl was taken at 5 cycles with scan rate 0.1 V s−1 (B)
The modified surface was electro-inactive. So, the surface was activated by reducing it in the 0 and -1.2 V potential range using 0.1 V s-1 scanning rate with 5 cycles (Figure 1B).
After that, the surface of obtained rGu/GC electrode was washed to remove all impurities from the electrode surface and then it was used for other investigations described in this study.
Electrochemical characterization of rGu/GC electrode
As the surface characterization processes after modifications and reduction were performed electrochemically by using ferrocene redox probe in non-aqueous medium and Fe(CN)63- in aqueous medium with CV. For the characterization processes, it was made with ferrocene prepared by dissolving in 0.1 M NBu4BF4. A voltammogram with a potential range from +0.2 V to +0.6 V and a sweeping rate of 0.1 V s-1 was achieved (Figure 2A). In addition to that, characterization also has been done with Fe(CN)63- that was prepared by letting it dissolve in BR buffer solution, pH 2.0. A voltammogram with the potential range from +0.4 V to 0 V at a 0.1 V s-1 sweep rate was carried out (Figure 2B).
Figure 2: Overlaying the bare GC and rGu/GC electrode voltammograms at ferrocene and K3Fe(CN)6. A) 1 mM ferrocene redox probe solution vs. Ag/Ag+ (10 mM) in CH3CN+ 0.1 M NBu4BF4 using 0.1 V s-1 scanning rate and B) 1 mM K3Fe(CN)6 redox probe solution vs. Ag/AgCl/KCl(sat) reference electrode in BR buffer solution, pH 2.0 using 0.1 V s-1 scanning rate
Effect of buffer solution pH on the sensor response
The pH value of the electrolyte can affect the peak shape, peak potential, and peak current of the modified electrode. It is also more conducive to estimating the ratio of protons and electrons participating in the electrode reaction. As shown in Figures 3A, 3B, it was investigated the effect of pH values using BR buffer solutions ​​from 2.0 to 12.0 at a scan rate of 0.1 V s−1.
Figure 3: A) Effect of pH values use of BR buffer solutions ​​from 2.0 to 12.0 to determine paracetamol at rGu/GC electrode by DPV from -0.2 to +0.8 V using 0.1 V s-1 scanning rate, and B) Relation between pH and peak currents for 1 mM PAR at rGu/GC electrode
The observed peak potential shifted in the negative direction with increasing pH, confirming the direct involvement of protons in the rate-limiting step. Comparing the peak currents of paracetamol, the maximum response was observed at pH 5.0. Therefore, the same pH was chosen for further determination.
Effect of scan rate on CVs of paracetamol at rGu/GC electrode
The effect of scan rate on the electrochemical behavior of paracetamol was investigated by CV in BR of pH 5.0. Figure 4A shows the CVs of 1.0 mM paracetamol for BR pH 5.0 at different scan rates from 25 to 500 mV s-1.
Figure 4: A) CVs obtained at rGu/GC electrode in BR pH 5.0 containing 1.0 mM paracetamol at various scan rates (25-500 mV s-1), B) shows the plot of the anodic peak current versus scan rate. C) Relation between log scan rate and log peak current. D) Calibration plot of the redox peak currents vs. square root of scan rate. E) Plot of variation of Ep,mV versus log Ï…, mV s-1
For oxidation and reduction processes, the peak potentials shift towards more positive and negative values, respectively, indicating that both peak potentials (Epa and Epc) are a function of the scan rate. The oxidation and reduction peak currents (Ipa, Ipc) increase linearly with the square root of the scan rate (Ï…1/2) over the examined range.
Ipa(µA) = - 81.094 + 27.507 υ1/2(mV s-1)1/2
R2 = 0.9937
Ipc(µA) = 69.65 - 19.061 υ1/2(mV s-1)1/2
R2 = 0.9935
These phenomena suggest that the electrochemical reaction is a diffusion-controlled process. On the other hand, a linear regression line plot of the logarithmic peak current and the logarithmic scan rate also provides information about the process controlled by diffusion or adsorption.
Two linear relationships were obtained (Figure 4C) and represented by the following equation:
logIpa = 0.7349 logÏ… + 0.7739; R2 = 0.993
logIpa = 0.8205 logÏ… + 0.3778; R2 = 0.990
The plot of peak potential (Epa and Epc) versus log Ï… is shown in Figure 4E, and the corresponding regression equation is:
Epa = 93.63 log Ï… + 226.6; R2 = 0.9417
Epc = - 35.331 log Ï… + 364.41; R2 = 0.8401
Effect of scan rate on LSV of paracetamol at rGu/GC electrode
From Figure 5A, for oxidation processes, the peak potentials shift towards more positive values, indicating that peak potentials (Epa) are a function of the scan rate. As shown in Figure 5D, the oxidation peak currents (Ipa) increase linearly with the square root of the scan rate (Ï…1/2) over the examined range.
R2 = 0.9931
Figure 5: A) Impact of scan rate on 1.0 mM paracetamol in BR pH 5.00 at rGu/GC electrode from 20-500 mV s-1, B) shows the plot of the anodic peak current versus scan rate. C) Relation between log scan rate and log peak current. D) Calibration plot of the redox peak currents vs. square root of scan rate. E) Plot of variation of Ep, mV versus log Ï…, mV s-1
The linear relationship was obtained (Figure 5C) and represented by the following equation:
logIpa = 0.8272 logv + 0.4416; R2 = 0.9991
The plot of peak potential (Epa) versus log Ï… is shown in Figure 6E, and the corresponding regression equation is:
Epa = 81.159logv + 231.26; R2 = 0.8925
Voltammetric determination of paracetamol
Compared with conventional CVs, DPV has advantages of higher sensitivity and high resolution in quantitative analysis. Therefore, DPV was used to determined paracetamol in 0.1 M BR from 0 to +0.6 V using 0.1 V s-1 scanning rate as shown in Figure 6.
Figure 6: A) DPV curves of paracetamol on its concentration in the range of a) 5.0 × 10−4 M, b) 2.5 × 10−4 M, c) 1.0 × 10−4 M, d) 7.5 × 10−5 M, e) 5.0 × 10−5 M, f) 2.5 × 10−5 M, g) 1.0 × 10−5 M in BR buffer solution pH 5.0, and B) The plot of Ipa versus concentration of paracetamol
The oxidation experiments were performed under optimal conditions. The results of DPV are shown in Figure 6A. The oxidation peak current of paracetamol increases linearly with increasing of concentration. Figure 6B shows the linear relationship from 0.5 mM to 10 μM using the following linear equation:
Ipa(μA) = 0.0931 μM x [paracetamol] + 0.3688
R2 = 0.9904
The limit of detection (LOD) was 0.9 µM, and the limit of quantification (LOQ) was 2.7 µM.
Discussion
Guanine and adenine are important components of DNA (Wang et al., 2002). The electrode surface can be prepared by electrochemical oxidation or reduction in a suitable medium under optimal preparation conditions. Although electrode modification is widely used in aqueous media. Our previous studies (Mulazımoglu and Demir Mulazımoglu, 2012; Demir Mulazımoglu and Mulazımoglu, 2013), demonstrated electrode modification with amine-containing compounds. The main purpose objective of this study was to improve a new chemical sensor electrode for the determination of paracetamol by using electrochemical and spectroelectrochemical techniques. (Demir Mulazımoglu and Mulazımoglu, 2013; Dundar et al., 2011; Celik et al., 2020). The main target of this study was achieved to electrochemically modify guanine onto the GC electrode surface by using cyclic voltammetry (CV) and characterize Gu-modified GC (rGu/GC) electrode by CV. This study also was performed to investigate whether the new sensor obtained is sensitive to the paracetamol through differential pulse voltammetry (DPV).
Recently, electrochemical methods have attracted much attention due to their simplicity, low cost, high sensitivity, and the possibility of miniaturization (Beitollahi et al., 2013; Esfandiari Baghbamidi et al., 2013; Mohammadizadeh et al., 2018; Mohammadi et al., 2019). Electrochemical techniques are of great significance in environmental monitoring, medicine and biotechnology, and industrial process management (Mohammadi et al., 2021). Paracetamol is an electroactive compound that can be detected using voltammetry. Voltammetry has high sensitivity, high precision, low cost, low detection limit, and wider linear range (Ejaz et al., 2017). The voltametric determination of paracetamol is widely used in blood samples, water, drugs and mixed compounds. Results showed recoveries of 97-102%, with highly reproducible responses (Ozcan et al., 2007; Akbari et al., 2018; Berto, 2018; Tanuja et al., 2018).
Among them, carbon-based electrodes are widely used in voltammetry because of their low cost, wide potential window, low resistivity, and versatility of chemical modification. Chemically modified electrodes are very interesting tools for the analysis of various trace substances using sensitive electroanalytical techniques. In this case, it is very important to choose the most suitable modifier for each analyte because the sensitivity and selectivity of the electroanalytical reaction depend on the nature of the modifier (Barreto et al., 2023; Liu et al., 2023; Siavashi et al., 2023; Smajdor et al., 2023). Modification of carbon electrodes with organic molecules can improve the sensitivity and selectivity of paracetamol determination. The presence of specific modifier molecules on the surface of the electrode allows only certain molecules to diffuse to its surface.
In this work, by using paracetamol as test analytes, the effectiveness of using modified carbon-based electrodes as an inexpensive and simple in pharmaceutical analysis is evaluated (Akca et al., 2022; Gashu et al., 2022; Kablan et al., 2022; Kassem et al., 2022; Ozkan et al., 2022; Budak et al., 2023; Mohamed et al., 2023).
The test analytes were determined individually by differential pulse voltammetry using guanine-modified glassy carbon electrode. Under optimized experimental conditions (BR buffer solution pH 5.0), the electrode showed a linear response in the range of 0.5 mM to 10.0 μM for quantitative determination of paracetamol with lower limit of detection of 0.9 µM. In this research, advantages were taken of its efficiency, availability, and low cost, avoiding complex, time-consuming. This study provides a reliable and economical basis for the development of low-cost, biodegradable electrode materials for electrochemical sensors. The technology can be used more than once sensor device, similar to a glucose sensor (Sun et al., 2022; Yang et al., 2022; Youcef et al., 2022; Zainul et al., 2022), to measure and quantify paracetamol.
Produced electrodes were tested for acceptable reproducibility. Ultimately, the practical application of the developed sensor can be successfully used for the quantitative measurement of paracetamol in drug and serum samples (Hussain et al., 2023; Nigussie et al., 2023; Qomi et al., 2023; Richard et al., 2023; Uzun et al., 2023). Therefore, this strategy is expected to provide a simple and novel approach for sensor fabrication for the determination of paracetamol.
Conclusion
The rGu/GC electrode presented an acceptable analytical performance for paracetamol determination with a wide linear range, from 0.5 mM to 10 μM with the limit of detection of 0.9 µM.
References
Akbari M, Shayani-Jam H, Yaftian M, Parinejad M. Electrochemical oxidation of acetaminophen in the presence of diclofenac and piroxicam: Synthesis of new derivatives and kinetic investigation of toxic quinone imine/drugs interactions. J Electroanal Chem. 2018; 827: 160-66.
Akca Z, Izem Ozok H, Yardim Y, Senturk Z. Electroanalytical investigation and voltammetric quantification of antiviral drug favipiravir in the pharmaceutical formulation and urine sample using a glassy carbon electrode in anionic surfactant media. Turk J Chem. 2022; 46: 869-80.
Al-Ghannam M, El-Brashy M, Al-Farhan S. Fluorimetric determination of some thiol compounds in their dosage forms. Farmaco 2002; 57: 625-29.
Barreto F, Leme da Silva M, Cesarino I. Copper nanoparticles and reduced graphene oxide as an electrode modifier for the development of an electrochemical sensing platform for chloroquine phosphate determination. Nanomaterials 2023; 13: 1436-47.
Beitollahi H, Mohammadi S. Selective voltammetric determination of norepinephrine in the presence of acetaminophen and tryptophan on the surface of a modified carbon nanotube paste electrode. Mater Sci Eng C. 2013; 33: 3214-19.
Berto S. Application of an electro-activated glassy-carbon electrode to the determination of acetaminophen (paracetamol) in surface waters. Electrochim Acta. 2018; 284: 279-86.
Boumya W, Taoufik N, Achak M, Barka N. Chemically modified carbon-based electrodes for the determination of paracetamol in drugs and biological samples. J Pharm Anal. 2021; 11: 138-54.
Budak F, Cetinkaya A, Kaya SI, Atici EB, Ozkan SA. Sensitive determination and electrochemical evaluation of anticancer drug tofacitinib in pharmaceutical and biological samples using glassy carbon and boron-doped diamond electrodes. Diam Relat Mater. 2023; 133: 109751.
Bui N, Li A, Han N, Pham X, Seong H. Determination of acetaminophen by electrochemical co-deposition of glutamic acid and gold nanoparticles. Sens Actuators B Chem. 2012; 174: 318-24.
Celik H, Ozcan S, Demir Mülazımoğlu A, Yılmaz E, Mercimek B, Çukurovalı A, Yılmaz I, Solak AO, Mulazımoglu IE. The synthesis of a novel DDPHC diazonium salt: Investigation of its usability in the determination of phenol and chlorophenols using CV, SWV and DPV techniques. Inorg Chem Commun. 2020; 116: 107893.
Delvadiya K, Kimbahune R, Kabra P, Sunil K, Patel P, Nargund L. High-performance liquid chromatographic determination of paracetamol, propyphenazone, and caffeine in pharmaceutical formulations. Indian J Pharm Educ Res. 2013; 47: 65-72.
Demir Mulazımoglu A, Mulazımoglu IE. Electrochemical properties of MDA/GC electrode and investigation of usability as sensor electrode for determination of Que, Kae, Lut and Gal using CV, DPV and SWV. Food Anal Methods. 2013; 6: 141-47.
Demir Mulazımoglu A, Mulazımoglu IE. Electrochemical behaviors of 2-amino-3-hydroxypyridine onto the glassy carbon sensor electrode: Simultaneous and independent determinations of quercetin, galangin, 3-hydroxyflavone, and chrysin. Food Anal Methods. 2013; 6: 845-53.
Dundar E, Mulazımoglu IE, Ozkan E. Electrochemical and spectroelectrochemical investigation of the behaviors of HF, 3-HF, 6-HF, 3,6-DHF on glassy carbon electrode surfaces. Rev Anal Chem. 2011; 30: 18-20.
Ejaz A, Jeon S. A highly stable and sensitive GO-XDA-Mn2O3 electrochemical sensor for simultaneous electrooxidation of paracetamol and ascorbic acid. Electrochim Acta. 2017; 245: 742-51.
Esfandiari Baghbamidi S, Beitollahi H, Mohammadi S, Tajik S, Soltani-Nejad S, Soltani-Nejad V. Nanostructure-based electrochemical sensor for the voltammetric determination of benserazide, uric acid, and folic acid. Chin J Catal. 2013; 34: 1869-75.
Filik H, Aksu D, Apak R, Sener S, Kılıc E. An optical fibre reflectance sensor for paminophenol determination based on tetrahydroxycalix[4]arene as sensing reagent. Sens Actuators B Chem. 2009; 136: 105-12.
Gashu M, Kassa A, Tefera M, Amare M, Aragaw B. Sensitive and selective electrochemical determination of doxycycline in pharmaceutical formulations using poly(dipicrylamine) modified glassy carbon electrode. Sens Bio-Sens Res. 2022; 37: 100507.
Hussain S, Sadiq I, Baig J, Sadiq F, Shahbaz M, Solangi I, Idrees M, Saeed S, Riaz S, Naseem S. Synthesis and characterization of vanadium ferrites, electrochemical sensing of acetaminophen in biological fluids and pharmaceutical samples. Ceramics Int. 2023; 49: 8165–71.
Kablan S, Recber T, Tezel G, Timur S, Karabulut C, Karabulut T, Eroglu H, Kır S, Nemutlu E. Voltammetric sensor for COVID-19 drug Molnupiravir on modified glassy carbon electrode with electrochemically reduced graphene oxide. J Electroanal Chem. 2022; 920: 116579.
Kassem M, Awad M, Morad M, Aljahdali B, Pashameah R, Alessa H, Mohammed G, Sayqal A. Sensor based on copper nanoparticles modified electrochemically activated glassy carbon electrode for paracetamol determination. Int J Electrochem Sci. 2022; 17: 220441.
Kumar G, Letha R. Determination of paracetamol in pure form and in dosage forms using N,N-di-bromo dimethylhydantoin. J Pharm Biomed Anal. 1997; 15: 1725-28.
Liu Y, Zhang Z, Li Y, Shi F, Ai Y, Wang B, Zhang S, Zhang X, Sun W. Electrochemical detection of hydroquinone based on marine biomass carbon from shrimp shells as electrode modifier. Int J Electrochem Sci. 2023; 18: 100063.
Mohamed E, Mahmoud A, Nashat N, El‑Mosallamy S. Fabrication of novel electropolymerized conductive polymer of hydrophobic perfuorinated aniline as transducer layer on glassy carbon electrode: Application to midazolam as a model drug of benzodiazepines. BMC Chem. 2023; 2023.
Mohammadi S, Beitollahi H, Askaria M, Hosseinzadeh R. Application of a modified carbon paste electrode using core–shell magnetic nanoparticle and modifier for simultaneous determination of norepinephrine, acetaminophen and tryptophan. Russian J Electrochem. 2021; 57: 74–84.
Mohammadi S, Beitollahi H, Khodaparast B, Hosseinzadeh R. Electrochemical determination of epinephrine, uric acid and folic acid using a carbon paste electrode modified with novel ferrocene derivative and core–shell magnetic nanoparticles. Res Chem Intermed. 2019; 45: 1117-29.
Mohammadizadeh N, Mohammadi S, Kaykhaii M. Carbon paste electrode modified with ZrO2 nanoparticles and ionic liquid for sensing of dopamine in the presence of uric acid. J Anal Chem. 2018; 73: 685-94.
Montaseri H, Forbes P. Analytical techniques for the determination of acetaminophen: A review. Trends Anal Chem. 2018; 108: 122-34.
Mulazımoglu IE, Demir Mulazımoglu A. Investigation of sensitivity against different flavonoid derivatives of aminophenyl-modified glassy carbon sensor electrode and antioxidant activities. Food Anal Methods. 2012; 5: 1419-26.
Mulazımoglu IE. Electrochemical determination of copper (II) ions at naringenin-modified glassy carbon electrode: application in lake water sample. Desalin Water Treat. 2012; 44: 161–67.
Nebot C, Gibb W, Boyd G. Quantification of human pharmaceuticals in water samples by high performance liquid chromatography: Tandem mass spectrometry. Anal Chim Acta. 2007; 598: 87-94.
Nigussie M, Kassa A, Guadie A, Mulu M, Lijalem T, TeferaM. Selective and sensitive determination of tinidazole in pharmaceuticals and biological matrix using poly(diphenylamine-4-sulfonic acid) modified glassy carbon electrode. Sens Bio-Sens Res. 2023; 39: 100552.
Ozcan L, Sahin Y. Determination of paracetamol based on electropolymerized-molecularly imprinted polypyrrole modified pencil graphite electrode. Sens Actuators B Chem. 2007; 127: 362–69.
Ozkan E, Cetinkaya A, Ozcelikay G, Nemutlu E, Kır S, Ozkan S. Sensitive and cost-effective boron doped diamond and Fe2O3/Chitosan nanocomposite modified glassy carbon electrodes for the trace level quantification of anti-diabetic dapagliflozin drug. J Electroanal Chem. 2022; 908: 116092.
Pérez-Ruiz T, MartÃnez-Lozano C, Tomás V, Galera R. Migration behavior and separation of acetaminophen and p-aminophenol in capillary zone electrophoresis: Analysis of drugs based on acetaminophen. J Pharm Biomed Anal. 2005; 38: 87-93.
Qomi M, Sarvestani M. Highly sensitive voltammetric determination of acetaminophen by an overoxidized poly (p-aminophenol) modified glassy carbon electrode. Int J New Chem. 2023; 10: 140-50.
Richard Y, Lincy S, Saravanakumar R, Maheswaran R, Dharu-man V. Sensitive detection of acetaminophen in body fluids, pharmaceuticals and herbal medicines at undoped mesoporous carbon nitride film electrode. Microchem J. 2023; 184: 108175.
Siavashi R, Beitollahi H. Molecularly imprinted polymer based sensor for measuring of levodopa: Evaluation as a modifier for glassy carbon electrode in electrochemically detection. Russian J Electrochem. 2023; 59: 70–78.
Smajdor J, Paczosa-Bator B, Grabarczyk M, Piech R. Glassy carbon electrode modified with CB/TiO2 layer for sensitive determination of sumatriptan by means of voltammetry and flow injection analysis. Sensors 2023; 23: 5397-412.
Sun Y, Xue W, Zhao J, Bao Q, Zhang K, Liu Y, Li H. Direct electrochemistry of glucose dehydrogenase-functionalized polymers on a modified glassy carbon electrode and its molecular recognition of glucose. Int J Mol Sci. 2023; 24: 6152.
Tanuja S, Kumara S, Vasantakumar P. Voltammetric determination of paracetamol using polyvinyl alcohol (PVA)-Fe3O4 modified glassy carbon electrode. Ind Chem. 2018; 4: 1-10.
Uzun D, Calam T. Electrochemical behavior and ultrasensitive, simple and effective voltammetric determination of acetaminophen using modified glassy carbon electrode based on 4- hydroxyquinoline-3-carboxylic acid. Electroanalysis 2023; 35: 2200182.
Wang H, Ju H, Chen H. Simultaneous determination of guanine and adenine in DNA using an electrochemically pretreated glassy carbon electrode. Anal Chim Acta. 2002; 461: 243-50.
Wangfuengkanagul N, Chailapakul O. Electrochemical analysis of acetaminophen using a boron-doped diamond thin film electrode applied to flow injection system. J Pharm Biomed Anal. 2002; 28: 841-47.
Yang T, Xie Y, Zhang X, Guo R, Yang X. One-step electro-deposition of CuNi bimetallic nanocatalyst anchored on reduced graphene oxide modified glassy carbon electrode for nonenzymatic sensing of glucose. Int J Electrochem Sci. 2022; 17: 22098.
Youcef M, Hamza B, Nora H, Walid B, Salima M, Ahmed B, Malika F, Marc S, Christian B, Wassila D, Djamel Eddine M, Larbi Z. A novel green synthesized NiO nanoparticles modified glassy carbon electrode for non-enzymatic glucose sensing. Microchem J. 2022; 178: 107332.
Zainul R, Isa I, Yazid S, Hashim N, Sharif S, Saidin M, Ahmad M, Suyanta M, Amir Y. Enhanced electrochemical sensor for electrocatalytic glucose analysis in orange juices and milk by the integration of the electron-withdrawing substituents on graphene/ glassy carbon electrode. J Anal Methods Chem. 2022; 5029036.
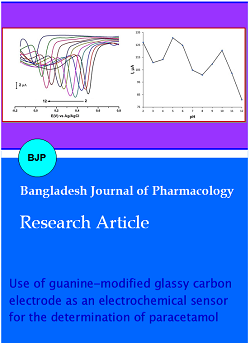